Reaching Strict VOC Requirements with Outstanding Durability
The focus of this paper is on the advancements of “Addition Curing” technologies pertaining to the reduction of VOCs, and improving exterior durability, particularly as it relates to gloss, color and corrosion resistance with minimal impact on cost.
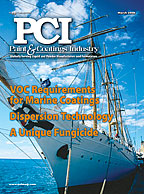
The world is shrinking! In 1903, Orville and Wilbur Wright flew the first self-propelled aircraft. That same year, H. Nelson Jackson and Sewall Crocker were the first to drive across the United States via automobile, which took them 57 days! During this time frame most citizens did not travel outside of their county, much less to other states and countries. However, a short 44 years later, Orville and Nelson both lived to hear about Chuck Yeager flying at the speed of sound. Now, we are able to cross oceans, deserts, forests and reach any destination on earth within 24 hours or so. This poses the question: Who are our neighbors? How can manufacturers provide workable solutions according to regional needs? What might be the impact regarding such issues as environmental sustainability? Responsible companies ask themselves this question daily, thinking globally about the economic and environmental impact they have on countries beyond their continent. The focus on preserving the quality of life – the “GREEN REVOLUTION”1 – has forced companies to develop marketing strategies focused on decreasing VOCs and toxicity in coating systems,2 and, in addition, meet customer needs.
“Reaching strict VOC limitations with outstanding durability for industrial maintenance and marine coatings” are some of the largest challenges faced today by coating formulators.3 Due to increasing maintenance costs, customers in these market segments are looking for longer-lasting, more durable coatings. Some of the most important criteria remain corrosion resistance, UV resistance and lower VOCs. The new polysiloxane hybrid technologies are meeting these challenges.
The focus of this paper is on the advancements of “Addition Curing” technologies pertaining to the reduction of VOCs, and improving exterior durability, particularly as it relates to gloss, color and corrosion resistance with minimal impact on cost.
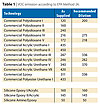
These organic polymers have different methods of degradation, which are related to the bond strength and stability of the hydrocarbon backbone. Typical bond strengths are approximately 85 kcal/mole for the C-C bond and approximately 87 kcal/mole for the C-O bond. However, the replacement of carbon with silicon (Si-O-Si) increases the bond strength to approximately 110 kcal/mole.4 Therefore, the incorporation of siloxane-based materials will increase the durability of organic polymer-based technologies. In addition the inherent tendency of siloxanes to have low viscosity enables coating manufacturers to formulate coatings requiring less solvent and, in some cases, siloxanes can be used as reactive diluents.
The first coating applied onto metal substrates is designed to protect the surface from corrosion and is typically an inorganic (or organic) zinc-rich primer. These technologies rely on cathodic corrosion protection from the zinc and are generally coated with an epoxy sealer used to form a barrier. Unfortunately, these coatings are not UV resistant and require topcoating with an organic UV-resistant coating.5 The types of topcoats used have typically been silicone alkyds, urethanes and condensation-curable polysiloxanes. These systems generally contain solvents, which are required for application; or, in the case of the condensation-curable siloxane technologies, will typically give off VOCs during curing (hydrolysis). In addition, urethanes used as topcoats are considered to be potentially toxic due to the possibility of minute amounts of free isocyanate, and they are generally formulated with a significant amount of solvent (see Table 1). Several applications have moved away from using urethanes to a greener technology such as those based on siloxanes.
The polysiloxanes have been used to form coatings based on an interpenetrating network (IPN). These polysiloxanes utilize the alkoxy functionality on the silicone to homopolymerize and crosslink with silanes, which require a moisture curing mechanism.
These compositions also require a high degree of alkoxy functionality on the silanes and/or polysiloxane components, which, after curing, will have a VOC (Table 1) due to the generation of alcohol as a byproduct (Figure 1). In addition, these coatings will tend to wrinkle and/or crack if a good through cure is not achieved due to the continuation of hydrolysis after exposure to moisture and heat (sunlight). The byproducts are normally methanol; however in some cases ethanol, propanol or butanol can be present. The silanes are generally organofunctional and will react with organic resins as a secondary mechanism for curing.
