Off-Diagonal Effects of Alkanolamines in Waterborne Coatings
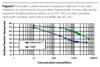
Alkanolamines are ideal pH-neutralizing agents in a number of ways, including compatibility with latex emulsions, solubility in latex emulsions, a sufficiently high pKa value to allow for efficient neutralization to pH values between 8 and 10, and intermediate vapor pressures that allow for evaporation at a controlled rate. The optimum alkanolamine for a given coating formulation will be the one with the best balance of physical properties, allowing for the maximum number of benefits and the minimum number of negative side effects (e.g., odor).
One of the easiest ways to evaluate and compare different alkanolamines as additives in waterborne coatings is through the concept of off-diagonal effects, and this paper will, for clarity, start with a generic description of the off-diagonal analysis of additive effects. The generic discussion will be followed by some physical data summaries useful in illustrating how off-diagonal effects can be predicted ab initio. Ultimately, we hope to convince the reader that Arkema's Advantex™ additive is a useful neutralizing alkanolamine alternative for waterborne coatings.

The Off-Diagonal Effect
Coating formulations are composed of multiple ingredients added for various reasons. In a typical waterborne coating, the following generic ingredients may be present:- Solvent: water;
- Antifreeze: propylene glycol;
- Coalescent: 2,2,4-trimethylpentyl-1,3-pentanediol monoisobutyrate;
- Resin: butylacrylate - vinyl acetate copolymer;
- Pigment: titanium dioxide, other inorganic and organic color pigments and various extenders;
- Surfactant: nonionic surfactant;
- Dispersant: polyacrylic acid;
- Rheology Modifier: hydroxyethyl cellulose;
- pH Adjuster: alkanolamine; and
- Biocide: isothiazolinone.
Each of these ingredients is used for a reason. The solvent provides a vehicle for mixing, as well as a carrier for the other ingredients. The antifreeze stabilizes the mixture, allowing freeze/thaw cycling. The coalescent improves the physical properties of the dried binder by permitting the formation of a continuous film. The resin, which becomes the actual film, also acts as a binder for the pigment and other non-volatile components of the paint. The pigment provides for color, opacity and reinforcement. The dispersant allows for proper pigment dispersion during the grind and for stabilization of pigment-binder interactions in the finished liquid paint. The rheology modifier adjusts the viscosity of the coating, allowing for the appropriate flow properties and smooth application. The alkanolamine is used to adjust the pH to an optimal point, and the biocide is used to extend the shelf stability of the product by guarding against biological attack. Each of these primary purposes can be thought of as the diagonal effects of the ingredients.
The 10 x 10 matrix shown in Table 1 can be used to represent the effects of the individual additives on the coating formulation. The primary (diagonal) effects of the additives are represented by checks along the diagonal. The off-diagonal effects of the additives are given as a +/- symbol in all of the off-diagonal positions. These +/- symbols represent the potential positive or negative contributions that each additive can have on all the properties of the coating for which it was not primarily added. For instance, certain readily biodegraded resin binders will have a negative impact on the biostability of the coating during storage (so-called ‘in-can' stability). Many organic solvents have a positive effect on the freeze stability and resin coalescence properties of a given coating formulation. It is only through maximum enhancement of the off-diagonal effects in a given coating that a truly optimal formula can be found.
The primary purpose of this paper is to show that while all alkanolamines exhibit off-diagonal effects, both positive and negative, only certain alkanolamines are exceptional with respect to their ability to improve latex paint through significant positive impacts on odor, corrosion properties, adhesion, biostability, pigment stability, coalescence and overall performance. What follows is a comparative summary of the physical and chemical properties of several alkanolamines used in latex paint, with an emphasis on how these properties impact paint performance.

Off-Diagonal Effects in Action
Vapor PressureThe vapor pressure of a material can have consequences in a liquid paint as well as in a dried coating. With respect to the alkanolamine, any unpleasant odor associated with this additive will be enhanced by its volatility, and the volatility of an alkanolamine is a direct function of its vapor pressure. During the drying stage of a waterborne coating, a controlled volatility can also promote better film formation. As is usually the case, a balance of properties is needed to optimize coating performance.
