Disc Pumps Keep Fluids Moving
Shear-sensitive latexes, abrasive Ti02 and other pigment slurries, and delicate emulsions all present pump-related problems that have posed major challenges to the coatings industry. Too often, the industry has settled for excessive pump operating costs and staggering product losses, as much as 50% in some cases. These losses would be considered unacceptable in any other industry.
Types of Pumps
There are three basic pump types. In a centrifugal pump, fluid enters at a high speed and is thrown against the casing by impellers. Another group uses positive displacement, meaning liquid is displaced with each revolution of the pumping elements. This is done by trapping liquid between a pumping element, commonly gears, lobes, rotary pistons, vanes, or screws and a stationary casing. Reciprocating pumps use a piston moving back and forth in a cylinder with valves that regulate the flow of liquid. All of these systems have one thing in common — they use a device to impel or push the fluid through the pump.Titanium dioxide is one of the most abrasive materials that can be put through a pump. It is moved in slurries that can average as much as 70% solids content by volume — literally liquid sand.
Centrifugal pumps are a regular choice for pumping Ti02. They are one of the most versatile, widely used and commonly understood industrial-application pumps. They come in all different sizes and configurations. For abrasive applications, they can be made of special resistant metallurgies and fitted with protective rubber linings. They need this help because, in reality, the centrifugal pump has the worst possible design for pumping abrasive product.
Abrasive Handling
Centrifugal pumps rely on narrow internal clearances called close tolerances to maintain the pressure in the pump needed for maximum efficiency. These tolerances wear away in abrasive service and the pumps steadily lose efficiency and eventually fail.In addition, all wetted areas in the pump are contact areas with impingement angles varying between 20° and 90°. The abrasion resistance of any pump design depends on the flow path of a solid as it passes through the pump. If the particle makes contact with a surface, the surface will wear. The amount of wear depends on the impingement angle (angle of attack) at contact and the relative velocity between the abrasive particles and the pump surfaces. It’s like a car wreck: At 90°, the impact is head on and, when you add a little speed, the destruction can be devastating. To solve this problem, centrifugal pump manufacturers have attempted to reduce impingement by lowering the impeller vane angles. After years of effort to extend the life of their pumps, most manufacturers still receive 60–80% of their total business from spare parts.
One way to use a centrifugal pump for an abrasive product is to oversize the pump and slow it down to reduce the velocity and impact of the solids. The larger pump will cost more to buy and generally operate with lower efficiency. Even after making these concessions, the slower pump could have more trouble with the solids. When fluid velocities fall, the solids can fall out of solution and clog the pump. In many cases, product would have to be diluted to get it through the pump. A more suitably sized, faster running pump will do the job more efficiently, but have a shorter, maintenance-intensive life.
Another choice could be the diaphragm pump. This pump can be used in applications including paint, chemicals and food. It generates a pulsating flow, produces less shear than a centrifugal pump, and is relatively inexpensive.
One problem with the pump in abrasive service is that it uses ball-and-cone or spring-loaded wafer valves to separate the intake from the discharge side. The valves can wear quickly and need constant attention. One end user called it nuisance maintenance, but it can also translate into expensive downtime. For another it was disastrous, as the particles worn from the ball valves contaminated their expensive product. For many other end users, their pumping difficulties seem so unsolvable that their attitude becomes “I know it’s going to break down so I want the least expensive pump for that job so I can afford to replace it.” However, the operating costs of a diaphragm pump can be 10 times that of a standard centrifugal, and replacing the pump four times a year or more in abrasive applications can be expensive.
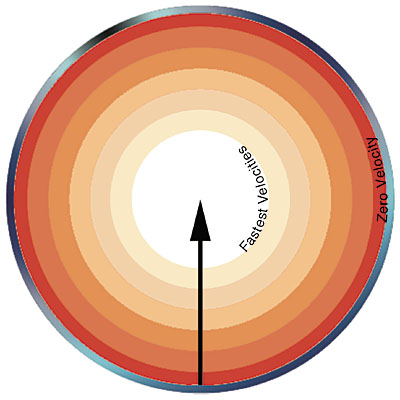
New Technology
The disc-operated pump is based on a non-contact, non-impingement technology. The operating principle behind the disc pump is called boundary layer-viscous drag. Its application to pumps is new, but it has been used widely in fluid engineering for over 100 years. A common example of this principle is the phenomenon of pressure drop or friction losses through a piping system.Examination of the cross-section of a pipe (see Figure 1) under laminar (non-turbulent) flow conditions shows that layers of liquid travel at different velocities. The stationary pipe exerts a “drag” force on the flowing liquid. The velocity of the layer adjacent to the pipe is zero where the drag force is the strongest. Successive layers flow increasingly faster with the highest velocities at the center point between the pipe walls.
