Using Transparent Iron Oxide Pigments in Wood Finish Applications
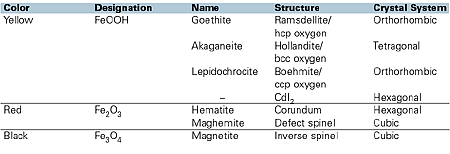
Since the end of the 19th century, iron oxide pigments have been manufactured on a commercial scale, overcoming the variability in the colors obtained from natural sources. These pigments have again been predominantly large particle sized pigments maximizing opacity and providing consistent, clean products with high color strength. The main applications for this pigment type have been the construction industry.
In the past 40 years, a market has developed for a pigment where color, transparency and ultraviolet (UV) light protection are required. Synthetically produced transparent iron oxides, offering high purity, consistency, cleanliness and clarity meet this requirement.
The synthetic production of transparent iron oxide pigments provides for a range of different hues and morphologies, whereby acidic (pH 3.0) to slightly alkaline products (pH 8.5) are available. Acidic pigments tend to exhibit cleaner shades but are difficult to fully disperse. Acidic grades are not recommended for waterborne systems because the chemical balance used in the formation of the waterborne resin systems can be disrupted and the resin precipitated. Neutral and slightly alkali pigments offer greater dispersion stability and better performance in these systems.
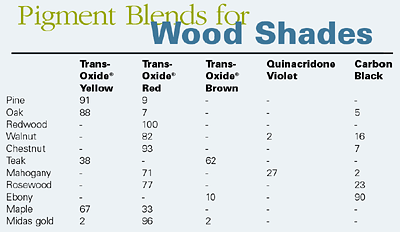
Transparent iron oxides are multi-functional nontoxic pigments that combine a range of color shades with excellent UV absorption, transparency and weathering stability. It is possible by the blending of transparent iron oxide pigments with other pigments to achieve a range of standard wood shades.
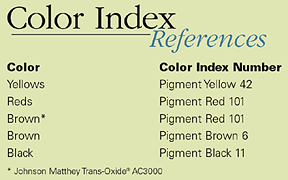
Environmental Aspects
Having undergone numerous toxicological testing, iron oxide pigments have shown no harmful effects and are considered to be both environmentally and ecologically safe. Tests have shown the LD50 to be greater than 10,000 mg/kg and, although it is not an irritant, at high concentration levels mechanical irritation effects can occur. American dust restrictions for iron oxide pigments are OSHA (Z-3) 15 mg/m3 TWA and ACGIH (1989) 10 mg/m3 TWA. In Germany, there is a general dust threshold value of 6 mg/m3, which applies to all materials. A further restriction for iron oxide dusts, which contain more than 1% crystalline silica, does not apply to synthetic transparent iron oxides as these contain less than the 1% total silica. Therefore, the only requirement for transparent iron oxide pigments is to ensure that the occupational hygiene measures are sufficient to prevent these dust limits being exceeded. In Europe, transparent iron oxide pigments are not classified as “Special Waste” or hazardous under the Chemicals Hazard Information and Packaging for Supply regulations 1994. Transparent iron oxide pigments meet the FDA’s purity requirements for various applications. For example, they are suitable as colorants for toys, wooden building blocks and rocking horses. They also meet the requirements of the Council of Europe AP (89)1 for polymers in contact with food, for example, wooden kitchen utensils and bowls.

Particle Form
Careful control of the preparation process of transparent iron oxide pigments results in the formation of pigments with very small primary particle sizes. When fully dispersed, they do not scatter light and are hence completely transparent. The very small primary particle size results in powders with high surface areas, typically between 80m2g-1 and 120m2g–1 and therefore, for inorganic pigments, relatively high oil absorption figures, typically 40–45 % v/w.A further consequence of the very small particle size of transparent iron oxide is that very high interfacial forces exist between the primary particles. These forces cluster the primary particles into aggregates, which are difficult to wet out and disperse. The aggregates, produced during the manufacturing process, also tend to adhere together and form agglomerates. These agglomerates are, however, very easy to wet out, break down and disperse. It is the aggregates, which are present in all transparent iron oxides, that necessitate high shear dispersion processes such as bead milling or attrition milling to fully exploit the transparency of these pigment types (see Figure 1). High-speed mixing will not provide sufficient shear to disperse the aggregates and thereby develop the high level of transparency and dispersion stability normally associated with transparent iron oxide dispersions. A common solution for the paint maker is to purchase the pigment in a pre-dispersed form. Once the pigment is fully dispersed, the size is such that the resultant colloidal suspension is stable and good in-can stability is achieved. This is not the case with larger dense particles which need additional stabilization through rheology and/or charge modifiers to be included in the formulation.