The vapor pressure of an alkanolamine can be described by a number of equations, including the Clausius-Clapeyron equation ln(P) = -AT +B, the Antoine equation ln(P) = -AT-C +B, and the Riedel equation ln(P) = -AT +B+Clog(T)+DTE wherein A,B,C,D and E are regarded as correlation constants, P = vapor pressure of the pure liquid, and T = absolute temperature. The constants can be determined empirically by best-fitting pressure-temperature data from vacuum distillations. The Clausius-Clapeyron equation is sufficient to provide a good estimate of vapor pressure behavior between 20 and 150 ºC. Correlation constants for five alkanolamines commonly used in waterborne coatings are given in Table 2. Using the Clausius-Clapeyron equation, one can equate the constant A with the enthalpy of vaporization divided by the universal gas constant R (8.31447 J/ºK-mole) and the constant B with the entropy of vaporization divided by the universal gas constant.
Triethanolamine has a very low vapor pressure and correspondingly low odor, but evaporation of triethanolamine from coatings is extremely slow. AMP and MEA have adequate volatility for evaporation, but these amines produce sufficient vapor pressure to create noticeable odor. The vapor pressure of Advantex™ additive is an excellent compromise that allows for evaporation while still producing a very low overall odor.

HLB
The HLB (hydrophile-lipophile balance) of a surfactant molecule is a measure of the balance of hydrophilic (water loving) and lipophilic (oil loving) properties of the molecule. Originally, HLB values were measured experimentally by determining which of a number of standard oil-water emulsion mixtures a given surfactant was capable of emulsifying. More recently, a number of useful approximate calculations of HLB values have been developed. One useful method involves taking the percentage of the molecule's overall MW that can be identified as hydrophilic and dividing it by 5.1 This method was applied to a number of alkanolamines commonly employed in waterborne coatings (MW of hydrophilic portion of the molecule taken as 60) along with a reference number for a pure alkane to yield the values shown in Table 3.The differences in calculated HLB values for different alkanolamines, as given here, are manifested in the real world as subtle differences in the surfactant-like behavior of the alkanolamines. Figure 1 shows a semi-log presentation of surface tension (dynes/cm, aqueous solution - air) versus concentration (mmol/liter) data collected at room temperature (22 ºC) and standard pressure (1 atm). The surface tensions were measured by the maximum bubble pressure method with automatic hydrostatic pressure correction on a SITA® T-60 unit. Advantex™ additive shows an apparent critical micelle concentration (CMC) of approximately 1M, while AMP, with a higher calculated HLB, shows an almost continuous variation in surface tension from a concentration of 10 mmol all the way up to 50% wt/wt. The subtle surfactant-like behavior of Advantex™ has real benefits with respect to pigment surface wetting, pigment dispersant activity, film forming, corrosion inhibition and emulsion stability.
One practical manifestation of HLB is in corrosion protection. Materials with lower HLB values tend to form better corrosion barriers on metal surfaces. To illustrate this, we ran the following experiment. Brass (2" x 2" x 0.032") panels were immersed in aqueous solutions of alkanolamine (0.3 M) and octanoic acid (0.2 M) and adjusted with H3PO4 and/or KOH to pH equal to 8.5 at room temperature. A 450-gram portion of leach solution was weighed carefully and transferred to a wide-mouth screw-cap glass bottle. The brass panels were washed with 10% Liqui-Nox® cleaner and buffed dry with a paper towel. The panels were immersed in the various solutions (all panels oriented at the same angle within the solution) and the cap placed tightly on the jar. A 5-gram sample of each solution was collected at regular intervals and analyzed by ICP/emission for metals content. In this graph, the 30-day concentrations are reported. The brass alloys were leaded (CA-360, 3% Pb) and unleaded (CA-260) alloy. HLB values: Advantex™ additive = 10 (Cu concentration = 9 ppm for both leaded and unleaded), AMP = 13 (Cu concentration = 74 ppm for leaded and 123 ppm for unleaded), MEA = 20 (Cu concentration = 134 ppm for leaded and 211 ppm for unleaded), DGA = 20 (Cu concentration = 168 ppm for leaded and 234 ppm for unleaded), and TEA = 20 (Cu concentration = 165 ppm for leaded and 171 ppm for unleaded). The passive corrosion rates for brass panels immersed in neutralized aqueous solutions, as described above, are plotted versus the HLB of the alkanolamine-neutralizing agent in Figure 2.
