Waterborne Polyurethane Dispersions with Enhanced Hydrophobicity
for Direct to Metal Applications

Polyurethane dispersions (PUDs) are employed in many high-performance waterborne coatings due to their excellent properties and weatherability. Considerable attempts have been made to improve the corrosion resistance and mechanical properties through polymer structure and composition engineering to provide a robust system for direct-to-metal (DTM) applications. In this work, hydrophobic modification and structural design, including silane and Bisphenol A alkoxylate incorporation of waterborne PUDs resulted in excellent anti-corrosion performance and water resistance compared to conventional PUDs. An adhesion study on cold-rolled steel was also conducted and compared the effect of each modification by peel strength test.
Introduction
PUDs, like many other waterborne polymers, have gained increasing interest in recent years, and are considered as an alternative solution for replacing solvent-based coatings due to their outstanding performance, versatility and environment-friendly features. 1 More and more stringent environmental regulations on the emissions control of volatile organic compounds (VOCs) seems to be a driving force for developing these high-performance waterborne polymers. PUDs are primarily used in wood coatings, textile and fabric coatings, leather coatings, glass fiber sizing, and automotive interior coatings.
Typically, PUDs consist of polyurethane particles dispersed in water as a continuous phase; the general hydrophobic PU portion is made dispersible by incorporating an internal ionic stabilization center (or hydrophilic groups) into its backbone. 2 As a result, despite the high performance of PUD coatings from a property perspective, they are not directly used in protecting metal substrates compared to other waterborne resins, as the hydrophilic segments embedded in the polymer backbone are considerably susceptible to water and lead to corrosion propagation. Therefore, there is still room for improvement in waterborne PUDs regarding their barrier properties and electrochemical impedance to equal the performance of solvent-based anti-corrosion coatings.
Multiple coats, including primer and topcoat, are usually applied in aforesaid anti-corrosive coatings systems to achieve the highest protection. In contrast, a DTM monocoat may be applied, which is required to meet a wider performance spectrum, including adhesion, corrosion resistance, chemical resistance, UV resistance, abrasion resistance and hardness, and also becomes attractive due to the economic advantage. Usually, 1K waterborne DTM coatings do not perform as well as multi-layered systems, and are suitable for light-duty applications. However, PUD resins can be one of the top choices if we address the issue by reducing their hydrophilicity. 3
Several advanced technologies have been employed in polyurethane coatings to improve their corrosion resistance, e.g., grafting graphene oxides to polymers, 4 using a hybrid of organic and inorganic components such as silica or clay nanocomposites to form a hydrophobic matrix, 5 or using cerium salts as a corrosion inhibitor. 6 These approaches are designed to improve the barrier properties that prevent oxygen and water/moisture penetration, or to increase the electrochemical impedance. In addition, another common approach is to use organosilane coupling agents for metal protection, which help increase the surface hydrophobicity and form a dense barrier layer to prevent moisture and oxygen penetrating, and improve the adhesion to substrate. 7,8 Enhanced corrosion resistance is also demonstrated in modification of waterborne polyurethanes with self-crosslinking acrylic hybrid systems. 9 The methods described above have been proven to have a significant effect on enhancing corrosion resistance.
Based on the corrosion mechanism, there are several key factors that initiate and accelerate the process: a) presence of water and oxygen, b) formation of anode and cathode, and c) electrolytes. Electrochemical reactions will take place when water and ions reach the interface between polymer and metal. Thus, to prevent corrosion, eliminating any of these factors would be an effective solution. However, in real-world practice, it is very difficult to completely prevent the metal substrates from coming into contact with water, oxygen or electrolytes for a long period of time. From an inhibition standpoint, at least we can delay the corrosion process by limiting the penetration of water and oxygen, preventing the formation of cathode, and impeding the electrolyte flows. In other words, it can be concluded in barrier properties, adhesion properties and electrochemical impedance of coatings.
Development of binders with improved barrier properties and adhesion properties is attainable via polymer structural engineering, morphology design, hydrophobic embedment and incorporating functional groups. In this work, to improve the barrier and adhesion properties of PUD binders, three different approaches were proposed:
a) introducing hydrophobic building blocks into the polymer structure, which is believed to reduce water uptake and penetration,
b) incorporating silane functional groups for crosslinking,
c) using BPA ethoxylate to construct urethane linkages with isocyanates that might improve adhesion to metal substrates.
