Fungal Deterioration of Anti-Corrosive Coatings
Assessment by Electrochemical Techniques and Scanning Electron Microscopy

Microorganisms grow on all kinds of materials, including metals, inorganic minerals and organic polymers under humid conditions. 1 A complex community of microorganisms and their extracellular polysaccharides established as biofilm is a prerequisite for corrosion and/or deterioration of the underlying materials. 2, 3 This affects great-economic-value metallic structures like bridges and pipes; 70% of the corrosion in gas transmission lines are due to problems caused by microorganisms. 4,5 Metallic structures are generally protected by anti-corrosion coatings, but biofilm over these coatings can lead to blistering and adhesion loss, causing failure of the protective system. 6
Filamentous fungi are regarded as the microorganisms that cause the most spoilage of paint films. 7 They are eukaryotic microorganisms that have extensive vegetative phase networks (mycelium) that release enzymes, glycoproteins and organic chelators. 8 Therefore, they are actively involved in corrosion processes because they can modify the substrates they grow on by chemical degradation and differential aeration and cracked zones. 4,9 In this sense, localized corrosion has been registered in a spatial relationship with fungal hyphae. 10 Fungi grow in a wide pH range and are the most desiccant-resistant microorganisms, remaining active at moisture levels too low for bacteria, and also form desiccation-resistant spores. 11,12 Fungal-influenced corrosion (FIC) can be defined as an electrochemical process in which the participation of fungi is able to initiate, facilitate or accelerate the corrosion reaction. 4 Therefore, the metallic protection of substrates requires a more comprehensive view as regards to the use of special coatings to prevent their FIC, taking into account the fungal susceptibility of the protective systems in order to prevent future failures that lead to efficiency loss.
This work deals with the assessment of fungal deterioration on different anti-corrosive coatings using electrochemical techniques (corrosion potential and ionic resistance). First, fungal isolates were obtained from biodeteriorated paint films following conventional microbiological techniques. The most frequent and deteriorating fungi, in relation to the subject studied, were selected and identified. The identification was based on micro and macro morphological observations. 13,14 Molecular analysis of the isolated strains was also done to support their identification.
Selected fungi of the genera Aspergillus (H1) and Penicillium (H2) were used to inoculate the evaluated paint films. Coatings containing various types of anti-corrosive pigments (zinc phosphate and modified zeolite with rare earth ions) and a coating without anti-corrosive pigment were studied. It should be considered that in the past, paints formulated with zinc chromate prevented microbiological growth and were considerably more resistant to deterioration. 6 Due to the high toxicity of chromates and their subsequent prohibition, new anti-corrosive pigments are intensively investigated. The employment of extender pigments modified with rare earth metal (REM) cations such as cerium or lanthanum may constitute an alternative to phosphates as they are found to possess excellent inhibitive properties and can be considered as “green” inhibitors. 15-18
The inoculated samples and the corresponding controls were arranged in containers in order to regulate the internal relative humidity. 19 After two months, electrochemical measurements were made on the inoculated test substrates. Results showed that the electrochemical techniques are useful to differentiate the fungi degrading activity of different fungal species on the anti-corrosive tested paints.
Materials and Methods
Isolation, Identification and Molecular Analysis of Fungi
Samples of biodeteriorated painted panels from La Plata city (34º54’S and 57º55’W) were taken by swabbing and diluted with physiological solution (PS). 100 L of the dilutions were seeded on plates with a rose bengal-based medium (BR) (2.0 g agar, 2.5 mg rose bengal, 1.0 g dextrose, 0.5 g peptone protease, 0.1 g KH 2 PO 4 , 0.05 g of MgSO 4 .7H 2 O and distilled water to 100 mL). In addition, an antibiotic was added to the culture medium in order to prevent bacterial growth. BR restricts colony spreading without affecting spore germination. The plates were incubated at 28 °C for at least 72 hrs to proceed with the isolation of fungi by conventional microbiological techniques. 13
The fungi were identified based on their micro and macro-morphological characteristics, using standard taxonomic keys. 13,14 To improve the isolates, observation of the microcultures and staining with cotton blue were carried out. The most frequent and deteriorating fungi were selected.
