Synthesis and Application of Phase-Separated Acrylic Copolymer Dispersions

Sequential polymerization technique is a very powerful tool for polymer structure creation and allows the preparation of dispersions, polymer films and coatings with the desired balance of physical, chemical and mechanical properties. Core-shell species are the best-known phase-separated copolymers. The differences in core and shell composition are responsible for film forming, anti-blocking and optical properties, hardness, resistance, adhesion and ability to be spray-dried8,9. Structured polymers in the form of dispersions or redispersible powders (after the spray-drying step) are characterized by unique properties often superior to blends. One of the potential applications for those types of dispersion is the formulation of cosolvent-free paints and coatings. Other important and very new fields of interest are the application of structured polymers, mainly in powder form, in biology, biochemistry and medicine as nanoscale carriers for drug molecules, catalysts and viruses 10,11.
The aim of this work was to present a synthesis of sequential copolymer dispersions and their possible application as a paint binder and cement additive in the powder form after drying. Multi-stage polymerization procedures together with acid functionalization were chosen in order to reduce the tackiness of the final polymer and to promote the low-temperature film formation of the latex and the water redispersibility of the powder.
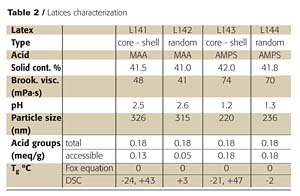
Latices Preparation and Characterization
Experimental latices were prepared by a multi-step sequential polymerization process described previously7,12. Polymer particles consisted of hard, high Tg shells containing free acid groups and soft, low Tg hydrophobic cores. Typically shell mass was 1/3 of total polymer weight. Examples of low Tg (about 0 ºC as a mean value) core-shell polymer composition are presented in Table 1. As acid functionality, two types of acid co-monomers were used: methacrylic acid (MAA) and 2-acrylamido-2-methylopropane sulfonic acid (AMPS); other monomers used were methyl methacrylate (MMA) and butyl acrylate (BuA). Random copolymers of the same chemical composition were also synthesized and used as a reference point to core-shell latices. Obtained latices were characterized by solids content, Brookfield viscosity, pH, particle size, Tg and acid group concentration/distribution (Table 2).Content of acid groups located on the particle surface or in the water phase (accessible acid groups) was measured via pH-metric titration with 0.1 N NaOH aqueous solution; total acid content was established by means of tetra-n-butylammonium hydroxide 0.1 N aqueous solution13. In AMPS both core-shell and random latices all acid groups were easily accessible for sodium hydroxide titre. This phenomenon is related to the hydrophilic nature of completely dissociated sulfonic groups from AMPS units located externally on the particle surface or in the water phase. In the case of MAA functionalized latices, a certain amount of acid units were buried inside polymer particles. In core-shell dispersion L141 about 70% of acid groups were able to react with sodium hydroxide solution, in the case of random L142 copolymer only 25% of the acid groups were available. Lower ionization of carboxylic groups during the polymerization process is responsible for the described behavior.
Particle sizes were measured with a Coulter N4 Plus dynamic light-scattering analyzer. Differences were found between MAA and AMPS functionalized latices. AMPS tendency for polymerization in the water phase is leading to a bigger amount of smaller polymer particles than in the case of MAA latices.
Glass transition temperatures were determined by differential scanning calorimetry (DSC) by means of a Perkin Elmer Model Pyris 1, Tg mean values were calculated from the Fox equation14. Multi-phase structure has been evidenced by means of DSC analysis. Films prepared from latex L141 showed two transition points (-24 ºC and +43 ºC), characterizing different phases of polymer structure.