Modification through silane crosslinking is also known to enhance coating performance in solvent resistance, adhesion and mechanical properties. 10 Moreover, a recent study has shown incorporating BPA alkoxylates improves anti-corrosion performance in conventional 2K polyurethane systems. 11 A similar approach was applied to water-based polyurethane dispersions system in this study.
In a nutshell, three different modifications were solely applied or integrated in preparation of seven different polyurethane dispersions in this study. The synthesized PUDs were then formulated in DTM coatings and applied to cold rolled steel (CRS) panels.
The coatings’ performance was evaluated to compare the effectiveness of each modification on the coatings’ general properties, water resistance, barrier properties, adhesion properties and anti-corrosive performance via salt spray test (SST). Based on the test results, we sought to develop the prototype anti-corrosive PUDs for DTM application.
Experimental
Materials
Commercially available linear polyols with average molecular weight 2,000 Da and isophorone diisocyanate (IPDI) were used to prepare the polyurethane backbone structure in this work. Polycarbonate diol was used in all syntheses except for one sample in which a polyester diol was used for comparison purposes. Bisphenol-A (BPA) ethoxylate and proprietary hydrophobic polyols were used in modification of the polyurethane backbone in the 10-15 wt% range. A hydrophilic diol, dimethylolpropionic acid (DMPA), was used as a potential ionic center. Distilled 2-butanone (MEK) was used as a diluent in the prepolymer reaction.
Catalyst (dibutyltin dilaurate, DBTDL), chain extenders (amino functional silane, and ethylenediamine, EDA) and a neutralization agent (triethylamine, TEA) were purchased from Sigma-Aldrich. All raw materials were used as received.
PUD Synthesis
PUDs were synthesized by the conventional prepolymer process. The NCO/OH ratio was kept constant for all PUDs. The general synthesis procedure was as follows. Polycarbonate diol (MW 2000) and DMPA were charged into a dry, clean glass reactor under nitrogen atmosphere and heated to 70 °C. IPDI was then added under constant stirring. The mixture was held at 80 °C for 4 hrs in the presence of 0.006 wt% of DBTDL, and a small amount of MEK (for viscosity control) to yield a NCO-terminated PU prepolymer. After the prepolymer reached the theoretical % NCO, it was neutralized with TEA, and mixing was continued for 30 min at 55 °C. The neutralized prepolymer was slowly charged into a calculated amount of deionized (DI) water under high-speed agitation, and the resulting aqueous dispersion was later chain extended with ethylenediamine (EDA). The MEK was later removed under vacuum to yield a stable, solvent-free PUD (solid content 32-35%). A series of samples was prepared by varying the composition of polyols and chain extenders with constant ionic content.
PUD Modifications
The object of this study was to evaluate the effectiveness of various modifications to the PUD structure on water resistance and anti-corrosive performance. Hydrophobic modification, introduction of BPA ethoxylates and structural design by crosslinking the silanol groups of the silylated polyurethane were targeted, as shown in Table 1.
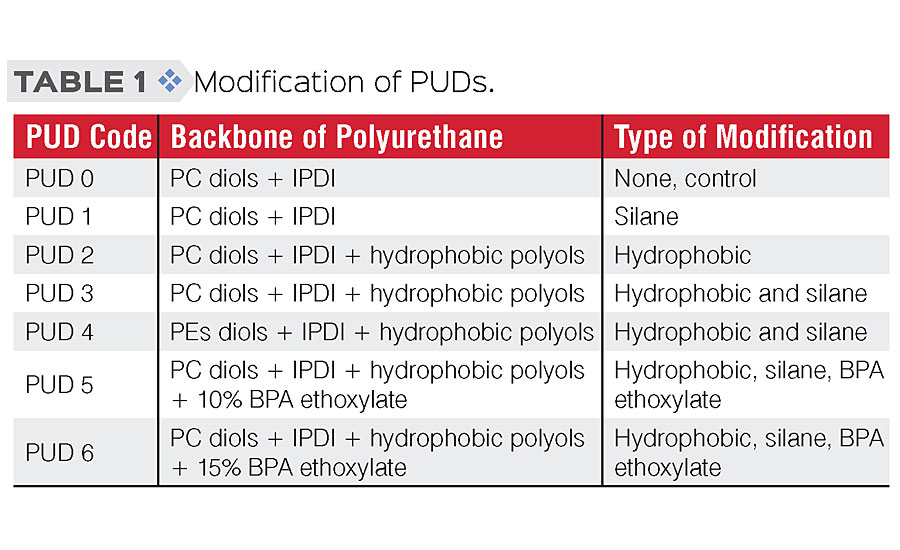
Formulation of Waterborne DTM Coatings
Proper formulation of PUDs into DTM coatings is very important to prevent early failure caused by poor film formation and coating defects. Additives such as defoamers, wetting agents and coalescence aids (co-solvents) were used in the low-VOC coatings formula (Table 2). The coatings were applied to CRS panels using a 6 mils wet film drawdown bar or spraying, flashed for 15 min under ambient condition, and then cured for 30 min at 70 °C to yield dry films with thickness in the range of 2.5 ± 0.5 mils.