The fungi selected were subjected to molecular analysis by direct sequencing of fungal ITS regions amplified by PCR (Macrogen-Korea) to confirm identification. 20 The primers used were ITS1 (5´-TCC GAT GGT GAA CCT GCG G-3´) and ITS4 (5´-TCC TCC GCT TAT TGA TAT GC-3´). The sequences were analyzed by Blast searches using the GenBank database 21 to reveal the closest known analogue.
In order to evaluate the deteriorating activity, the fungi isolated were grown on the same medium as before, BR, but without rose bengal adding 0.1% w/v of CaCO 3 and incubated for 7 days at 28 °C. The halo formation around the mycelium proves the release of acids by the fungus tested.
Formulation and Preparation of Paints
Selection of the Anti-Corrosive Pigments
Three different anti-corrosive pigments were selected. One was the traditional zinc phosphate and the other was one of its replacements, lanthanide-exchanged zeolite. Zinc phosphate has raised some environmental concerns because phosphate causes the eutrophication of water courses, and zinc itself is toxic, so replacements are sought. On the other hand, cerium and lanthanum-exchanged zeolites are being studied as alternative inorganic green inhibitors, zeolite being a natural mineral and the lanthanide ions less toxic than sodium. 15 The obtaining method for these pigments are detailed elsewhere. 17,18
Formulation, Preparation and Application of Paints
Table 1 shows paint compositions. In all cases, the content of anti-corrosion pigment was 30% relative to the total volume of the pigment mixture. This value is often recommended for phosphate pigments. 22-24 The anti-corrosion pigments used were: zinc phosphate (PZ20, SNCZ), a modified zeolite with Ce(III) ions (ZCe) and a zeolite modified with La(III) ions (ZLa). Both exchanged zeolites were prepared in the laboratory. 17 Non-anti-corrosion pigment paint was also formulated and prepared. Pigment formulations were completed with barium sulphate, titanium dioxide and talc. A medium oil, solvent-based alkyd resin (Alkypol 434/50) was added to maintain a PVC/CPVC ratio (pigment volume concentration/critical pigment volume concentration) of 0.7, and mineral spirit was employed as solvent. Different additives were used: wetting and dispersing (TEGO 652, 1% v/v), substrate humectant (TEGO 270, 1% v/v), levelling (TEGO 270, 1% v/v) agents, cobalt- (Casal del Rey, 0.06% v/v) and calcium-based driers (Casal del Rey, 0.12% v/v).
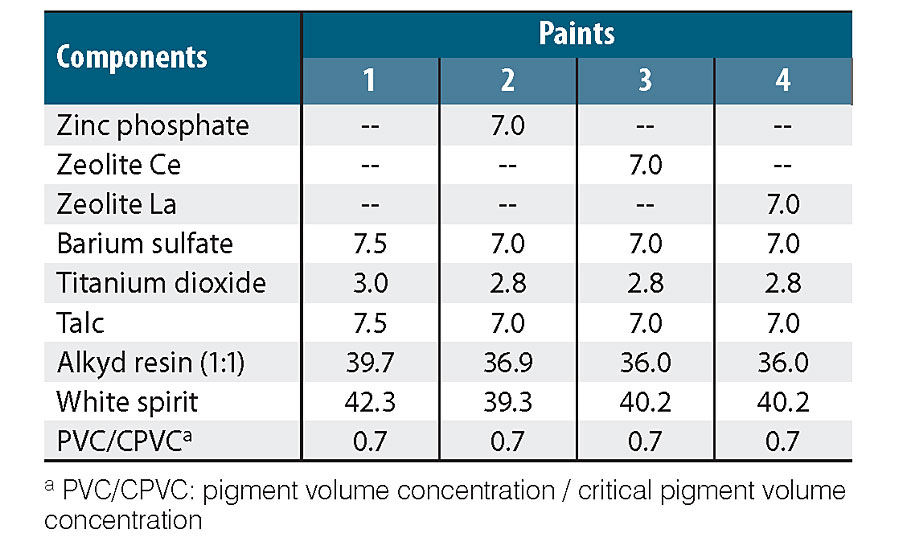
In all cases, paints were prepared in a ball mill, 25,26,17 allowing pigments to be dispersed in the vehicle (solvent and resin) for 24 hrs until reaching an acceptable dispersion degree (grade 5 on Hegman scale).