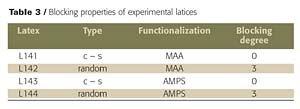
Latices Application
Waterborne varnishes and enamels are used for external and internal wood decoration. Binders for such applications should have superior anti-blocking properties. Mostly high-MFFT latices are applied together with a proper coalescing agent to improve film formation at low application temperatures. Coalescing agents reduce the glass transition temperature of the latex, decreasing the MFFT. After film formation, coalescent evaporates and the coating becomes harder. From another point of view, the reduction of VOCs is one of the biggest challenges in the coating industry. To prepare coatings without solvents, the binder needs to have a low MFFT. A core-shell technology could be a solution combining a required blocking resistance with suitable MFFT value.To evaluate anti-blocking behavior all obtained latices were tested as a clear binder diluted to 30% solids. Experiments were carried out in an air-conditioned room (23 °C and 50% RH). Dispersions were applied twice by brush on the smooth pine panels (20 x 10cm). After second coat application all panels were dried 24 hours. Random copolymer was giving a little bit of a tacky surface, whereas the core-shell dispersion produced a rigid, well-developed coating. To assess anti-blocking properties in a semi-quantitative way, two panels derived from the same dispersion were placed perpendicular to one another with coatings in contact and subjected to a 2 kg load for 8 hours. In each case the contact area was 100 cm2. Then the ease of manual panel separation was tested and results expressed in points from 0 up to 3:
- 0 - means no blocking (separation with no evidence of sticking)
1 - means slight blocking (little effort required for panels separation)
2 - means moderate blocking (medium effort required for panels separation)
3 - means strong blocking (very difficult panels separation).
A chosen heterogeneous dispersion (L143) has been applied as a binder in a white gloss enamel formulation (PVC = 18%, binder content = 28%, density = 1.26 g/cm3, viscosity 23 ºC (Cone & Plate) = 0.30 mPa·s). Prepared paint was applied on the window frame. After 10 hours from second coat application, the window was closed and the next day no blocking effect was observed. This practical test showed that the unique properties of phase-separated copolymer is potentially very useful as a binder for low, or 0%, VOC paints for wood decoration and protection.

Powder Preparation From Experimental Latices
Experimental dispersions were transformed into free-flowing powders according to procedures described in the literature9. The latices neutralized with calcium hydroxide and diluted to 30% solids, were formulated with 5% (on solid polymer) of calcium carbonate (Microcal 140) and 0.2% of antifoam to be spray dried. A Lab Plant Mod. SD04 laboratory spray drier, equipped with 0.7 mm static nozzle, was used for that purpose. The feeding rate of process was 300 g/h of formulated dispersion at a temperature range of -110 ºC to 130 ºC. In the case of random copolymers, the process was stopped almost immediately because of nozzle blocking. In contrast, the structured copolymer dispersions were converted into powders with high 85% yield without blocking the drying equipment. The results (see Table 4) additionally confirm the heterogeneous structure of polymer particles prepared in the multi-step polymerization technique and are in good agreement with our earlier observations9,12. Powders (P141 & P143) were formulated with 0.5% of microsilica (Silcasil S) to improve the free-flowing properties. The experimental products were fully redispersible in water according to a test described previously by the authors7. Investigation by transmission electron microscopy (TEM) did not reveal any significant differences in the particle size distribution between the original latex and the redispersed one. It was noted during storage that no sticking and blocking was observed, and flowability of low Tg powders was comparable with the conventional high Tg products present on the market.Powders Application
Powders P141 and P143 were tested as cementitious mortar modifiers for concrete repair. Samples were prepared in a Hobart type mixer using 425 Portland cement and sand with particle size from 0.1 to 2.0 mm and a specific gravity of 2.68 g/cm3 according to described procedure12. A typical repairing mortar was prepared with a sand/cement ratio 3 and a polymer/cement ratio of 0.07. The water level was adjusted to obtain a proper flow, and a defoamer (Lumiten EP 3108) was used to achieve wet densities higher than 2.1 g/cm3. Mortar specimens (4 x 4 x 16cm) were prepared and after 28 days of drying (23 ºC, 50% RH) were tested for mechanical properties. Samples showed better adhesion, flexibility and waterproofing properties, lower chloride penetration and better freeze-thaw stability than unmodified material. Some results obtained with experimental polymer powders are presented in Table 5. During fresh mortar preparation a positive water reducing effect was observed together with better workability of obtained mortar. In comparison with unmodified mortar, the compressive strength of polymer-modified samples was very close to the original one.Conclusions
Multi-step sequential polymerization technique is a useful tool for synthesis of phase-separated dispersions. Because of distinct morphology, these polymers show different physical and chemical properties than the corresponding random species. The distribution of MAA acid units depends strongly on polymerization methods; in the case of water-soluble strong AMPS acid, all acid groups are accessible in the aqueous environment independently from the synthesis processes.Core-shell latices with differentiated morphology in terms of Tg are promising binders with excellent blocking properties for paints and varnishes for wood decoration. The possibility of influence on MFFT value could be important for environmentally friendly, 0% VOC paints.