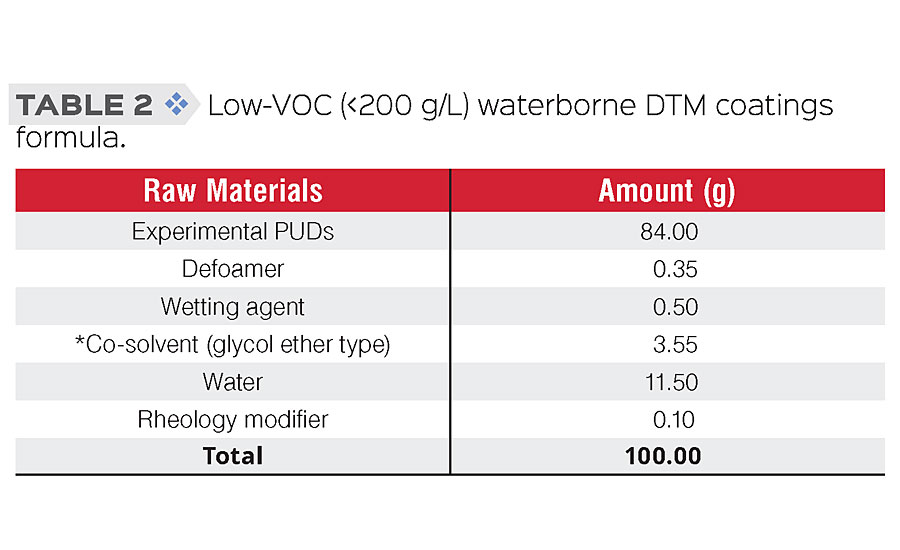
Characterization and Testing
General physical properties and film properties of PUDs were tested according to standard procedures. Testing results are shown in Table 3 (physical properties) and Table 4 (film properties). As the results show, we were able to modify those PUDs while retaining similar physical and general film properties.
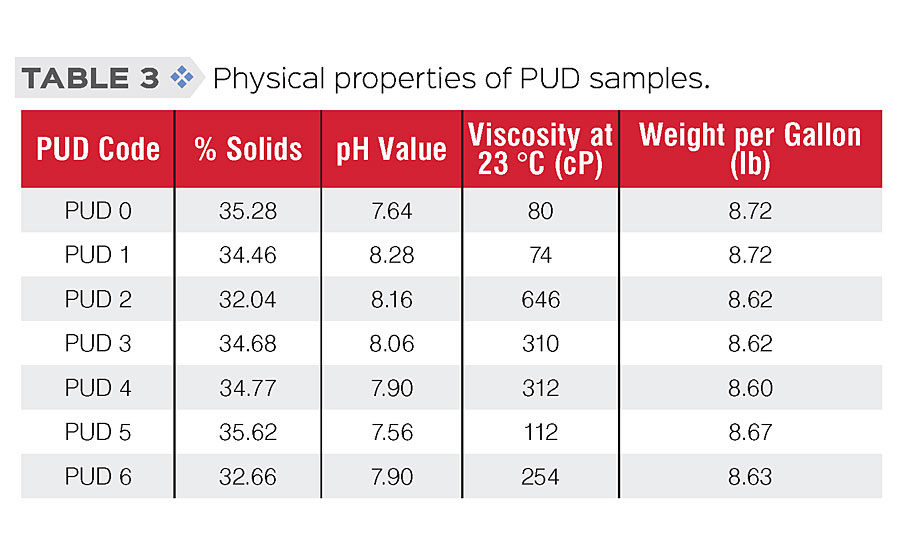
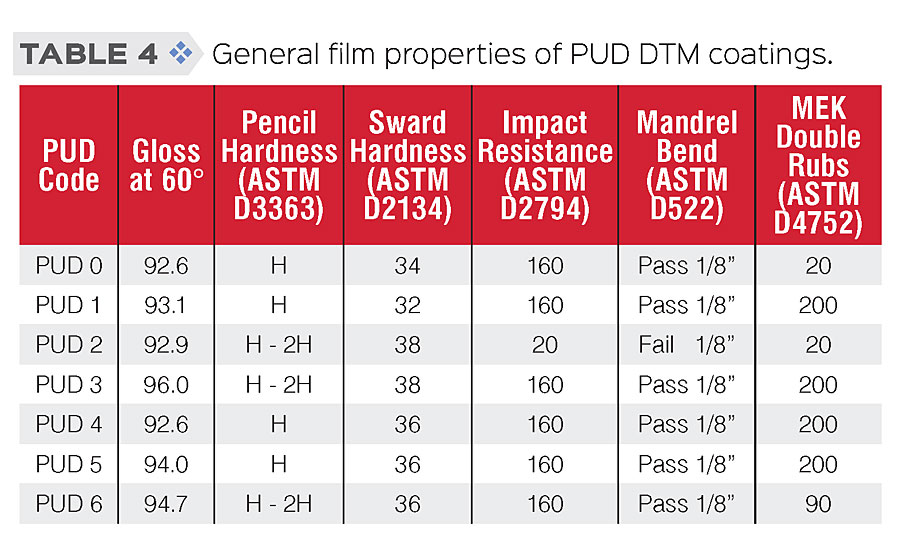
Barrier Property and Corrosion
Based on the corrosion protection mechanism, the corrosion process can be inhibited by prevention of water/oxygen penetration and anode/cathode formation, and limitation of electrolyte (ions) movement in the binders. Several tests were selected to evaluate the barrier property and adhesion property of PUD DTM coatings.
Anti-corrosive performance of the DTM coating was evaluated by SST according to ASTM B117, and monitored for corrosion at 100, 200 and 300 hrs. (Most failure seen after 300 hrs).
Water exclusion performance was evaluated by measurement of static contact angle, water uptake upon water immersion for 24 and 168 hrs, and early water resistance by 1-hr water dwell test.
Contact angle tests were conducted using the sessile drop method on DTM coated CRS panels at constant room temperature.
To determine early water resistance, PUD DTM coatings were applied to sealed Leneta cards at 6 mils wet film thickness and allowed to dry at ambient condition (23 °C) for 72 hrs. A water dwell test was conducted by putting 2 mL of water on the coating surface for an hour (covered with a watch glass to minimize evaporation).
To determine water absorption, PUD-free films were cast and prepared in 40 mm x 12 mm strips at a thickness 0.5 ± 0.1 mm. The free films were conditioned in a 50 °C oven for 24 hrs, and then fully immersed in water at ambient condition for 24 hrs and 168 hrs. The weight of the films before and after immersion testing was accurately measured in an electronic balance with an accuracy of 0.0001 g. Water absorption was calculated using the equation:
Water Absorption, % = (Wet Weight – Conditioned Weight)/(Conditioned Weight) ×100
Adhesion and Peel Strength
Adhesion test was performed according to ASTM D3359 on coated aluminum and CRS panels with a crosshatch drawn and removed with adhesive tape. Adhesion of PUDs to metallic substrate is generally very good. Therefore, peel strength on CRS panels (T-peel test) was further tested in this study. The test method was adapted from standard ASTM D1876. PUDs DTM coatings were applied to 4” by 1.25” cold rolled steel with an inter-layer of 4” by 0.75” nylon fabric. Two coats were needed to ensure full coverage of PUDs on top of nylon strips. A schematic test specimen is shown as Figure 1, and autographic recording of load versus distance peeled was done by MTS testing machine.

Results and Discussion
A series of waterborne PUDs based on polycarbonate diols and IPDI were synthesized and modified through composition and structural engineering to achieve the desired properties. Low-VOC water-based DTM coatings were prepared and applied to cold rolled steel (CRS), and their film property and anti-corrosive performance were evaluated. In general, these coatings showed very good appearance (high gloss) and film properties, such as good balance in hardness and flexibility, and excellent solvent resistance (MEK D-rubs >200), especially for those PUDs with silane modification.
Effect of Hydrophobic Modification on Barrier Properties
Water absorption was measured to determine the polymer hydrophilicity and the accessibility of water, which was expected to have a substantial impact on corrosion inhibition. Water exclusion properties of each DTM coating, as characterized by static water contact angle and water absorption test, are shown in Figure 2 and Figure 3, respectively.