SAE 1010 (15.0 x 7.5 x 0.2 cm) steel panels were sandblasted to Sa 2 ½ grade (SIS 05 59 00 standard), degreased with toluene and painted with the formulated paints. The dry film thickness was 80 ± 10 μm, and painted panels were maintained for 7 days in a laboratory environment (20 ± 2 °C and 65% relative humidity) before being tested.
Assessment of Fungal Biodeterioration by Electrochemical Methods
A methodology similar to that used by E. Juzeliunas et al. 2007, was followed. 19 First, suspensions of selected fungi were prepared from the cultures of the selected fungi in the BR-based medium incubated at 28 °C for 15 days. The conidia concentration of 10 5 /mL was adjusted with a Neubauer chamber. The suspension solution composition used was: 5.0 g NaCl, 1.0 g HK 2 PO 4 , 1.0 g (NH 4 )H 2 PO 4 , 1.0 g (NH 4 ) 2 SO 4 , 0.2 g MgSO 4 , 3.0g of KNO 3 , 0.05 g of Tween 20 per 1,000 mL of distilled water.
Three culture cells were built on each panel, delimiting 3 cm 2 circular zones on the painted surface with an acrylic tube (7.0 cm high). These cells were irradiated with a germicide UV lamp (Philips, 20W) for 40 min on each side and inoculated with 100 µL of fungal suspensions. Control with 100 µL of the sterile solution without fungal spores was prepared too. The samples were disposed in 5L-capacity environmental chambers (EC) with 1L of a saturated K 2 SO 4 solution to maintain relative humidity at 97%. Then, the chambers were closed and kept in laboratory conditions at 25± 2 °C for 2 months before making the corresponding electrochemical measurements. Three chambers were employed to prevent contamination, one with the control samples and the others with each selected fungi. The time was stipulated based on bibliographic data and taking into account a preliminary evaluation of the methodology used. 19
The evaluation of the fungal deterioration was carried out by measuring the ionic resistance of the paint film and the corrosion potential of the painted substrate. These variables were selected taking into account that metal protection by coatings is afforded by a barrier effect and an anti-corrosion action due to the presence of an active pigment. 27-29 The measurement of the ionic resistance is a good tool to study the barrier effect as it diminished according to the water entrance due to the presence of pores or the increased of coating permeability. The barrier effect delays water entrance but, once water has reached the metal surface, the anti-corrosion pigment action is the main protective effect. 28
The resistance between the painted steel substrate and a platinum electrode was measured with an ATI Orion Conductor Model 170 with a frequency of 1,000 Hz. The corrosion potential was monitored with a high-input impedance voltmeter against a saturated calomel electrode (SCE). Before the measurements, the acrylic tubes were filled with 10 mL of PS, used as electrolyte.
After measurements, samples from supernatant close to the paint film were taken with a pipette and plated with BR (without rose bengal) to verify the lack of microbiological growth in the control specimens and the growth of the fungi inoculated at the beginning of the assay in the other cases.
Observation by Scanning Electron Microscope (SEM)
The samples exposed for 2 months in the EC were observed by SEM. The working conditions were low vacuum (10 -2 -1 Torr) and the microscope was a Philips FEI Quanta 200. The observation conditions were established on the basis that these kinds of samples do not require dehydration to keep their natural morphology.
The paint was removed by tweezers from the panels, and the metal underneath also observed by SEM.
Results and Discussion
Molecular Analysis and Identification of Fungi
The most frequent and deteriorating fungi found were selected and subjected to molecular analysis. Figure 1a and c show 1-week cultures in BR of selected isolates. Some observations of the microcultures by optical microscopy are shown in Fig. 1b and d. In these images, conidiophores with characteristic form corresponding with the genus Aspergillus and Penicillium 13 , 14 can be seen. Spherical form conidia in both cases were also observed. Isolate from the genus Aspergillus was the most frequent, and Penicillium was the more deteriorating due to the large quantities of acidic compounds released, which was evidenced when growth in BR supplemented with 0.1% w/v CaCO 3 . At the end of the incubation week, a halo was formed around the mycelium as a result of the reaction of the acids released with CaCO 3 , as can be seen in Fig. 1e. This leads one to think about its corrosive potential against metals.