One might surmise that the lowest possible HLB would be ideal for optimization of corrosion inhibition, but HLB values much lower than 10 lead to insolubility in aqueous media. Thus, it can be seen that Advantex™ additive has balanced properties that allow for optimal performance (e.g., good corrosion inhibition and aqueous solubility) in waterborne coatings. The calculated HLB value of 10 is indicative of a molecule that provides excellent corrosion inhibition, emulsification assistance, pigment wetting, leveling assistance, etc., while still being high enough to allow for complete solubility in and compatibility with a waterborne coating formulation.

pKa
The pKa of an alkanolamine can be conveniently measured by a careful pH titration of highly purified alkanolamine with degassed standard acid solution. Based on the Henderson-Hasselbach equation: pKa = pH - log [R3N]/[R3NH+], the pH at 1/2 neutralization will be equal to the pKa. The pKas of a number of purified alkanolamines determined by pH titration of the free base with nitric acid are given in Table 4.The pKa of the alkanolamine used in a waterborne coating has an influence on the performance of the coating. Higher pKa alkanolamines allow for more efficient neutralization and higher working pH levels, while lower pKa alkanolamines have some utility as reserve alkalinity in open industrial systems. The pKa of Advantex™ is high enough to allow for very efficient neutralization coupled with some reserve alkalinity. Overall, Advantex™ has an excellent pKa for latex paints and waterborne coatings.
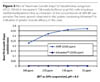
Biocide Synergy
The best way to prevent the microbial colonization of paint is to add biocide. Many classes of biocides are known, and many measurements of the Minimum Inhibitory Concentrations (MIC = the minimum concentration of the biocide necessary for control of a particular species of bacteria or fungi) of these different biocides have been made. However, toxicology, regulatory, economic and chemical stability issues complicate the otherwise straightforward strategy of adding a biocide to a commercial product. So whereas the use of biocides is nearly universal, the minimization of biocide concentrations is sought.How can the formulator deal with the contradictory goals of absolute biological control and minimization of biocide concentrations? One possible approach is the use of biocide synergy. Biocide synergy is a term used to describe the favorable effect of certain "non-biocidal" additives on the overall biological stability of a fluid.2,3 Biocide synergy is an off-diagonal effect that was first reported for alkanolamines by Ed Bennett in 1979,4 and that biocide synergy is one of the best-researched examples of an off-diagonal effect of an additive.5-7
One of Arkema's contributions to general work in this field has been the development, in collaboration with Microbe Inotech Laboratories (MIL, Saint Louis, MO), of standardized methods for the comparison of various alkanolamines as biocide synergists. We have used high-throughput optical absorbance measurements of bacterial growth in transparent broth media to compare various alkanolamines as synergists. In brief, the Arkema/MIL method involves dosing an appropriate pH-buffered transparent broth with various levels of biocide (e.g., triazine, BIT, CMIT/MIT) and alkanolamine. The prepared broths are dosed into the wells of a microtiter plate followed by standardized inoculation of all wells with a bacterial suspension of approximately 1010 CFU/ml (ª 108 CFU/ml after dilution). The prepared microtiter plate is placed in a temperature-controlled instrument chamber (typical inoculation T = 25 ºC), and absorbance measurements at 660 nm are made on all wells once every 15 minutes. The incubation is allowed to continue for two days.
After two or more days, the absorbance data is analyzed to determine how rapidly the bacteria grew in each of the wells. Typically, at least three replicates of each solution are used to ensure that statistical abnormalities did not influence the data. The maximum growth slope is calculated by computer analysis of 15 data point sequences from the beginning to the end of the experiment. The greatest slope found is taken as the maximum growth slope (MGS). The r2 value is checked to ensure that the slope value came from a reasonably linear portion of the data. The data can be fit to a partial log model, but such conversion is not necessary for quantification of relative maximum growth rates. Once an accurate MGS is determined, it can be plotted versus the concentration of various additives present in the different growth media. Through an examination of MGS values for different alkanolamines in otherwise identical solutions, an assessment of relative biocide synergy can be made. A typical graph of "Maximum Growth Slope" versus the concentration of the biocide benzoisothiazolinone (BIT) is given in Figure 3.