Micropulverization does not produce easier-to-disperse transparent iron oxide pigments, as the aggregates tend to become compacted and are thus more difficult to wet out and disperse. Johnson Matthey generally recommends the micropulverized pigments for applications where screen clogging is an issue, or when low-viscosity systems are being used with poor agitation allowing settlement of the larger particles before they are de-agglomerated.
Light Interactions
Light is a form of energy. As such, in accordance with the law of Conservation of Energy, energy can not be destroyed or gained with any given closed system; it can only be converted to other forms of energy.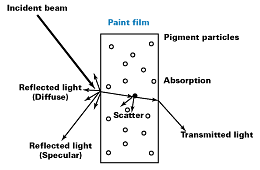
Reflection
When a light beam strikes a pigmented film, a portion of the light is “reflected” at the surface of the film. This can be specular reflection, where the angle of incidence is the same as the angle of reflectance. This is commonly recognized as gloss (see Figure 2). Due to surface imperfections, the reflected light can be reflected in many different directions. This is known as diffuse reflection; if it occurs to a large extent, then the film is matte.Refraction
The rest of the light is “refracted” as it enters the pigmented film; light is always refracted at any interface where the refractive index of the two components is different. The greater the difference in refractive index, the greater the shift in the angle of refraction.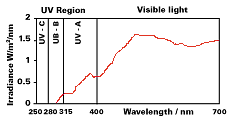
Absorption
Once the light has entered the pigmented film, it interacts with the pigment. When the light strikes a pigment particle, some of the energy is “absorbed” by the pigment. The color of the transparent iron oxide pigments is a result of crystal field splitting of the five d orbitals of the Fe3+ ion, thus allowing for the absorption of certain wavelengths of light resulting in the familiar shades of iron oxides. The crystallinity of the crystal structure determines the specificity of the wavelengths of light absorbed, which defines the purity of the color shade.Subtle nuances of shade can also be obtained by changing the manufacturing processes to influence particle shape. For example, changes in the needle aspect ratio can change yellows from a green shade to a redder shade of yellow.
Because light can only penetrate a few atomic layers at the surface of the iron oxide particles, the absorption mechanism can only be achieved from a small proportion of the iron oxide material. By reducing the particle size, the effective surface area available to create the color is increased, thus giving high color strength pigments and improved UV light protection.
The ultraviolet component of sunlight (280–400 nanometers) is principally responsible for this degradation through the decomposition of the lignin in wood and resultant destruction of the cell structure (see Figure 3). Transparent iron oxide is a strong absorber of UV radiation, providing protection for the wood substrate, while its color and transparency enhance the appearance of the natural wood grain.

Scatter
Light, which strikes the iron oxide particle and is reflected, not absorbed, within the paint film, is said to have been scattered. Based on the Mie theory, the scattering of light by a particle can be shown to be a function of the ratio of the particle dimension, the wavelength of light, [symbol missing], and the ratio of the refractive indices of the pigment and the medium (see Figure 4).