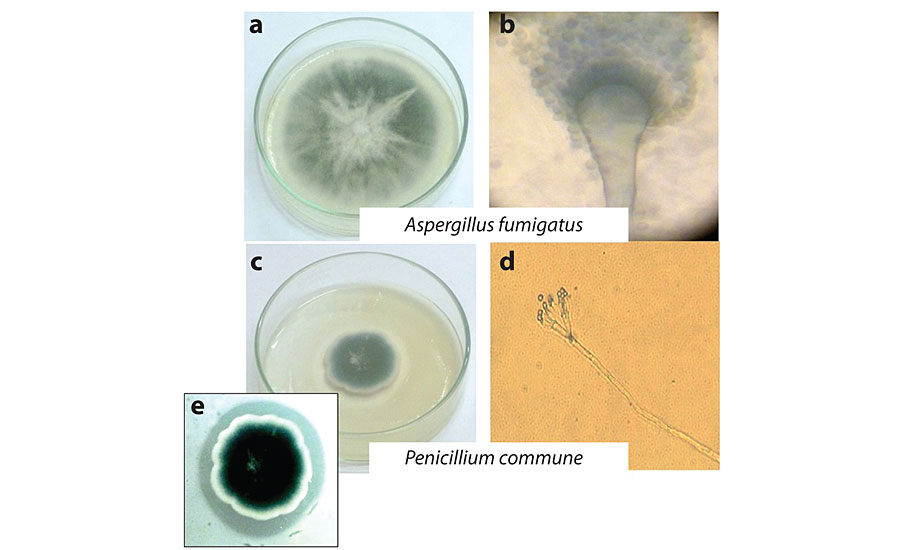
ITS regions of the ribosomal DNA sequences from isolate strains selected to perform the bioassays were analyzed by a Blast search of sequences available in the NCBI database. Identification of fungi based on the closest match of ITS sequences in the GenBank data base resulted as Aspergillus fumigatus and Penicillium commune with the accession numbers AB976023.1 and HQ652873.1, and a similarity to known species of 97% and 98% (E=0), respectively. Morphological analysis supported identification obtained from the NCBI sequence data. Sequences obtained have been deposited in the GenBank database, and the accession numbers KU936230 and KU936231 to the isolated fungi, A. fumigatus and P. commune , were assigned respectively.
Assessment of Fungal Biodeterioration by Electrochemical Methods
After the incubation period of the test specimens, the corresponding electrochemical measurements were performed. Figure 2a and b show the ionic resistance (Ri) and corrosion potential (Ec) measurements, respectively.
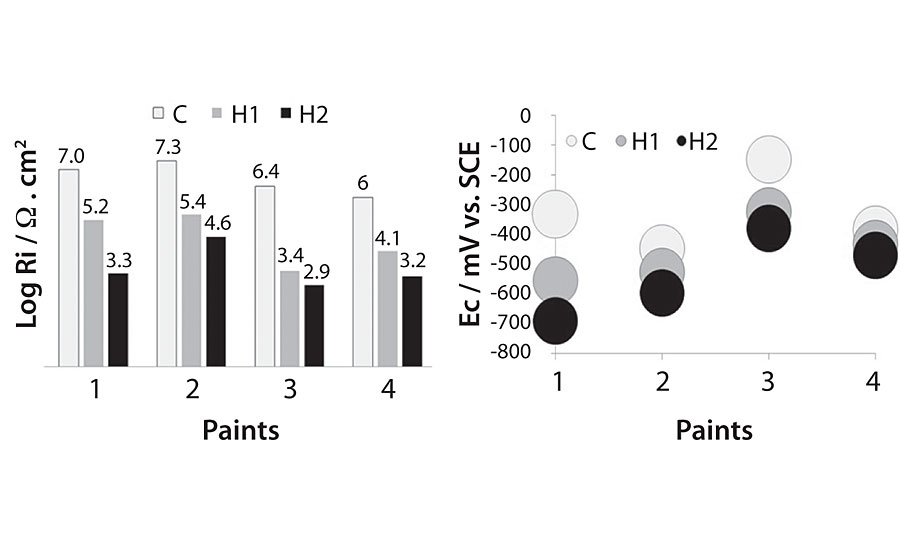
It can be observed that the ionic resistance and corrosion potential values follow the same tendency in all paints. Ri values registered for the control were between 10 6 and 10 7 Ω.cm 2 , whereas for the paints in contact with A. fumigatus the Ri values were more than one order of magnitude lower (10 3 and 10 5 Ω.cm 2 ) and even lower for paints in contact with P. commune (10 3 and 10 4 Ω.cm 2 ). The corrosion potential values for all the painted panels were, in general, more negative for the inoculated ones in respect to the controls.