Improvements in polysiloxane technology utilize the durability of the siloxane network without relying on alkoxy functionality as the main crosslinking mechanism. The newer siloxane technologies have incorporated organic functional groups such as epoxy or amines onto the backbone of the polymer, utilizing addition curing mechanisms for crosslinking.6 Reducing the amount of condensation and increasing the addition reaction mechanism reduces the level of cracking that occurs after exposure to sunlight and moisture.

The alkoxy functional group on silanes or silicone polymers will react with water under ambient conditions to form silanol groups and generate alcohol as a byproduct (Figure 1). The silanol-functional siloxanes will homopolymerize to form oligomers and/or ultimately high-molecular-weight silicone resins. Generally moisture is provided by the atmosphere and, therefore, in thick films there can be a problem with through cure. The modification of this type of system with organic polymers decreases the tendency for this to occur, however, it is not completely eliminated.
If the silane or silicone polymer is silanol functional then the byproduct of the reaction will be water (Figure 1). Generally the silanol functional polymers are either a flake or in a high-pH or low-pH environment if in solution. These resins are excellent for use as blending resins or as modifiers for other organic resins.
In each case the alkoxy- or silanol-functional siloxane can react with hydroxyl-functional organic polymers to form silicone-modified resins (i.e., silicone-modified alkyd or silicone-modified polyester), as seen in Figure 2. This reaction is driven by heat, and in both cases is a reversible reaction. The silicone-oxygen-carbon linkage is susceptible to hydrolysis and, therefore, the addition of moisture and heat will hydrolyze the polymer to form the reactants. If this occurs on exposure, the result would be oxidized organic polymer (oxidation accruing from UV degradation) and siloxane, which would be more hydrophilic.
The incorporation of an addition curing group on the silicone polymer will eliminate the reversible reaction and still allow the chemist the ability to form an ambient curing technology. The attachment of a functional group via a hydrolytically stable Si-C linkage and not the hydrolysis susceptible Si-O-C linkage will react with standard addition-curing crosslinkers to form hydrolytically stable systems.
The modification of the polysiloxane or silicone resin with epoxy-functional groups enables formulators to use typical crosslinkers, which react with epoxy groups, such as acid functional acrylic, phosphates, amines, etc., to form a film (Figure 3). The reaction of the epoxy with acrylic to form the acrylic ester is supported by the use of C13-NMR. There is a decrease of the epoxide peak and the acrylic acid peak with the formation of an epoxy-C-O-C(O)-acrylic peak.
Conversely the modification of silicone with amine groups enables formulators to use raw materials, which will react with amine-functional polymers, such as aliphatic epoxy resins, GMA acrylic, etc. (Figure 4).6
The intrinsically low viscosity of the base polysiloxane reduces the overall viscosity of the modified polymer, which makes it possible to formulate products with improved exterior performance and lower VOC than classical technologies (Table 1).
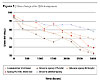
The use of QUV-A as a tool for elimination of good and bad candidates of the same chemistry was found to be acceptable; however, when comparing different types of chemistries, caution would have to be used. The relative position of the silicone epoxy I and silicone epoxy II/acrylic formulations were the same after exposure to more than 3000 hours in QUV-A (Figures 5 and 6) and after 4 years exposure in South Florida (Figures 7 and 8; Photos 1 and 2). A rough estimate of the relationship of hours in QUV-A and years at South Florida exposed at 45° south is that 750 hours corresponds to approximately 1 year.
On the other hand, the comparison of the commercial acrylic urethane performed much better in the QUV-A for gloss retention verses natural exposure (Figures 5 and 7; Photos 3 and 4).
It is suspected that this formulation used UV absorbers and light stabilizers, which cause the film to appear essentially unchanged from 300 hours to approximately 1200 hours in QUV-A.
This occurs during years 1-2 and makes it difficult to find a correlation between QUV and South Florida exposure.
The first year would be ~500 hours and the second year would be after ~2000 hours in QUV-A.
The non-iso acrylic/epoxy system also uses large amounts of UV absorbers and stabilizers, which performed much better in QUV than in natural sunlight (Figures 9 and 10). The non-iso system had excellent performance in QUV but dropped significantly after 1-2 years exposure in South Florida.