Transmission
The particle size of transparent iron oxide pigments is manufactured to be acicular in nature with dimensions of less than 20 nanometers wide by less than 150 nanometers long to ensure that there is no interference with some wavelengths of light, and hence the products are transparent.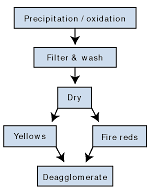
Manufacturing Process
Iron oxides can be produced by way of several different manufacturing routes, but the preferred processes for the manufacture of transparent iron oxide involve precipitation and oxidation of iron from a ferrous salt solution (see Figure 5). Ferrous sulphate is the preferred salt, as it is readily available, a byproduct, from the manufacture of titanium dioxide pigment or from the steel pickling industry.The iron oxide particles are produced by the combination of the ferrous salt with an alkali, which produces a “green rust” gel. This gel is readily oxidized to produce the particles of iron oxide. The crucial parameters to produce the correct color, dispersibility and transparency are pH, concentration, temperature, and rates of reaction.
The purity of the crystals produced, both in terms of foreign metal impurities and crystal phase, is an important feature in determining the hue and chroma of the pigment. The different phases of iron oxide are different hues. For example, goethite is a green shade yellow, whereas lepidochrocite is a red shade yellow.
Black iron oxides can be manufactured either by a direct precipitation and oxidation route, or by the partial reduction of red iron oxide during a calcination process. Brown iron oxides are usually achieved by the blending of red, yellow and black shades, resulting in the customer-specific requirement. In 1995, Johnson Matthey patented a process of iron substitution within the hematite structure that results in a single pigment brown, offering greater thermal stability, light and weatherfastness than is achieved by using blends of pigments.
After the crucial stage of formation of the goethite crystals, the iron oxide is then filtered and washed to remove salts generated during the precipitation process. Failure to remove these tend to cause greater aggregation (reduced dispersibility) and the salts can impact on the performance of the resin medium.
The drying process, especially for non-calcined products, is another crucial process — over-drying causes aggregation reducing the products ability to disperse.
Although transparent red iron oxide can be produced by direct precipitation, again the conventional route taken is to dehydrate the goethite crystals by firing. The dehydration process commences at low temperatures, typically 180ºC, which can be influenced by ambient conditions and duration at which the product is held at temperature.
2FeOOH —————> Fe2O3 + H2O (yellow) 180ºC (red)(steam)
Although the principal characteristics of the pigments size and shape are determined during the precipitation and oxidation process, the calcination also plays a key role. During the calcination process, the acicular nature of the goethite crystals may be lost in favor of more elliptical to spherical particles. In addition, particle aggregation and inter-particle sintering can take place effectively increasing the particle size.
Any milling or size reduction process that is incorporated tends to be detrimental to the dispersibility and development of transparency — it tends to compact agglomerates, making them more difficult to wet the surfaces of the pigments primary particles.
Benefits of Transparent Iron Oxides
The high durability and weatherfastness of transparent iron oxides make them the natural choice for wood stains and furniture finishes. They are resistant to acids, alkalis and solvents, and are non-bleeding/non-migratory. They exhibit excellent weatherfastness and gloss retention properties as confirmed by industry standard external exposure testing.Transparent iron oxide differs from the opaque iron oxide principally in terms of particle size and shape. These differences, however, impart significantly different pigmentary properties, similar to water in the form of rain or fog! The opaque pigments offer higher tinting strength. The transparent iron oxides offer higher UV protection and, important for the wood finish formulator, offer far greater aesthetic value to the finished article.
The heat stability of yellow iron oxides is lower than that of red iron oxides. The chemical composition of yellow iron is a hydrated form of the red iron oxide and this dehydration process occurs at a relatively low temperature. Since the surface area of transparent iron oxide is significantly higher than opaque iron oxide, the thermal stability is reduced slightly further. The stability of yellow transparent iron oxide is both temperature and time dependent. The stability is generally recognized as being approximately 180ºC, and is therefore suitable for wood applications.
As discussed previously, transparent iron oxides offer extremely good UV protection of the supporting medium and the substrate. Although pigments such as opaque iron oxide, carbon black and zinc oxide can also be used as UV absorbing materials, the recommended usage level renders these products to range between opaque and translucent, thus impairing the aesthetics of the wood grain finish. For UV-cure wood applications, it may be necessary to slightly increase the loading of the photoinitiator.