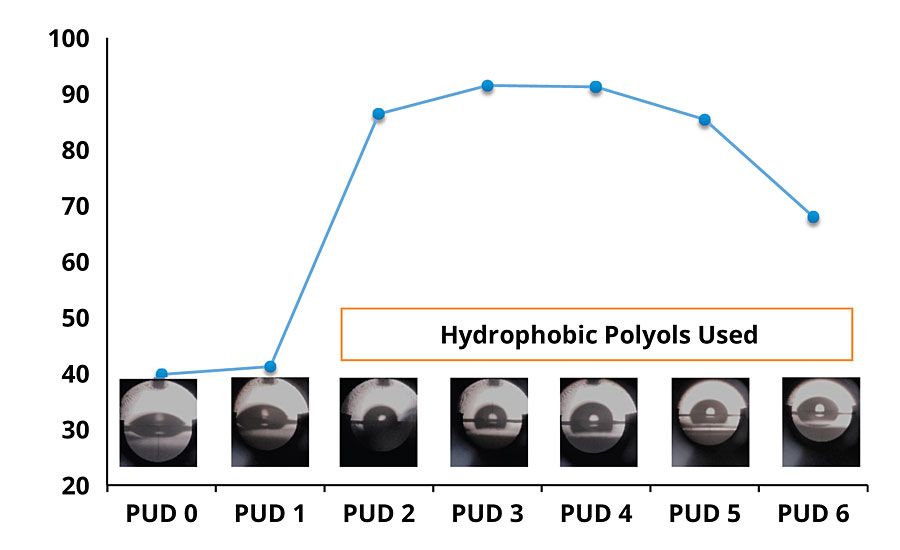
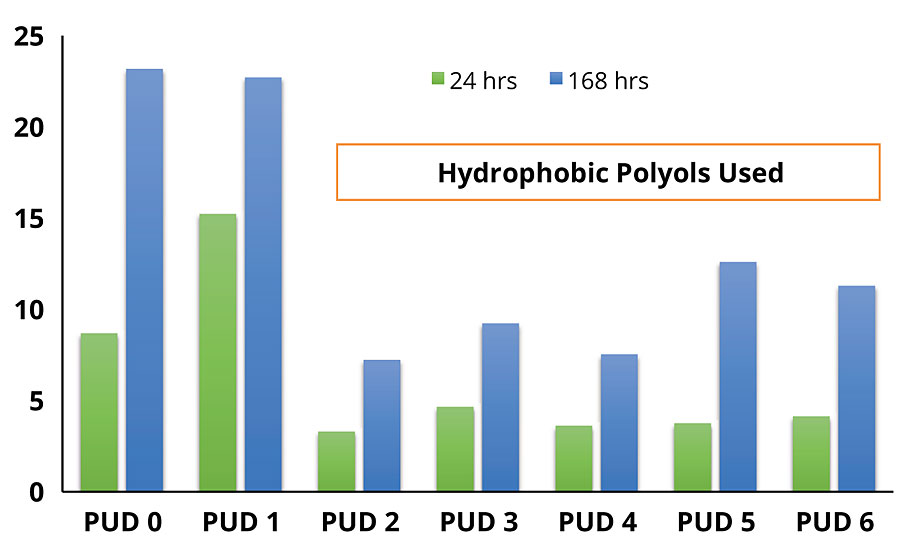
As can be seen, there is a remarkable difference in contact angle and water absorption between the DTM coatings based on non-hydrophobic modified PUDs (PUD 0, 1) and hydrophobic modified PUDs (PUD 2, 3, 4, 5, 6). In addition, the built-in hydrophobic building blocks also help to develop early water resistance, which is beneficial to outdoor/field applications. Significant improvement in water resistance was noted with PUDs 2-6, with no damage by the water dwelling test after ambient cure for 72 hrs (Figure 4).