The differences in Ri and E for the controls are due to the different protection afforded by the anti-corrosive pigments. 17,30 These results show that the presence of the fungi used in this study modifies the paint films by reducing their barrier capacity (generating pores and opening channels through the paint, due to the penetration of the hyphae and facilitating the water entrance or increasing the permeability), and accelerating the initiation of the corrosion process as water penetrates the coating easily, reaching the metal substrate sooner. Additionally, the release of acids by the fungi enhances corrosion. 31
The PS extracted from each sample, plated and incubated at 28 ºC for 48 hrs, showed that the inoculated paints presented positive results (growth was observed) on all plates, while control paints (not inoculated) were negative (no growth) after 2 months of exposure under pre-established conditions. These results agree with the measurements of ionic strength and corrosion potential in the sense that the values of higher resistance and more positive corrosion potentials were recorded for panels where no fungal growth was observed (controls).
The lowest Ri measurements and the most negative Ec values were recorded from panels in contact with P. Commune , indicating the more deteriorating action of this fungus on the studied paints. This result agrees with the one obtained with CaCO 3 , that showed the more deteriorating activity of this.
Observation by Scanning Electron Microscope (SEM)
Figure 3, 4, 5 and 6 show SEM micrographs of a non-inoculated (a) painted panel (with Paints 1, 2, 3 and 4 respectively) and another inoculated with P. Commune of the same formulation (b) after 2 months. Also, micrographs of the exposed metal substrates after inoculated paint removal can be observed.
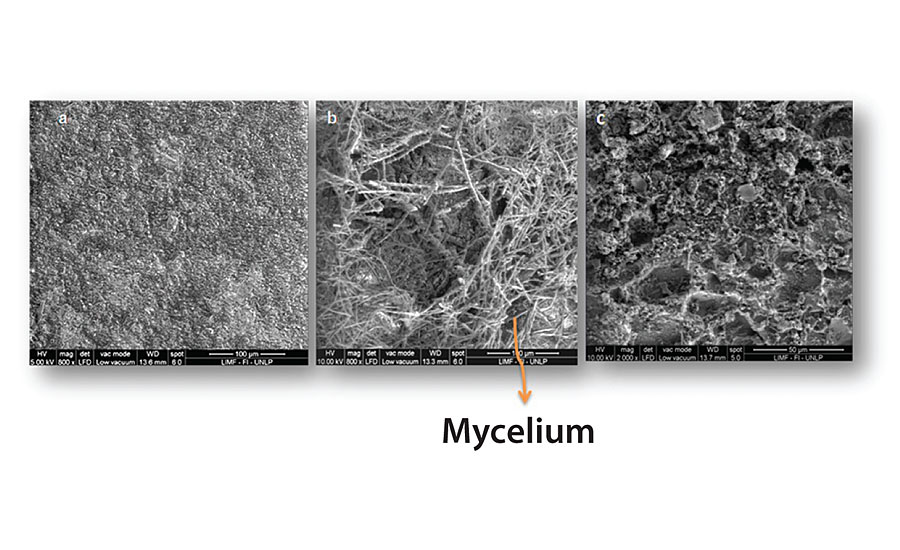
Over the non-inoculated substrate, no fungal growth was observed, as expected, in all cases. All inoculated paints presented some grade of fungal development, which was lower in the case of Paints 2 and 3 (Figure 4 and Figure 5), compared with the others. The most abundant biofilms were observed on the control paint (Fig. 3b), and especially on Paint 4, which showed a high grade of fungal development with a great amount of specialized reproductive hyphae and conidia scattered (Figure 6b). This paint showed a greater deterioration degree in relation to the rest. An invasive growth of the inoculated fungus can be seen crossing the paint film until reaching the metallic substrate where an abundant mycelium and conidia can be seen (Fig. 6c). These microscopy observations are consistent with the ionic resistance values measured, being the lowest of all tested paintings.