Transparent iron oxide pigments, because they are inorganic, offer excellent permanence. They do not migrate or bleed from the finished article and are of a size small enough to penetrate into the pores of the wood structure. Greater cost effectiveness and permanence can be achieved by using transparent iron oxide pigments rather than organic dyes in conjunction with expensive organic UV absorbers, which tend to be sacrificial by their mechanism of absorption.

Dispersion Process
Transparent iron oxide pigments, as described earlier, are notoriously difficult to disperse. A number of companies specialize in the manufacture of dispersions at a high pigment loading, which can easily be stirred into a customer’s formulation. The dispersion process is carefully controlled to ensure adequate wetting of particles, disruption of aggregates and subsequent stabilization of primary particles in the dispersion.Dispersions for wood applications are produced using transparent iron oxide pigments at a final pigment content of 30–50% in a range of media systems for solventborne (e.g., long oil alkyd/white spirit) or waterborne applications. The waterborne systems may contain suitable resin, providing excellent performance in complementary paint formulations, or they may be surfactant-based, which offers far wider application but with a slightly inferior performance compared with the resin-based counterpart.
Transparent iron oxide pigments are available in both neutral and acidic grades. Neutral grades tend to be used in both solvent- and waterborne systems, whereas acidic pigments tend to be more system specific and are only used in solventborne formulations. The correct formulation of the system is key to the successful dispersion of transparent iron oxide pigments. If the formulation fails to provide sufficient binder for the demand of the high surface area of the pigment, then an unstable dispersion or incomplete optimal transparency may result. The ideal formulation is also dependent on the dispersion process.
For relatively low-viscosity systems, a bead mill containing glass, steel or zirconia media is preferred, although ball mills or attritors may also be used. Where high viscosity formulations are required, for example pastes or concentrates at high pigment loading, then two or even three roll mills may be required.
The first stage of a dispersion process is to wet the pigment surface, then de-agglomerate and de-aggregate the primary particles, and finally to develop the stability of the dispersion. Trans-Oxide® pigments are manufactured with controlled surface absorbed moisture to enhance the first stage of dispersion.
The premix is preferably carried out at a high viscosity (5–50 poise), and is a key phase in the surface wetting and initial disruption of agglomerates. If the pigment loading is too low, then low viscosity results and extended grind times become essential to achieve optimum transparency. Modification of the amount of solvent added prior to the dispersion stage can control viscosity of the system being dispersed. Additional solvent can then be added as necessary as a final adjustment. High-viscosity premixing is most suitable for solventborne systems; waterbornes tend to have a lower system viscosity.
Normally the viscosity of the premix is reduced to between five and 10 poise prior to beadmilling by the addition of solvent. The beadmill is preferably horizontal with zirconia media, although glass and steel are also suitable. Dispersion times are usually significant (25+ hours), with continued transparency improvements achieved after acceptable levels of dispersion and stability are reached. The dispersion process can often be enhanced if the temperature of the dispersion is raised above ambient, e.g. 40–50ºC, except where safety requires the lower temperature. Solvent loss should be considered and minimized (see Table 2).
Conclusion
Synthetic transparent iron oxides are readily available in powder form and as pre-dispersed dispersions for solvent- or waterborne applications. Available in a range of natural color shades from yellow through red to brown, transparent iron oxides offer the wood-finish formulator the combination of the following.- Excellent transparency
- Permanence to light
- Durability
- Chemical resistance
- UV screening
- Economy
- Non-toxicity
These pigment types are currently being used on a global basis in thin film preservatives, furniture wiping stains and exterior stains adding protection, glamour and warmth to the natural beauty of wood. They find application on a wide variety of wood care systems, including those for fencing, decks, lawn furniture, siding, cabinetry and flooring.
For more information on transparent iron oxide pigments or dispersions, contact Johnson Matthey Pigments and Dispersions, 4820 Industry Drive, Fairfield, OH 45014; phone 800/770.2634 or 513/858.6670; fax 513/858.6703;
e-mail mckenmf@jmusa.com; visit www.matthey.com.
Links
Looking for a reprint of this article?
From high-res PDFs to custom plaques, order your copy today!