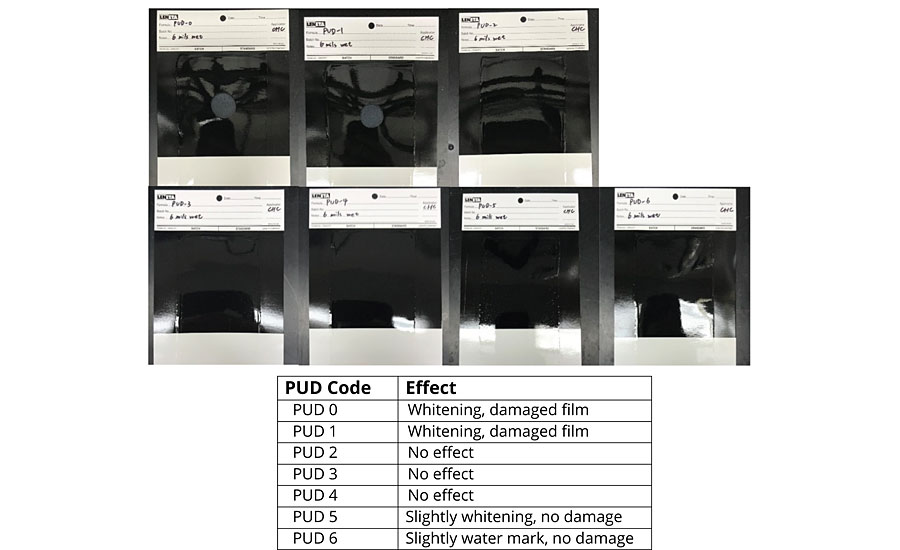
Adhesion Properties
Adhesion properties of DTM coatings were tested by crosshatch and tape test, and the peel strength was analyzed on CRS panels by T-Peel test. All coatings scored 5B on both CRS and aluminum substrates according to ASTM D3359. Peel strength on CRS panels provides further insights on the impact of each modification (Figure 5).
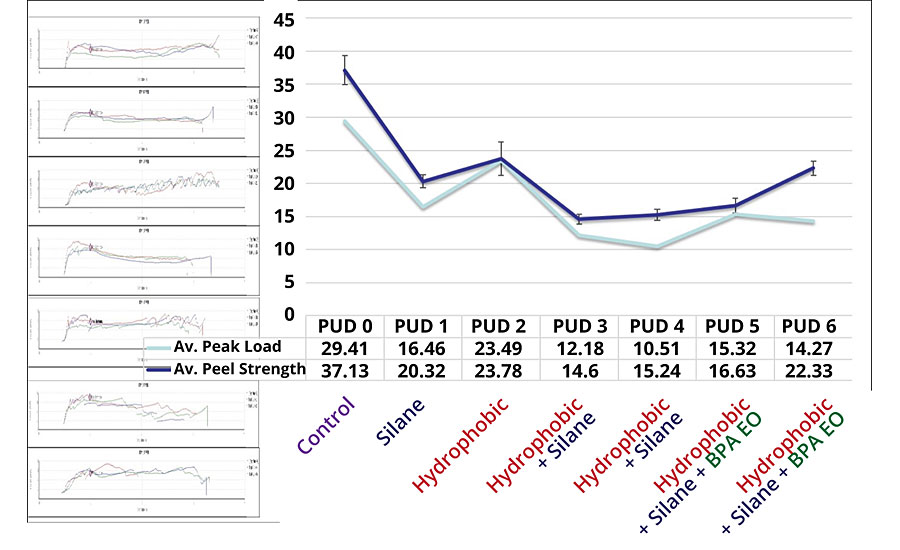
Effect of Silane Modification on Adhesion
A comparison between PUD 0 and PUD 1, and between PUD 2 and PUD 3 shows a trend of decreasing peel strength among two sets of DTM coatings with and without silane modification. The results indicate that silane modification to PUDs may have a negative impact on peel strength, presumably because self-crosslinking may have already occurred in the PUD particles instead of forming covalent bonding to metallic substrate. Moreover, increased rigidity of polymer chains may limit the orientation of polar groups, resulting in reduced surface energy (increase in water contact angle). A study on the effect of chain length for silane adhesion promoter explained the decrease of adhesion based on entropically favorable silane chain conformations. 12
Effect of Hydrophobic and BPA Ethoxylate Modification on Adhesion
A comparison between PUD 0 and PUD 2, and between PUD 1 and PUD 3, i.e., between two sets of DTM coatings with and without hydrophobic modification, also showed the negative effect on peel strength. This may have been because the hydrophobic building blocks reduced the polarity of the polymeric system. On the other hand, the introduction of BPA ethoxylates in the backbone had a positive effect on peel strength [comparing PUD 3 to PUD 5 (10%) and PUD 6 (15%)] due to the increase of polarity from phenolic alkoxylates groups.
Coating adhesion is a complex phenomenon that is affected internally and externally by a number of factors including surface energy, chemical bonds, surface roughness, cleanness, and mechanical strength of binders and substrates. In this study, the synthesized waterborne PUDs are relatively harder than other waterborne coatings and may have resulted in higher build-up of internal stress during curing, resulting in lower bonding strength.