Low Tg phase-separated latices undergo an easy spray-drying process leading to redispersible free-flowing powders. Such powders may be applied in dry paint compositions and as cementitious admixtures in modified mortars.
During core-shell binder application on the wood panel as well as during the spray-drying process or storage of prepared powder, very good anti-blocking and anti-sticking properties have been observed. A properly designed core-shell structure is responsible for such behavior.
For more information, contact the authors at maciej_uminski@ici.com and leo-mario.saija@cray valley.com.
This paper was presented at the 5th International Conference Advances in Coatings Technology (ACT '02), November 5-8, 2002, Katowice, Poland.
References
1 Schwartz, M. Acrylic Latex Paints: A Comparison Study (Part 1), European Coatings Journal. 112-119, no 3 (2001).
2 Sohrantz, J. J. Mod. Paint Coat. 1995, 85, 65.
3 Tsai, M. Ch.; Burch, M.J.; Lavelle, J.A. In Polymer-Modified Hydraulic Cement Mixtures; Kuhlmann, L.A.; Walters, D.G. eds. ASTM: Philadelphia, 1993; pp 63-75.
4 Aguiar, A.; Gonzalez-Villegas, S.; Rabelero, M.; Mendizabal, E.; Puig, J.E.; Dominguez, J.M.; Katime, I. Core-Shell Polymers with Improved Mechanical Properties Prepared by Microemulsion Polymerization, Macromolecules, 32, 6767-6771 (1999).
5 Bückmann, F.; Overbeek, A.; Nabuurs, T. Self-Crosslinking Surfactant-Free Acrylic Dispersions. European Coatings Journal, 53-60, no 6 (2001).
6 Pieh, S.A.; Saija, L.M. High Flexible Polymer-Cement Composites, Conchem J., 2, 51-54 (1994).
7 Saija, L.M.; Umi´nski, M. Water-Redispersible Low Tg Acrylic Powders for the Modification of Hydraulic Binder Compositions. Journal of Applied Polymer Science, 71, 1781-1787 (1999).
8 Desor, U.; Krieger, S. Waterborne Acrylic Dispersions for Wood Lacquers. European Coatings Journal, 920-923, no 12 (1996).
9 Umi´nski, M.; Saija, L.M. Spray Drying of Low Tg Acrylic Dispersions. Surface Coat. Int., 81, 557-560, November 1998.
10 Wooley, K.L.; Zhang, Q.; Liu, J.; Ma, Q.; Murthy, K.S.; Baroni, T.E.; Becker, L.M. Nanoscale Constructs Inspired From Biological Systems, Proceeding of World Polymer Congress IUPAC MACRO 2000, Warsaw, vol. 3, p. 1053, July 2000.
11 Akashi, M.; Serizawa, T.; Baba, M.; Uchida, T.; Hiwatari, K-I. Core-Corona Polymeric Nanospheres: Synthesis and Application, Proceeding of World Polymer Congress IUPAC MACRO 2000, Warsaw, vol. 3, p. 1069, July 2000.
12 Saija, L.M.; Umi´nski, M. Synthesis and Characterisation of Core-shell Acid Functionalised Polyacrylate Dispersions. Surface Coat. Int. Part B: Coating Transactions 85, B2, 149-153, June 2002.
13 Umi´nski, M.; Saija, L.M. Fast Method of Total Acid Groups Determination in Functionalized Acrylic Dispersions. J. Dispersion Sci. Techn. 16 (6), 511-519 (1995).
14 Gupta, M.K. Estimation of the Glass Transition Temperature of Acrylic Copolymers. J. Coat. Technology, 67 (846), 53-58, July 1995.
Looking for a reprint of this article?
From high-res PDFs to custom plaques, order your copy today!