This is the boundary-layer viscous drag principle behind the operation of the disc pump. The pump uses a series of parallel discs called the Discpac. The discs create “drag,” and an initial “boundary layer” of fluid adheres to their surfaces as flow enters the pump.
The boundary layer on the discs is stationary, like it is on the walls of the pipe. With rotating discs, the boundary layer is stationary relative to the discs. If we were standing on the discs, the boundary layer would have zero velocity. This means that it is at rest on the discs — thereby it is traveling at the same velocity. The streams at the center of the Discpac would be flowing with the fastest velocities. Relatively speaking, someone standing outside the pump will see that the “stationary” fluid layer on the disc surfaces has the highest velocity with fluid layers at the center between the Discpac having the lowest velocities.
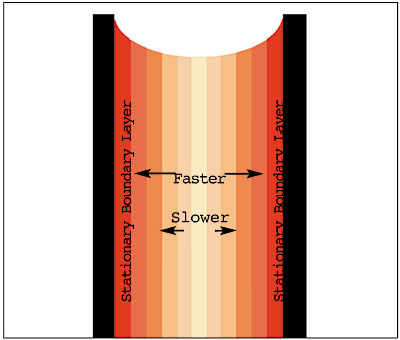
This system uses no pushing or impingement device to push product into the pump. The non-impingement pumping has some compelling advantages over traditional pump choices in handling difficult and shear-sensitive fluids.
Disc Design
The discs that are the pumping mechanism in the disc pump rotate in a plane perpendicular to the pump inlet so that the fluid flows are parallel. Except for the initial uptake of fluid, there is no contact or impingement between the pumpage and the discs. The impingement angle of the solids as they exit the Discpac is close to zero. Even if contact is made, it has little force and with a boundary layer fluid buffer, little or no damage results to pump or to product. Moreover, viscous drag/boundary layer operation forces solids that are heavier than the carrying fluid, to the point of lowest velocity at the center of the Discpac and away from the discs surfaces.Higher Solids
Typical Ti02 slurry from a bulk producer has a 70%-solids content. At this level, almost any pump will have clogging problems, but slurries containing up to 80% solids by volume can be pumped without clogging a disc pump. In-house testing at Discflo has shown that particles in slurries with a solid content of up to 40% pass through the pump without contact. At higher levels, there is still very little contact. This non-contact fluid handling can reduce pump wear by 80%, even in the most abrasive service conditions.Application Info
One of the world’s largest Ti02 producers pumps slurry that is more than 50%-solids by volume in a belt feed press application. A disc pump replaced a centrifugal pump with very good results. A recent inspection revealed that after more than a year of service, there is very little wear inside the pump. This was achieved without the linings that were required with the old centrifugals. This is not trivial. The rubber linings had to be replaced every 18 days. It is a labor- and time-intensive task and, because linings all look the same, it was common for an incorrect liner to be used. With an ill-fitting liner, the pump could go down in a single day. After taking care of start-up glitches, the disc pump has run trouble-free. During this time, three inspections have been done. The last showed a small amount of wear on the casing, but because the system requires a 3” suction line (inlet side) feeding a 6” discharge, the pump is almost certainly in a full state of cavitation.
Cavitation
Cavitation describes a vacuum-like condition in the pump. It can happen when liquid in the low-pressure area of the pump vaporizes. Vapor bubbles implode as they pass to regions of high pressure and can create a shock wave powerful enough to lift metal off the pump. The energy required to accelerate the liquid to high velocity and fill the void left by the bubbles causes a drop in capacity. Because the flow is laminar throughout the disc pump, there is minimal pressure loss and the risk of cavitation is reduced. Even when it does occur, the disc pump is relatively unaffected because it is protected by the boundary layer of fluid.