The other variables not measured by QUV are the effect of dirt pickup, abrasion due to blowing particulate, colder climates and wind driven rain. The exposure panels were prepared from the same paints to minimize variability when comparing QUV verses Florida exposures. The silicone epoxy I and silicone epoxy II performed better than urethane and non-iso systems.
The silicone epoxy (Figure 11) used in the silicone epoxy I/acrylic formulation is used as the crosslinker for the acid-functional acrylic, which is approximately 16% by weight based on total formulation (Table 2). The silicone epoxy II had a lower functionality and, therefore, the coating formulation (silicone epoxy II/acrylic) contained much higher silicone content (25%). The commercial urethane had a gloss of 26 versus the silicone epoxy I formulation having 38-39 and the silicone II having 66 measured at a 60° angle.
The silicone epoxy II had less than 53% change in color (delta E 1.28) compared to the urethane (delta E of 2.42) after 4 years. Increasing the silicone content of the addition curing technology will further increase the performance as it relates to gloss and color retention. If gloss and color were the only predictors for exterior durability, the silicone epoxy II system would last 2.5 times longer than the urethane technology and the silicone epoxy I would last ~30% longer.
One set of panels was sent out after applying direct to steel (non-treated cold rolled steel) to evaluate corrosion protection. There is very minimal corrosion detected on the silicone epoxy I panels after 3 years (Photo 5); however the silicone epoxy II panels had approximately 15-18% of the panel covered with corrosion (blisters) (Photo 6).
This propagated from the bottom edge of the panel upward. The same acrylic with a different epoxy crosslinker (non-iso system) had red rust showing on the panels after 2 years exposure (Photo 7) and the polyurethane had approximately 13-16% coverage with corrosion (Photo 8). However the evaluation of an older commercially prepared alkoxy technology had the highest degree of rusting which resulted in a majority of the coating delaminating (not pictured).
The older technology relies on the alkoxy reaction, which if moisture penetrates the system, the under layer can react and continue to delaminate. The replacement of the alkoxy system with the addition curing technology minimizes the level of cracking and has a much higher degree of elongation (Table 3).
Exposure of the addition-curing technology in cold climates experiencing freeze thaw conditions amplifies problems associated with brittle technologies. The difference in gloss retention and color stability of all the systems over CRS was slightly lower (Figure 9 and 10) than the coatings over aluminum.
The evaluation of amino functional silicone (Figure 12) with an aliphatic epoxy crosslinker was introduced late in the exposure study and, therefore, to simplify this paper the data was added to the graphs of the chosen exposures. The silicone amine/epoxy system had excellent UV protection with a high gloss retention (see Figures 5-8); however the corrosion protection is less than that of the silicone epoxy/acrylic systems, which may be related to formulation technique. The silicone amine/epoxy system contains approximately 33% silicone based on total coating (Table 2), which may contribute to the higher gloss retention.
Market analysis of commercially available acrylic polyol polyurethanes concluded that the average VOC, as supplied, is 373 g/L (EPA Method 24). The VOC after using recommended dilution directions had an average of 389 g/L. The low-viscosity siloxane-based materials enable formulators to prepare products with VOCs ranging from 50 to 160 g/L and the ability to use VOC-exempt solvents if necessary for dilution.
The non-isocyanate-based systems used the same acid-functional acrylic as part A. This system had UV stabilizers added to increase exterior durability.
Panels were prepared by using a wire-wound draw down bar and in some cases a bird blade as the applicator. The substrate used was non-treated cold rolled steel and non-treated aluminum from Q-panel.
Panels were exposed 45°south in South Florida (Q-Panel). One panel was sent back after exposure each year for evaluation. The edges and backs were taped on the steel panels to prevent backside corrosion. Panels were evaluated after washing half with a wet sponge.
The accelerated weathering test was completed by using QUV-A bulbs with a 4-hour UV exposure cycle with a 15-minute spray and a 4-hour condensation cycle.
