Like conventional binders, HLPs are intended to serve as the sole film-forming component in the paint formulation, and can be used to make paints with film properties similar to those made from conventional binders. In addition, like conventional light-scattering pigments, they provide opacity to the paint. Because the binder itself in a paint made with a HLP provides some opacity to a paint film, coatings can be made at a given level of hiding using lower amounts of conventional pigments such as TiO2. This, in turn, can result in lower raw material costs for the paint formulation.
The structure of a HLP closely resembles that of a conventional opaque polymer pigment particle encapsulated in film-forming binder. Because it is necessary to consider both the pigment and binder aspects of HLPs, it is often convenient to think of them as a combination of opaque polymer and binder, and that language will be used frequently in the discussion that follows. However, as will be seen, a HLP behaves quite differently than a simple physical blend of a binder with an opaque polymer and allows for the formulation of rather unique paints.
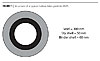
Hollow Latex Particle Structure
The morphology of a typical HLP is shown schematically in Figure 1; a TEM image of a dry film made from such a HLP is shown in Figure 2.

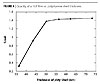

Contribution to PVC
Many film properties such as scrub resistance, stain resistance, cracking resistance, etc., correlate well to a paint’s fraction of critical PVC (FCPVC).1 Because of the utility of the fraction of critical PVC concept, it is useful to consider whether or not HLPs contribute to a paint’s PVC. HLPs are both binders and pigments; they contain both film-forming and non-film-forming components. Although the non-film-forming polystyrene shell is completely encapsulated by film-forming binder, it turns out that it is still useful to think of the polystyrene shell as contributing to overall paint PVC, i.e., if one does not assign a PVC contribution to the HLP, then the FCPVC calculated from the conventional pigments and extenders will predict better paint properties than one measures in the lab.However, if one assigns all of the volume occupied by the hard shell and the air void as contributing to PVC, then the resulting calculated FCPVC predicts paint properties that are worse than what is measured in the lab. It appears that the best approach is to assume that only a fraction of the volume occupied by the pigment part of a HLP contributes to PVC. It is not exactly clear why this occurs, but it is thought that the complete encapsulation of the pigment in binder, which prevents any pigment-pigment contact in the dried film, results in a better distribution of binder and pigment (and extender) in the film than what would occur with a random distribution, as is the case in a conventional paint.
For conventional pigments and extenders, the FCPVC of a paint can be estimated as:
FCPVC = Σ (PVCi / CPVCi) (1)
where CPVCi is the critical PVC of the ‘ith’ pigment or extender, and the sum is over all of the pigments and extenders in the paint. The CPVC of a pigment or extender is often determined by measuring some paint property as a function of PVC and looking for an inflection point in the resulting plot.1 With a HLP it is necessary to determine both the CPVC and the fractional contribution of the pigment volume to PVC. Equation (1) can then be written as:
FCPVC = Σ (PVCi / CPVCi) + α *VHLP / CPVCHLP (2)
where α is the fractional contribution of the pigment volume of the HLP to PVC, and VHLP is the percent volume of the paint occupied by the pigment part of the HLP. Unfortunately, α and CPVCHLP cannot be determined independently; it is necessary to assign a value to one of the parameters and then measure the other. We have found it most convenient to assign the CPVC value measured for 400 nm solid polystyrene beads (CPVC = 60) to a HLP, and then measure α by making a series of paints at increasing levels of some pigment or extender and measuring some paint property that correlates with FCPVC as a function of the extender PVC. The inflection point in the plot gives the pigment or extender PVC at which FCPVC=1.0, and Equation (2) can then be solved for α.
An example of such a plot, using TiO2 as the pigment and measuring abrasive scrub resistance, is shown in Figure 5.
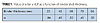
As is well known, the value obtained for a pigment’s CPVC (or in this case the value of α for a HLP) will depend somewhat on which paint property is used in the determination. In the example above, abrasive scrub resistance was used to determine α; the values obtained this way turn out to be very close to the numbers obtained using a number of other properties such as stain resistance, gloss and opacity. However, if crack resistance for exterior paints is used to determine α then a somewhat higher number is obtained. For example, for a HLP with a 60 nm binder shell α is found to be 0.55, compared to the value of 0.50 obtained using the abrasive scrub resistance test.