Efficiency Considerations
Operating conditions like these are common in belt press feed applications. In this case, the disc pump feeds the Ti02 slurry to a dryer. Previous pumps had required more carrying fluid to move the Ti02 to keep them from clogging. In fact, the suction side had to be hosed almost continuously to keep the centrifugals running. More fluid means more energy is spent in the drying process. The disc pump has wide internal clearances that have an affect on energy efficiency.The disc pump is engineered with wide internal tolerances because all of the disc surfaces are involved in pumping. Obviously, this design has advantages and disadvantages. Wider clearances reduce clogging and give the pump excellent handling capability in pumping abrasive and shear-sensitive materials. Another advantage is that there is no efficiency loss due to wear. The downside is that wider clearances reduce the pump’s efficiency in water-like applications.
In this application, the slurry had a viscosity of around 500 centepoise (cPs). A distinctive characteristic of the disc pump is that its pumping efficiency increases as viscosity rises. At viscosities greater than 350 cPs, the pump is actually more efficient than a comparatively sized centrifugal pump in a similar application.
Viscous drag is more efficient at transferring energy through layers of viscous fluid. The difference is illustrated in Figure 3, showing velocity profiles across the Discpac at viscosities of 1 cP and 5,000 cPs. At 5,000 cPs, the disc pump transfers about 25% more volume for the same horsepower and at 660 cPs, the disc pump will require half the horsepower of a similarly sized centrifugal pump. The disc pump can handle heavy viscosities up to several hundred thousand cPs.
Energy used by a pump, however, is only one consideration in the overall efficiency of a process system. In this particular case, the efficiency of the process was greatly increased because of a shorter drying time.
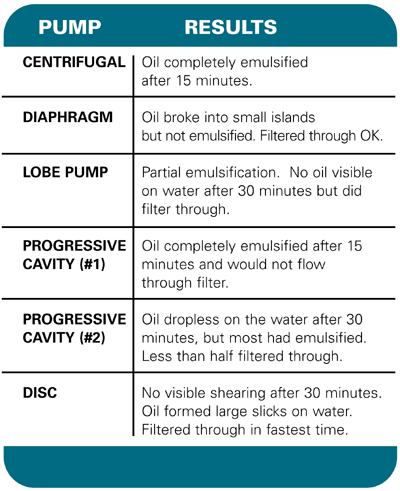
Viscosity
Like Ti02, calcium carbonate, and kaolin and calcined clays are common raw materials used in coatings. In slurry form, they can have a solids content as high as 50–70%. Like Ti02 slurries, abrasives have a high content that can make them hard to pump. They pose an additional difficulty as well; they are adversely affected by shear in the pump that is created by impingement and flow turbulence. Clay slurries thicken and can even set to a solid when subjected to the pressure and agitation of pumping.Historically, positive-displacement pumps are the foremost option for shear-sensitive fluids because they are low velocity and low shear. An old technology, a progressing cavity pump is a positive-displacement pump using in its basic form a single spiral-shaped rotor that turns eccentrically in a double-threaded stator. As the rotor turns, a series of sealed cavities progresses from inlet to discharge. This produces a continuous pulsating flow along a limited path through the pump. A compression fit that acts much like an o-ring sealing between stator and rotor makes the pump relatively efficient. However, this efficiency is gained at the expense of the ability to handle solids and abrasion. As with centrifugals, stators made of elastomer materials can be used to help the pump withstand abrasion, but when you have solids passing through a pump with extremely close tolerances, excessive wear and maintenance are a constant and unhappy fact of life.
Many clay products are so shear-sensitive that even in a progressing cavity pump the constant friction between the rotor and stator in the pump can create enough shear to raise the viscosity of the slurry high enough that the rotor of the pump will snap.
Users and manufacturers of clay-pigment slurries experience pump failures regularly. The track record for progressing cavity pumps was an unimpressive and expensive few weeks to a month for one producer who makes a 72.5%-solids calcium carbonate slurry used in a coating for fine paper. Crystals in the coating are specially engineered and are, says the distributor, “like pumping needles.” This combination of abrasion and shear was disastrous. After just a one-week demo trial with the disc pump, the company was completely convinced and purchased three more. Broken shafts became a thing of the past.