Effect of Different Modifications on SST Performance
The performance of the PUD-based DTM coatings in the neutral SS over 100, 200 and 300 hrs are shown in Figure 6. PUD 0 and PUD 1 synthesized without hydrophobic building blocks failed badly in the SST (including blistering, corrosion and scribe creep) compared to other PUDs, indicating that hydrophobic modification significantly improved the corrosion resistance. The effect of silane modification was noted by comparing PUD 2 with PUD 3, as corrosion along with the scribed area was much reduced after 300 hrs. Thus, crosslinking of silanol groups is seen to further enhance the barrier property by impeding moisture/ion penetration. PUDs based on polycarbonate diols (PUD 3) performed slightly better than polyester diols (PUD 4) with the same modifications and similar hydrophobicity and adhesion property. BPA ethoxylate-modified PUDs (PUD 5 and PUD 6) did not significantly improve the corrosion resistance compared to PUD 3; however, better adhesion performance was noted at higher BPA ethoxylate content (PUD 6).
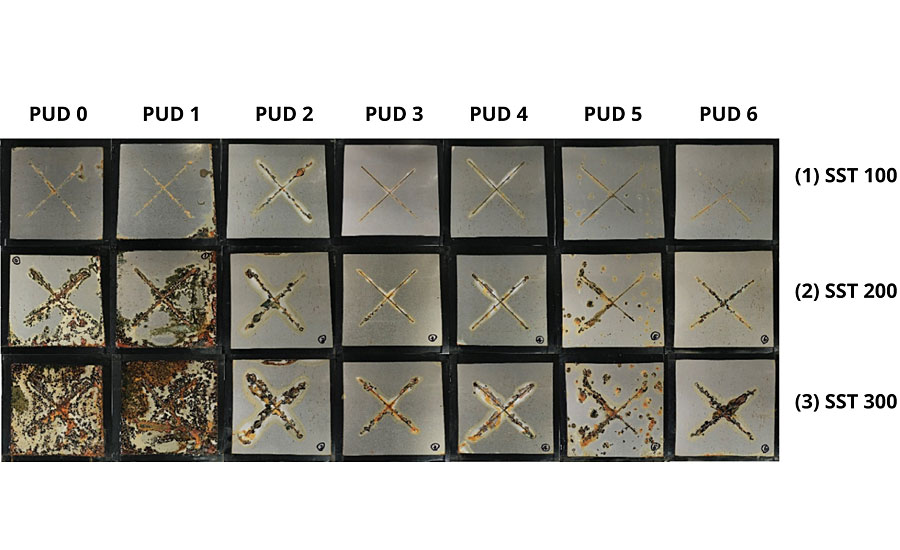
Based on the SST results, the barrier properties of the bulk coatings are seen to play a more important role than its adhesion properties, as the peel strength analysis was not highly correlated with anti-corrosive performance. A similar observation was noted in the corrosion resistance study of waterborne styrenated-acrylic systems, i.e., good adhesion does not necessarily result in good corrosion resistance.
13
Thus, hydrophobic modification is considered to be the most effective and prominent approach to improve corrosion resistance, and silane modification further enhances the corrosion protection and coating properties.
Although BPA ethoxylate incorporation did not have a significant effect on corrosion protection, it did enhance adhesion to metal substrates, which can be found in peel strength test result of PUD 6.
Conclusions
The object of this study was to evaluate the effectiveness of different modifications on anti-corrosive performance. Straight PUDs were used to prepare DTM coatings with only small amounts of additives and without the assistance of corrosion inhibitors, passivation fillers or pigments. Each modification was reviewed and discussed by comparing the coating performance for water exclusion, adhesion and how they correlated to corrosion protection. The effective barrier properties achieved by introducing hydrophobic building blocks and organosilane could dramatically reduce the penetration of water and ions, resulting in improved anti-corrosive performance and early water resistance. However, these two modifications both showed a negative impact on adhesion to metal substrates; incorporation of BPA alkoxylate could be a solution for adhesion enhancement.
While solvent-based coatings still dominate the protective coatings market, research efforts to improve the performance of waterborne binders through polymer architecture and morphology design, hydrophobic embedment and corrosion-inhibiting function groups have demonstrated commercial success in styrene-acrylic latex and alkyd emulsion-based systems.