HLP Optical Properties
Dry HidingThe water-filled polystyrene shell in the center of a HLP is very similar in structure to the opaque polymers sold commercially as opacifying pigments for waterborne paints. Not surprisingly then, the light scattering of a HLP follows most of the same rules as light scattering from opaque polymers.2 Light scattering is caused by a difference in refractive index between the air void at the center of the HLP (η = 1.00) and the polymer surrounding the air void (η = 1.48 for the acrylic binder and η = 1.60 for the polystyrene shell). The amount of light scattered by a single HLP depends on the magnitude of the difference in refractive index of the polymer and air void (with larger differences resulting in higher scattering) and on the size of the air void. The optimum size for the air void is roughly one half the wavelength of light to be scattered, or around 280 nm for visible light.3 Air voids smaller or larger than this optimum size scatter less light per unit volume of air, and impart less opacity to a paint; this is shown in Figure 6.


S = A * T * (1 – B * T⅓) (3)
where S is the Kubelka-Munk scattering coefficient, T is the TiO2 PVC, and A and B are constants whose values depend on the grade of TiO2 and on the size and refractive index of the latex particles. In a paint made from a HLP, however, Equation (3) must be modified as:
S = (A0 + A1* F(λ)) * T * (1 – B * T⅓) + C * VHLP (4)
where A0 replaces A in Equation (3), A1 and C are constants dependant on the structure of the HLP, F(λ) is a complex function of the paint’s FCPVC, and VHLP is the volume percentage of the pigment part of the HLP in the dried film. The C * VHLP term specifies the amount of light scattering off of the air voids at the centers of the HLPs and the A1* F(λ) term specifies the amount of increased light scattering from the TiO2 particles due to the lowering of the average refractive index around them.
Although the relative magnitudes of the C * VHLP and A1* F(λ) terms will vary depending on the exact HLP and TiO2 used, as well as on the paint’s FCPVC, in typical paints with FCPVC values around 0.8 the two terms are nearly equal. This means that in paints formulated with either a HLP or a combination of conventional binder and opaque polymer such that the two paints have the same number of encapsulated air voids, the paint made from the HLP will have roughly twice the increase in hiding from the HLP than the conventional paint has from the opaque polymer.
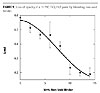
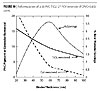
Formulating With HLPs
Both the amount of opacity provided by a HLP and its contribution to FCPVC is dependant on the binder shell thickness; thinner binder shells are preferred to maximize opacity while thicker binder shells are preferred to minimize the HLP’s contribution to FCPVC. With thinner binder shells less TiO2 is needed to achieve a given level of opacity but less extender can be used in the formulation and still obtain a given level of film quality. The balance between TiO2 removal and allowable extender PVC as a function of binder shell thickness is shown in Figure 10 for a typical HLP, where the TiO2 and extender levels are adjusted to hold opacity and abrasive scrub resistance constant as the binder shell thickness is varied. Although the exact shape of these curves will depend on the exact nature of the paint formulation and on the prices used for the raw materials, the results in Figure 10 are typical and show an optimum value of around 60 nm for the binder shell. Given this result, in considering how to formulate with HLPs and the resulting film properties of HLP-based paints we will limit the discussion to HLPs with 300 nm diameter voids, 50 nm polystyrene shells and 60 nm binder shells. With such HLPs the pigment portion of the HLP is one half the volume of the total particle, and one half of this pigment volume contributes to FCPVC, i.e., its fractional contribution to PVC (α) is 0.5.Formulating a paint with a HLP of the structure described above is very similar to formulating a paint with a conventional binder. The opacity of the HLP is augmented by adding TiO2 to the formulation until the desired level of hiding is achieved. Extenders are added to fill the paint to the desired film quality. Other formulation adjuvants such as thickeners, coalescents, defoamers, etc., are used in the normal manner.
Because part of the paint opacity is brought by the latex particles, less TiO2 is required to reach a given level of hiding power in a HLP-based paint than in a similar conventional binder-containing paint. The magnitude of the TiO2 reduction will depend on a wide range of factors, the most important being the level of TiO2 and the level of large particle size extenders in the formulation. TiO2 reductions, in formulations where the HLP can be used effectively (see sections on paint properties below), can be substantial. Such reductions in TiO2 usage can result in significant raw material savings. In general, paints with high TiO2 levels and high extender PVCs, i.e., paints in which the TiO2 is relatively crowded, will result in larger reductions in TiO2 levels with a HLP- based paint.
To understand how HLP-based paints compare to conventional paints, it is useful to consider reformulating a conventional paint with a HLP to simultaneously match dry opacity and film quality. With HLPs that have a fractional contribution to PVC of 0.5, it is generally the case that the reduction in a paint’s FCPVC caused by the reduction of TiO2 usage in replacing the conventional binder with a HLP is almost exactly offset by the increase in the paint’s FCPVC caused by the PVC contribution of the HLP. In these cases, the extender levels in the HLP paint would be the same as they were in the conventional binder-based paint to retain the initial film quality. However, when particularly large TiO2 reductions are necessary to match opacity, HLP-based paints can some times be formulated with somewhat more extender than their conventional binder-based counterparts to offset the loss of TiO2 PVC, while the reverse is true for paints with a particularly small TiO2 reduction. In these cases, it is often necessary to rebalance the mix of large and small particle size extenders, or to adjust the level of specialty flatting extenders (such as diatomaceous earths) to match the gloss level of the initial paint made with a conventional binder.
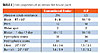
Film Properties
Interior House PaintsThe acrylic binder component of a HLP can be made to have the same composition, molecular weight, etc., of conventional binders. Therefore, just as it is possible to make conventional latex binders with a wide range of film properties, it is possible to make HLPs with a wide range of film properties. With few exceptions, reformulating a paint based upon a conventional binder/pigment combination with a HLP will reproduce a paint of similar film properties if the binder phase of the HLP is similar to the conventional binder. Table 2 shows some typical film properties of such a reformulation using a HLP with a 60 nm binder shell; the paint formulations themselves are given in Table 3. As described above, the TiO2 and extender levels in the HLP paint have been adjusted to match the opacity and abrasive scrub resistance of the paint based on the conventional binder. The other film properties of the two paints are also the same, within the noise of the experiment.
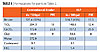
There are some exceptions to the observation that reformulations with HLPs produce paints with film properties similar to the initial conventional paint. One of these concerns the gloss level of the dried paint film. Because a HLP needs to be around 500 nm in diameter to optimize both opacity and binding capacity, one would not expect to be able to replace small particle size latexes in a high gloss paint with a HLP and match the gloss of the initial film, and this is in fact the case. However, the sheen of intermediate gloss/low PVC paints made from HLPs is even lower than one would predict based solely on the large particle size of HLPs; the reason for this is not currently understood. Calculations of the expected change in gloss that would occur by modifying the refractive index near the surface of the film predict a loss of only a few percentage points by placing air voids 100 nm or so beneath the film surface, as is the case with a HLP. Thus it seems unlikely that the effect is caused by refractive index changes alone.