There were 48 papers presented at NACE 2007 and seven papers presented at PACE-2007 focusing on development/utilizing green technologies. The International Coatings Exposition in October, 2007, which adopted the “Clean–Lean–Green: Innovative Solutions for the Global Coatings Community,” as its theme, speaks of how companies and, more specifically those in product development, are proactively looking at ways of protecting our environment and the environment of our neighbors. Reducing VOCs, using less-harmful raw materials and increasing coating durability are the beginning steps to preserve a cleaner more desirable place for our children.
Special thanks to Joel D. Kennard for his support in evaluation of analytical results.
Special thanks to the following companies for their support with raw materials and formulation guidance:
Cook Composite and Polymers, www.ccponline.com
For further information, visit www.wacker.com.
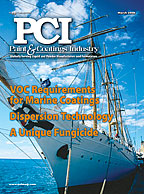
The world is shrinking! In 1903, Orville and Wilbur Wright flew the first self-propelled aircraft. That same year, H. Nelson Jackson and Sewall Crocker were the first to drive across the United States via automobile, which took them 57 days! During this time frame most citizens did not travel outside of their county, much less to other states and countries. However, a short 44 years later, Orville and Nelson both lived to hear about Chuck Yeager flying at the speed of sound. Now, we are able to cross oceans, deserts, forests and reach any destination on earth within 24 hours or so. This poses the question: Who are our neighbors? How can manufacturers provide workable solutions according to regional needs? What might be the impact regarding such issues as environmental sustainability? Responsible companies ask themselves this question daily, thinking globally about the economic and environmental impact they have on countries beyond their continent. The focus on preserving the quality of life – the “GREEN REVOLUTION”1 – has forced companies to develop marketing strategies focused on decreasing VOCs and toxicity in coating systems,2 and, in addition, meet customer needs.
“Reaching strict VOC limitations with outstanding durability for industrial maintenance and marine coatings” are some of the largest challenges faced today by coating formulators.3 Due to increasing maintenance costs, customers in these market segments are looking for longer-lasting, more durable coatings. Some of the most important criteria remain corrosion resistance, UV resistance and lower VOCs. The new polysiloxane hybrid technologies are meeting these challenges.
The focus of this paper is on the advancements of “Addition Curing” technologies pertaining to the reduction of VOCs, and improving exterior durability, particularly as it relates to gloss, color and corrosion resistance with minimal impact on cost.
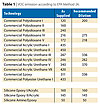
VOC emission according to EPA Method 24.
Background
Some of the first coatings or varnishes were derived from naturally occurring resins such as rosin (sap from pine trees) and oils (coconut, sunflower, etc.), which required a large amount of solvent for application.3 There was less of a concern regarding the VOC of these older technologies and more of a concern for cost and performance. The modification of these technologies and the development of synthetic products have created several new classes of resins with several different types of functionality. Alkyd, epoxy, polyester and acrylic resins are a few of the basic types of synthetic organic resins, and these could be subcategorized by type of functionality such as hydroxyl, carboxyl, amino, mercapto, isocyanato and silyl, etc. These resins are generally supplied as 50 - 80% solids, and have to be diluted during application to form a uniform film.These organic polymers have different methods of degradation, which are related to the bond strength and stability of the hydrocarbon backbone. Typical bond strengths are approximately 85 kcal/mole for the C-C bond and approximately 87 kcal/mole for the C-O bond. However, the replacement of carbon with silicon (Si-O-Si) increases the bond strength to approximately 110 kcal/mole.4 Therefore, the incorporation of siloxane-based materials will increase the durability of organic polymer-based technologies. In addition the inherent tendency of siloxanes to have low viscosity enables coating manufacturers to formulate coatings requiring less solvent and, in some cases, siloxanes can be used as reactive diluents.
The first coating applied onto metal substrates is designed to protect the surface from corrosion and is typically an inorganic (or organic) zinc-rich primer. These technologies rely on cathodic corrosion protection from the zinc and are generally coated with an epoxy sealer used to form a barrier. Unfortunately, these coatings are not UV resistant and require topcoating with an organic UV-resistant coating.5 The types of topcoats used have typically been silicone alkyds, urethanes and condensation-curable polysiloxanes. These systems generally contain solvents, which are required for application; or, in the case of the condensation-curable siloxane technologies, will typically give off VOCs during curing (hydrolysis). In addition, urethanes used as topcoats are considered to be potentially toxic due to the possibility of minute amounts of free isocyanate, and they are generally formulated with a significant amount of solvent (see Table 1). Several applications have moved away from using urethanes to a greener technology such as those based on siloxanes.