A company that produces the fine paper also resorted to disc pumps after several unsuccessful pump installations for unloading the specialty coating. The product is delivered to this customer by truck in slurry form and is pumped into holding tanks. Now when the truck pulls up, a disc pump is online and, because it is very efficient with viscous fluid, it empties the tank in 20 minutes — about half the time it took before.
Both of these pump installations occurred over a year ago and both have been maintenance-free. This is typical performance for disc pumps in an abrasive application with higher viscosity levels.
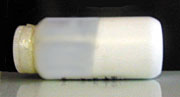
Gentle Fluid Handling
The same operating characteristics of the disc pump that allow it to pump viscous fluids and harsh abrasives make it one of the gentlest handling pumps available. In pumping emulsions, independent testing has shown that the disc pump has little impact on the product. In one test, a mixture of oil, water and detergent was circulated for 30 minutes through five conventional-style pumps and a disc pump. The amount of shearing, emulsification and disintegration for each pump was investigated, and then a sample was filtered through a membrane. The results are shown in the table. After similar testing, a large international producer of coatings for industrial and decorative uses made the disc pump a company-wide standard in the manufacture of a proprietary emulsion. The pumping is so smooth that shear in the pump is less than it is in the discharge piping.
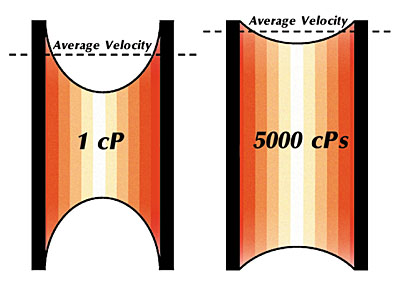
The Big Impact of Non-Impact Pumping
One of the pump’s big success stories is in working with latex. There is an inherent difficulty in working with latex products. Any kind of impact — whether pump on fluid or fluid on fluid — negatively affects the quality of the product. Many producers don’t understand why, no matter what kind of fluid handling system is designed, their products still suffer.The main culprit is shear, caused from impingement and by turbulence in the fluid flow. A stable, consistent viscosity is fundamental to the quality of most inks and coatings. Latex products are especially vulnerable. Friction in the pump generates heat that congeals it. In congealed form, it coats the interior surfaces of the pump and fills the stuffing box, destroying the seal. Clumps of latex particles clog the pump and the piping, and plug strainers and filters down-line. Not only that, congealed latex is product that cannot be sold.
Because there is no impingement and the flow is laminar, the disc pump produces no heat. One end user had tried several kinds of pumps but all produced shear that created latex solids in high concentrations. They wreaked havoc on the pump and then moved downstream and clogged the filters. The company reported, “In less than a month of operation, the Discflo pump has increased productivity approximately 12% by eliminating the latex solids produced by shear.” The increased productivity figure does not include filters or downtime.
Protection of latex emulsions is the top concern for producers of this expensive commodity, but pump performance is also important. The Discflo pump does not suffer in latex pumping the way conventional-style pumps do. Discflo has pumps in latex applications that have been in continuous service for more than 10 years.
Disc pumps are widely used for shear-sensitive dispersions and emulsions and are employed in the manufacture and processing of components in today’s industrial and decorative coatings — resins, polymers, monomers, acetates, silicates, alkyds, all kinds of pigments, and a host of finished products. In applications where abrasion, shear-sensitivity, high-solids content, viscosity and other difficult fluid conditions exist, disc pumps can provide a real solution and introduce new economy for processes where profitability has been hampered by high maintenance costs or unacceptable product degradation. A majority of the industry’s biggest and best producers have already found that out and depend on the handling characteristics of disc technology for many of their most challenging pumping processes.
Acknowledgments
The author would like to thank the following people for their technical contributions: John Pacello, Discflo VP Engineering; Mike Szczepanski, W.T. Bryan Inc., Cincinnati, OH; Jerry Cotreau, Diversified Pumps, Wakefield, MA; and Tero Lehtonen, Puwimex, Finland. cFor more information on disc pumps, contact Discflo, 1817 John Towers Ave., El Cajon, CA 92020; phone 619/596.3181; fax 619/449.1990; visit www.discflo.com; e-mail disflo@discflo.com.
Looking for a reprint of this article?
From high-res PDFs to custom plaques, order your copy today!