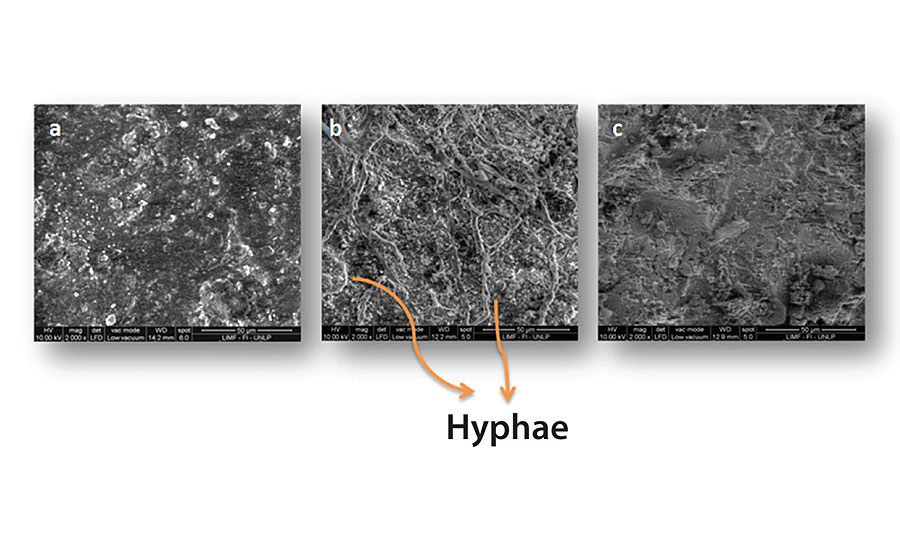
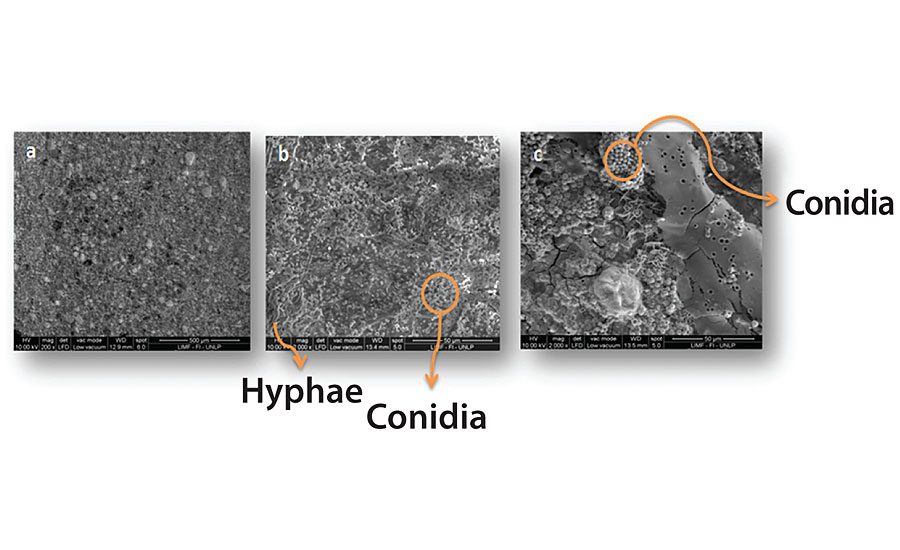
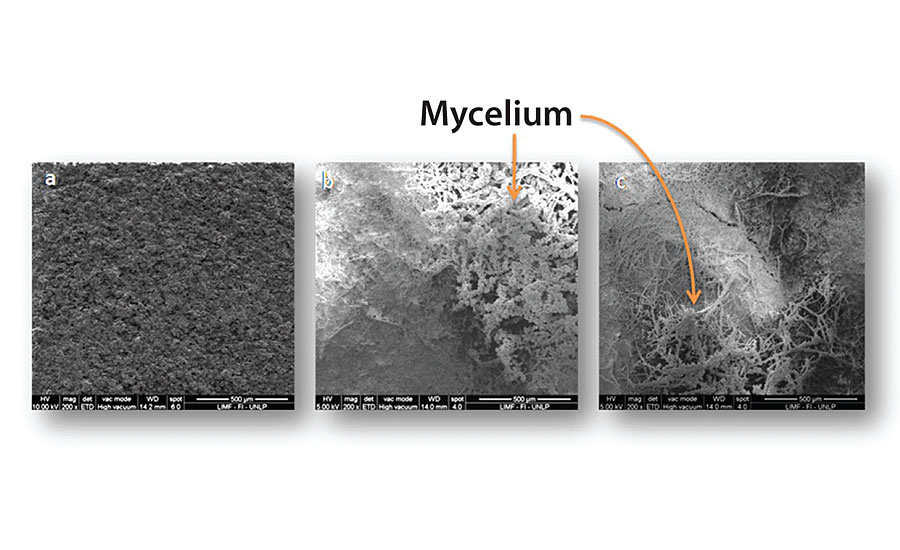
Conclusions
It was possible to isolate and identify by molecular methods frequent and deteriorating fungal specimens, A. fumigatus (KU936230) and P. commune (KU936231) from coating samples placed in La Plata city.