The polysiloxanes have been used to form coatings based on an interpenetrating network (IPN). These polysiloxanes utilize the alkoxy functionality on the silicone to homopolymerize and crosslink with silanes, which require a moisture curing mechanism.
These compositions also require a high degree of alkoxy functionality on the silanes and/or polysiloxane components, which, after curing, will have a VOC (Table 1) due to the generation of alcohol as a byproduct (Figure 1). In addition, these coatings will tend to wrinkle and/or crack if a good through cure is not achieved due to the continuation of hydrolysis after exposure to moisture and heat (sunlight). The byproducts are normally methanol; however in some cases ethanol, propanol or butanol can be present. The silanes are generally organofunctional and will react with organic resins as a secondary mechanism for curing.
Improvements in polysiloxane technology utilize the durability of the siloxane network without relying on alkoxy functionality as the main crosslinking mechanism. The newer siloxane technologies have incorporated organic functional groups such as epoxy or amines onto the backbone of the polymer, utilizing addition curing mechanisms for crosslinking.6 Reducing the amount of condensation and increasing the addition reaction mechanism reduces the level of cracking that occurs after exposure to sunlight and moisture.

Alkoxy functional group reaction with water.
Film Formation
Understanding silicone polymer film formation is important in order to build the foundation for understanding how to use these types of polymers and more importantly to highlight their potential weaknesses. This next section focuses on the crosslinking mechanisms (hydrolysis, homopolymerization, copolymerization and addition reactions) of silicone-containing moieties and how they are used to form coatings.The alkoxy functional group on silanes or silicone polymers will react with water under ambient conditions to form silanol groups and generate alcohol as a byproduct (Figure 1). The silanol-functional siloxanes will homopolymerize to form oligomers and/or ultimately high-molecular-weight silicone resins. Generally moisture is provided by the atmosphere and, therefore, in thick films there can be a problem with through cure. The modification of this type of system with organic polymers decreases the tendency for this to occur, however, it is not completely eliminated.
If the silane or silicone polymer is silanol functional then the byproduct of the reaction will be water (Figure 1). Generally the silanol functional polymers are either a flake or in a high-pH or low-pH environment if in solution. These resins are excellent for use as blending resins or as modifiers for other organic resins.
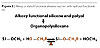
Alkoxy or silanol-functional siloxane reaction with hydroxyl functionality.
The incorporation of an addition curing group on the silicone polymer will eliminate the reversible reaction and still allow the chemist the ability to form an ambient curing technology. The attachment of a functional group via a hydrolytically stable Si-C linkage and not the hydrolysis susceptible Si-O-C linkage will react with standard addition-curing crosslinkers to form hydrolytically stable systems.

Organosiloxane/nucleophile reaction.

Organosiloxane/electrophile reaction.
The intrinsically low viscosity of the base polysiloxane reduces the overall viscosity of the modified polymer, which makes it possible to formulate products with improved exterior performance and lower VOC than classical technologies (Table 1).
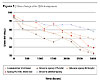
Gloss change after QUV-A exposure.
Results
Formulations using addition curing technologies have been prepared and coatings evaluated by QUV-A, south Florida, and Adrian, MI. The former was used as a tool to develop accelerated exposure data for the coating systems until exterior exposure data could be accumulated. In each case commercially available acrylic polyurethanes using aliphatic isocyanates were used as controls and other non-isocyanate-containing technologies. The use of commercially available materials as controls eliminates the lack of formulation experience as a variable in setting the industry control. The new siloxane hybrid technologies and the non-isocyanate-containing technologies were prepared from guide formulations.
Color change after QUV-A exposure.
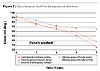
Gloss change on South Florida exposure on Aluminum.
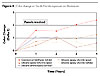
Color change on South Florida exposure on Aluminum.



Gloss change on South Florida exposure on steel.