However, conventional PUDs are considered susceptible to water because the presence of hydrophilic ionic centers, e.g. carboxylic, sulfonic or phosphoric groups, create a path for water and corrosive ions to diffuse through the coating. In this study, the use of ionic groups in experimental PUDs was not minimized so as to ensure a continuous and stable dispersion system. By optimizing the hydrophilic content in PUDs, further improvements in corrosion protection may be achievable. Moreover, post-addition of external crosslinkers such as polycarbodiimides that react with carboxylic groups may further boost coating performance.
14
The ultimate goal is to develop a low-environmental-impact, user-friendly 1K DTM polyurethane binder with superior coating performance and corrosion resistance.
References
1 Honarkar, H. Waterborne Polyurethanes: A Review, J. Disper. Sci. Technol., 2018, 39(4), 507-516.
2 Dieterich, D. Aqueous Emulsions, Dispersions and Solutions of Polyurethanes; Synthesis and Properties, Prog. Org. Coat., 1981, 9(3), 281-340.
3 Bai, C.Y.; Zhang, X.Y.; Dai, J.B.; Zhang, C.Y. Water Resistance of the Membranes for UV-curable Waterborne Polyurethane Dispersions, Prog. Org. Coat., 2007, 59(4), 331-336.
4 Ramezanzadeh, B.; Mahdavian, M.; Ghasemi, E.; Changizi, E.; Mohamadzadeh Moghadam, M.H. Covalently-Grafted Graphene Oxide Nanosheets to Improve Barrier and Corrosion Protection Properties of Polyurethane Coatings, Carbon, 2015, 93, 555-573.
5 Yeh, J.; Liou, S.; Lai, C.; Wu, P.; Tsai, T. Enhancement of Corrosion Protection Effect in Polyaniline via the Formation of Polyaniline − Clay Nanocomposite Materials, Chem. Mater., 2001, 13 (3), 1131-1136.
6 Rahman, M.M.; Zahir, H.; Haq, B.; Al Shehri, D.A.; Kumar, A.M. Corrosion Inhibition Properties of Waterborne Polyurethane/Cerium Nitrate Coatings on Mild Steel, Coatings, 2018, 8(1), 34.
7 Child, T.F.; van Ooij, W.J. Application of Silane Technology to Prevent Corrosion of Metal and Improve Paint Adhesion, T. I. Met. Finish, 1999, 77(2), 64-70.
8 van Ooij, W.J.; Zhu, D.; Stacy, M.; Seth, A.; Mugada, T.; Gandhi, J.; Puomi, P. Corrosion Protection Properties of Organofunctional Silanes - An Overview, Tsinghua Sci. Technol., 2005, 10(6), 639-664.
9 Clamen, G.; Ferrari, T.; Fu, Z.; Hejl, A.; Larson, G.; Procopio, L.; Rosano, W.; Sheppard, A.; Swartz, A. Protection of Metal with a Novel Waterborne Acrylic/Urethane Hybrid Technology, Prog. Org. Coat, 2011, 72 (1-2), 144-151.
10 Lai, X.; Shen, Y.; Wang, L. Preparation and Properties of Self-Crosslinkable Polyurethane/Silane Hybrid Emulsion, J. Polym. Res., 2011, 18, 2425-2433.
11 Spilman, G.E.; Christy, M.; Comstock-Reid, B. High Performance Coating Materials from Recycled Sources, The CoatingsTech Magazine, 2017, 14(10), 44-57.
12 Jenkins, M.L.; Dauskardt, R.H.; Bravman, J.C. Important Factors for Silane Adhesion Promoter Efficacy: Surface Coverage, Functionality and Chain Length, J. Adhes. Sci. Technol., 2004, 18(13), 1497-1516.
13 Bulick, A.S.; LeFever, C.R.; Frazee, G.R.; Jin, K.; Mellott, M.L. The Impact of Film Properties on Corrosion Resistance in Waterborne Acrylics and Next Generation Low VOC Resin Development, Proceedings of the 44th Annual International Waterborne, High-Solids, and Powder Coatings Symposium, New Orleans, LA, 2017, 19-24.
14 Hesselmans, L.C.J.; Derksen, A.J.; van den Goorbergh, J.A.M. Polycarbodiimide Crosslinkers, Prog. Org. Coat., 2006, 55(2), 142-148.
For more information, email Chung-Hsuan.Chen@anddev.com or call (517) 438-5217.
Looking for a reprint of this article?
From high-res PDFs to custom plaques, order your copy today!