It is clear from the results in Figure 3 that Advantex™ is an excellent biocide synergist, and these results are completely typical of that seen in the literature and throughout the extensive testing we have completed. Alkanolamines like triethanolamine (TEA) exhibit next to no biocide synergy. Alkanolamines like DGA and MEA exhibit minimal biocide synergy. Alkanolamines like AMP exhibit moderate biocide synergy, while alkanolamines like Advantex™ exhibit the highest level of biocide synergy obtainable in a commercially available product. The impact of biocide synergy on the formulation strategy of a paint maker is less significant than it is in other areas like, for instance, metalworking fluids, but the effect can still be used to advantage in paints and waterborne coatings in general.
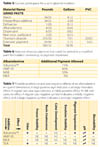
Pigment Dispersion
The physical properties of an additive result in unique behavior within a formulation. One of the more important side effects of paint additives is their positive or negative influence on pigment dispersion.Generally, there are optimal levels of dispersant and liquid required to give different types of pigment the best grind viscosity. These levels are based on the oil absorption of the pigments in the grind. The morphology of the pigments is also important, since the major role of dispersant is to act as a bridge between pigment particle and liquid carrier, displacing air from the pigment surface, and facilitating the breakdown of agglomerated pigment. Generally the dispersant present in a latex paint is either a sodium, potassium or ammonium salt of a polycarboxylic acid. As our work on HLB has shown, alkanolamines do have surface-active properties. Although they are relatively small molecules compared to typical commercial dispersants, it is obvious that they can help in the role of dispersant.
We have established "pigment dispersant synergy" with a number of experiments. In one simple experiment, several alkanolamines were tested to evaluate their dispersant characteristics. The alkanolamines studied included Advantex™, AMP-95, monoethanolamine (MEA) and methylaminoethanol (MAE). This grind paste from a typical semi-gloss paint was modeled with TiO2 and sodium potassium alumino-silicate (Minex 4) as the pigments, with their levels appropriate for a 40 NVV-24 PVC system. The base paint formulation employed 4 pounds/100 gallons of amine and 6 pounds/100 gallons of dispersant. The generic grind paste from that semi-gloss formulation is shown in Table 5.
The experiment involved replacement of all the dispersant with an equivalent amount (wt/wt, 6 pounds per 100 gallons) of alkanolamine followed by evaluation of the amount of additional pigment that could be successfully added to the grind without binding, excess strain and heating. The end point was approximate but quite obvious, as the addition of too much pigment, without added commercial dispersant, would lead to an extremely hard grind mass in the disperser with significant stain and excess heat generation. Table 6 presents the results of the experiment. Advantex™ gave the greatest assistance to the dispersion process, and one could infer from the results that Advantex™ is an exceptional "pigment dispersant synergist".
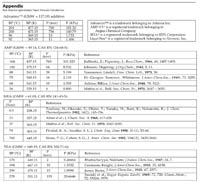
Conclusions
Just how much does the alkanolamine matter in a paint formulation? Let's reexamine a modified off-diagonal effect matrix with respect to the alkanolamine as given in Table 7. The alkanolamine may, in addition to changing the pH, have an impact on the odor, color development, freeze stability, coalescence (particularly in low-VOC formulations), emulsion stability, pigment suspension stability and biostability of the paint. Table 7 illustrates the average impact of several alkanolamines on the typical flat and semi-gloss formulations we examined.The impact of the alkanolamine on freeze stability is through modified emulsification, which is in turn a function of HLB - not necessarily the highest or lowest HLB, but the best balanced HLB for the system under consideration. The impact of the alkanolamine on resin coalescence is through vapor pressure - again, not necessarily the highest or lowest VP, but the best balanced VP for the system under consideration. The impact of the alkanolamine on pigment suspension stability and biostability is a complex function of molecular structure, but again the best effect is obtained through an ideal balance. In our testing, Advantex™ demonstrated an excellent balance of physical properties that make it effective as not only a pH-neutralizing agent but also as an optimal off-diagonal synergist.
For further information contact Michael D. Gernon, Arkema Inc., 610/878.6681, Michael.Gernon@ArkemaGroup.com; Conor M. Dowling, Arkema Inc., 215/419.7465, Conor.Dowling@ArkemaGroup.com; or Ben Carlozzo, DCA Coatings, 440/221.4265, Bcarlozzo@aol.com.
Looking for a reprint of this article?
From high-res PDFs to custom plaques, order your copy today!