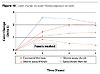
Color change on South Florida exposure on steel.
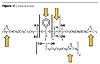
Silicone epoxy.

Silicone epoxy formulations.
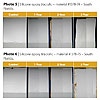

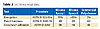
ASTM test result data.

Amino functional silicone.
The evaluation of amino functional silicone (Figure 12) with an aliphatic epoxy crosslinker was introduced late in the exposure study and, therefore, to simplify this paper the data was added to the graphs of the chosen exposures. The silicone amine/epoxy system had excellent UV protection with a high gloss retention (see Figures 5-8); however the corrosion protection is less than that of the silicone epoxy/acrylic systems, which may be related to formulation technique. The silicone amine/epoxy system contains approximately 33% silicone based on total coating (Table 2), which may contribute to the higher gloss retention.
Market analysis of commercially available acrylic polyol polyurethanes concluded that the average VOC, as supplied, is 373 g/L (EPA Method 24). The VOC after using recommended dilution directions had an average of 389 g/L. The low-viscosity siloxane-based materials enable formulators to prepare products with VOCs ranging from 50 to 160 g/L and the ability to use VOC-exempt solvents if necessary for dilution.
Experimentation
The silicone epoxy formulations with acid-functional acrylic were prepared by grinding the pigment in the acrylic resin. Additives were used for defoaming and flow. No time was devoted to finding the ideal additive package or solvent compatibility, which resulted in some surface defects that can be easily eliminated. The viscosity of the grind base was adjusted by adding low-viscosity siloxane. The silicone epoxy was used as the crosslinker and mixed as the “B” component. There were no UV absorbers or stabilizers added to the silicone-based systems. The formulation was designed to evaluate the stability of the silicone epoxy and silicone amine crosslinkers (Table 2).The non-isocyanate-based systems used the same acid-functional acrylic as part A. This system had UV stabilizers added to increase exterior durability.
Panels were prepared by using a wire-wound draw down bar and in some cases a bird blade as the applicator. The substrate used was non-treated cold rolled steel and non-treated aluminum from Q-panel.
Panels were exposed 45°south in South Florida (Q-Panel). One panel was sent back after exposure each year for evaluation. The edges and backs were taped on the steel panels to prevent backside corrosion. Panels were evaluated after washing half with a wet sponge.
The accelerated weathering test was completed by using QUV-A bulbs with a 4-hour UV exposure cycle with a 15-minute spray and a 4-hour condensation cycle.
Conclusion
The use of a coating prepared from silicone epoxy I and an acid-functional acrylic had ~30% increase in gloss retention and better corrosion protection than commercially available polyurethanes and non-iso systems. The lower-functional silicone epoxy system (silicone epoxy II) has 2.5 times better gloss retention after 4 years exposure over non-treated aluminum and after 3 years exposure over non-treated cold rolled steel. However the corrosion protection matched the urethane. The silicone amine/epoxy system had better gloss retention than urethane in QUV and South Florida with similar corrosion resistance. If corrosion and gloss/color retention were the only measure for the durability of a coating then the silicone acrylic formulations should last 30-250% longer than urethane technologies. This in itself would decrease VOC emissions that are associated with application and cleanup; in addition the new addition curing technologies can be formulated with at least 50% less VOC than commercially available urethanes.There were 48 papers presented at NACE 2007 and seven papers presented at PACE-2007 focusing on development/utilizing green technologies. The International Coatings Exposition in October, 2007, which adopted the “Clean–Lean–Green: Innovative Solutions for the Global Coatings Community,” as its theme, speaks of how companies and, more specifically those in product development, are proactively looking at ways of protecting our environment and the environment of our neighbors. Reducing VOCs, using less-harmful raw materials and increasing coating durability are the beginning steps to preserve a cleaner more desirable place for our children.
Special thanks to Joel D. Kennard for his support in evaluation of analytical results.
Special thanks to the following companies for their support with raw materials and formulation guidance:
Cook Composite and Polymers, www.ccponline.com
Rohm and Haas, www.rohmhaas.com
For further information, visit www.wacker.com.
Looking for a reprint of this article?
From high-res PDFs to custom plaques, order your copy today!