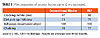
In addition to some of the properties of interest for interior house paints, exterior house paints must have acceptable cracking, dirt pick-up, and chalking resistance, as well as good tint retention and wet adhesion. Just as with interior paints, exterior house paints based on HLPs tend to perform similarly to paints based on conventional binders, with one notable exception. Typical results are shown in Table 5. The one difference in performance of exterior house paints based on HLPs is with tint retention.
Tinted paints that contain only TiO2 as the white pigment will lighten in color with time as the photoactive TiO2 pigment causes chalking of the film surface. It is well known that paints containing a mixture of TiO2 and opaque polymer will initially darken slightly upon exterior exposure, and then will reverse course and begin to lighten as chalking by the TiO2 begins to dominate.
Paints made from HLPs effectively contain high levels of opaque polymers, and generally contain lower levels of TiO2 than conventional paints. On exterior exposure they behave like opaque polymer containing paints only with a larger initial darkening and a delayed reversal in color change due to chalking. The magnitude of this effect will depend on the paint’s overall PVC and TiO2 level, as well as on the binder composition and degree of TiO2 photoactivity.
Conclusions
Hollow latex particles are a new class of raw materials for paints that function as both binder and pigment. The binder part of a HLP functions similarly to conventional binders of the same composition and structure. The pigment part of a HLP resembles an opaque polymer pigment particle, but because of the unique morphology of a HLP it provides twice the opacity at one half the contribution to PVC that conventional opaque polymers provide. This allows the HLP to be used as a sole binder in a paint, effectively bringing a large number of pigment particles into the formulation without a large increase in PVC. The opacity contribution of the HLP to a paint allows for a large reduction in TiO2 usage for a given level of hiding, with the potential for substantial raw material cost savings. The gloss potential of paints made from HLP is low, as is the burnish resistance of low PVC paints made with HLPs, which probably limits the current generation of HLPs to use in flat paints. However, flat paints made with HLPs offer properties comparable to those made from conventional binders.This paper was presented at The Waterborne Symposium sponsored by The University of Southern Mississippi School of Polymers and High Performance Materials and The Southern Society for Coatings Technology, 2008, New Orleans, LA.
Report Abusive Comment