The electrochemical tests have shown that coating ionic resistance decreases in the presence of A. fumigatus and P. commune , and the corrosion potential tends towards more negative values, except for paint containing 30% of the cerium ion exchanged zeolite.
Fungal growth on inoculated painted panels could be observed (macro and microscopically). These results were consistent with recorded values by electrochemical measurements.
It was possible to evaluate the biodeterioration caused by fungal biofilms on painted steel substrates by electrochemical techniques.
It would be appropriate to consider the addition of biocides in the anti-corrosive paint formulations.
For more information, e-mail c.deya@cidepint.ing.unlp.edu.ar.
References
1 Gu, J. Microbiological Deterioration and Degradation of Synthetic Polymeric Materials: Recent Research Advances. Int. Biodet. Biodeg. , 52, 2003 , 69-91.
2 Zhou, E.; Li, H.; Yang, C.; Wang, J.; Xu, D.; Zhang, D.; Gu, T. Accelerated Corrosion of 2304 Duplex Stainless Steel by Marine Pseudomonas Aeruginosa Biofilm. International Biodeterioration & Biodegradation , 127, 2018 , 1-9.
3 Zhang, H.; Tian, Y.; Wan, J.; Zhao, P. Study of Biofilm Influenced Corrosion on Cast Iron Pipes in Reclaimed Water. Applied Surface Science , 357, 2015 , 236-247.
4 Geweely, N.S.I. Evaluation of Ozone for Preventing Fungal-Influenced Corrosion of Reinforced Concrete Bridges over the River Nile, Egypt. Biodeg ., 22, 2011 , 243-252.
5 Myer, K. Handbook of Environmental Degradation of Materials Norwich, New York, USA, William Andrew, 2005.
6 Stranger-Johannessen, M.; Norgaard, E. Deterioration of Anti-Corrosive Paints by Extraceilular Microbial Products. Int. Biodet ., 27, 1991 , 157-162.
7 Gaylarde, C.C.; Gaylarde, P.M. A Comparative Study of the Major Microbial Biomass of Biofilms on Exteriors of Buildings in Europe and Latin America. Int. Biodet. Biodeg . , 55, 2005 , 131-139.
8 Usher, K.M.; Kaksonen, A.H.; Cole, I.; Marney, D. Critical Review: Microbially Influenced Corrosion of Buried Carbon Steel Pipes. Int. Biodet. Biodeg ., 93, 2014 , 84-106.
9 Zarasvand, K.A.; Rai, V.R. Microorganisms: Induction and Inhibition of Corrosion in Metals. Int. Biodet. Biodeg ., 87, 2014 , 66-74.
10 Little, B.J.; Gerke, T.L.; Ray, R.I.; Lee, J.S. The Mineralogy of Microbiologically Influenced Corrosion, Mineral Scales and Deposits Elsevier B.V ., 2015 .
11 Little, B.; Staehle, R.; Davis, R. Fungal in Uenced Corrosion of Post-Tensioned Cables. Int. Biodet. Biodeg., 47 , 2001 , 71-77.
12 Olaf, C.G.A.; Samson, R. Fundamentals of Mold Growth in Indoor Environments and Strategies for Healthy Living, Netherlands, Wageningen Academic Publishers, 2011.
13 Pitt, J.I.; Hocking, A.D. Fungi and Food Spoilage, New York, Ed. Springer, 2009.
14 Samson, R.A.; Hoekstra, E.S.; Frisvad, J.C.; Filtenborg, O. Introduction to Food-Borne Fungi, Netherland, CBS, 1995.
15 Bethencourt, M.; Botana, F.; Calvino, J.; Marcos, M.; Rodriguez-CHACON, M.A. Lanthanide Compounds as Environmentally Friendly Corrosion Inhibitors of Aluminium Alloys: A Review. Corr. Sci ., 40, 1998 ,1803-1819.
16 Kesavan, D.; Gopiraman, M.; Sulochana, N. Green Inhibitors for Corrosion of Metals: a Review. Chem. Sci. Rev . Lett., 1, 2012 , 1-8.
17 Roselli, S.; Bellotti, N.; Deyá, C.; Revuelta, M.; Del Amo, B.; Romagnoli, R. Lanthanum-Exchanged Zeolite and Clay as Anti-Corrosive Pigments for Galvanized Steel. J. of Rare Earths , 32, 2014 , 352-359.
18 Roselli, S.; Deyá, C.; Revuelta, M.; Di Sarli, A.R.; Romagnoli, R. Zeolites as Reservoirs for Ce(III) as Passivating Ions in Anticorrosion Paints. Corrosion Reviews , 36, 2018 , 305-322.
19 Juzeliunas, E.; Ramanauskas, R.; Lugauskas, A.; Leinartas, K.; Samulevičienė, M.; Sudavičius, A.; Juškėnas, R. Microbially Influenced Corrosion of Zinc and Aluminium – Two-Year Subjection to Influence of Aspergillus Niger. Corr. Sci ., 49, 2007 , 4098–4112.
20 Peterson, R.A.; Bradner, J.R.; Roberts, T.H.; Nevalainen, K.M.H. Fungi from Koala (Phascolarctos cinereus) Faeces Exhibit a Broad Range of Enzyme Activities Against Recalcitrant Substrates. Lett. App. Microbiol ., 48, 2009 , 218-225.
21 Chandler, K.A.; Hudson, J.C. Iron and Steel. In: Shreir, L. L. (ed.) Corrosion . Sussex: Newnes-Butterworths, 1976 .
22 Bittner, A. Advanced Phosphate Anti-Corrosive Pigments for Compliant Primers J.C.T. , 61, 1989 , 111-118.
23 Gerhard, A.; Bittner, A. Second Generation Phosphate Anti-Corrosive Pigments. Formulating Rules for Full Replacement of New Anti-Corrosive Pigments. J.C.T. , 58, 1986 , 59-65.
24 Romagnoli, R.; Vetere, V.F. Heterogeneous Reaction Between Steel and Zinc Phsophate. Corrosion NACE, 51, 1995, 116-123.
25 Del Amo, B.; Blustein, G.; Deyá, M.C.; Romagnoli, R. Zinc Molybdenum Phosphate: an Effective Anti-Corrosive Pigment for Solvent and Waterborne Paints. Corr. Rev. , 22, 2004 , 127-143.
26 Del Amo, B.; Romagnoli, R.; Deyá, C.; González, J.A. High Performance Water-Based Paints with Non-Toxic Anti-Corrosive Pigments. Progr. Org. Coat. , 45, 2002 , 389-397.
27 Johnson, W.C. Cost Effective Pigmentation in Alkyd Primers for Steel: Barrier Anti-Corrosion Mechanism. J.C.T. , 66, 1994 , 47-54.
28 Mayne, J.E.O. The Mechanisms of the Protective action of Paints. In: Shreir, L. L. (ed.) Corrosion. London: Butterworth-Heinemann Ltd., 1976 .
29 Sangaj, N.S.; Malshe, V.C. Permeability of Polymers in Protective Organic Coatings. Progr. Org. Coat ., 50, 2004 , 28-39.
30 Roselli, S.N.; Deyá, C.; Romagnoli, R. Tierras Raras Como Pigmentos Aanticorrosivos Para la Elaboración de Pinturas Inteligentes. 7mo Congreso de Medio Ambiente, AUGM. La Plata, Argentine, 2012.
31 Otero Huerta, E. Corrosión y Degradación de Materiales, Madrid, Spain, Sintesis, 1997.
Looking for a reprint of this article?
From high-res PDFs to custom plaques, order your copy today!