Introduction to IR-Reflective Pigments
While the human eye is sensitive to only a small part of the electromagnetic spectrum, pigment interactions with wavelengths outside the visible can have interesting effects on coating properties. One key area of the spectrum is the infrared (IR), specifically the near infrared. While not visible to the human eye, a pigment's, and thus a coating's IR properties can affect usability and durability.
The primary purpose of IR-reflective coatings is to keep objects cooler than they would be using standard pigments. This IR-reflective feature is the basis for their use in markets like Cool Roofing for the EPA's Energy Star Program1 and the California Energy Commission2 Title 24 2008 version. This technology is also finding use in transportation and other areas where the ability to stay cool is a valuable benefit.
The easiest way to increase IR reflectivity is to use white pigments like titanium dioxide. TiO2 reflects in the visible and in the infrared. The key to fight this "White Blight" and produce innovative, colored IR-reflective coatings is to use pigments that absorb in the visible to produce color and reflect in the IR for coolness. From these demands, Shepherd Color has developed a line of highly engineered products called Arctic® IR-reflective pigments. The Arctic line of pigments provides a palette of colors that allows the formulation of coatings and the design of materials to meet infrared reflectivity and long-term durability requirements, and provide deep and rich colors.
Articles have been written about the pigments used to make infrared reflecting coatings. This article is meant to inform chemists and formulators about some specific issues and phenomena pertinent to formulating and optimizing IR-reflective coatings. Some variables and factors that can affect a coating's IR reflectivity are individual pigment selection, milling and dispersing, mixing IR-reflective pigments, opacity, and contamination.
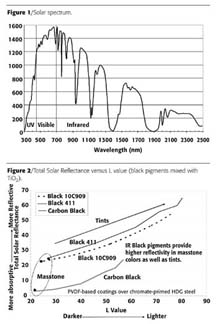
Solar Spectrum
Any discussion of IR coatings requires a short review of basic physics. The sun's energy that reaches the Earth's surface is divided into three parts.
For an object in an outdoor environment, the four main mechanisms of reflectivity, emissivity, convection and conduction determine its temperature. Convection is largely dependant on air flow, and conduction depends on how well an object is insulated to prevent heat flows. Reflectivity and emissivity are the factors that can be manipulated.
Cool Mechanisms
Objects reflect or absorb solar energy from these three regions: UV, visible and infrared. Total Solar Reflectance (TSR) describes how much of the sun's energy an object reflects. The common instrument for determining TSR is the Devices and Services3 Solar Spectrum Reflectometer Model SSR, more commonly known as the "D&S". The D&S returns a single number for the TSR, while a spectrophotometer reads individual wavelengths that can be used to make the spectral reflectance curves seen in this article. Reflectivity can be manipulated by the careful selection of high-IR-reflective Arctic pigments. The key is to reflect infrared and absorb and reflect in the visible region to produce the needed color.Cool Coatings System
Shepherd Color Company supplies pigment to the high-performance coatings market. These products, including the Arctic line, are highly engineered ceramic pigments. These pigments, also called mixed metal oxides (MMO) or complex inorganic colored pigments (CICP), provide lasting color for demanding applications. The inorganic ceramic nature of the pigments provides resistance to high temperatures, chemicals, acids, bases, weathering and environmental pollutants.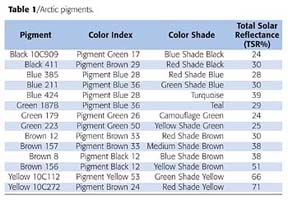
Colormatching Blindfolded Pigment Selection
The highest reflective pigments should be chosen for cool coatings. Carbon black, iron oxide black or copper chromite black are standard black pigments for most formulations, but they have very low infrared reflectivity and a TSR of about 6%. One key to formulating cool coatings is the use of an infrared-reflecting black pigment. In general, IR-reflective formulations incorporate Arctic Black 10C909 to lower the L value in colors made with the other Arctic colors. Black 411 provides a higher TSR, but with a redder undertone. A complete listing of Arctic pigments (Table 1) provides a nearly full color gamut to use to help formulate high-IR-reflective coatings.
This advantage in TSR for the masstones also continues when the pigments are added with TiO2 to make tints. Figure 2 portrays TSR on the vertical axis and the lightness L value on the horizontal axis. Each line represents a different pigment with the dot on the left-hand side (low-L-value) as the masstone. Adding white increases the L value and increases the TSR. What you can see from the graph is that low-L-value masstone colors with around 25% TSR can be achieved with IR-reflective blacks, while standard blacks need to be mixed to a light to medium gray before they can achieve 25% TSR.
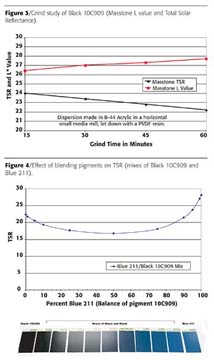
Dispersion
Arctic pigments are compatible in almost all solvent and aqueous coatings systems, including polyesters, acrylics and fluoropolymer systems. To achieve full dispersion and optimum properties, the pigments should be dispersed in a small media mill to at least a 7 Hegman. Care must be taken not to over grind the pigment. Additional grinding will break the pigment into smaller particles, causing color shifts, which usually increase tint strength but lightens masstones. Consistent grinding past the dispersion step needs to be carefully controlled to maximize color and IR properties. Figure 3 shows that with increased grinding, Arctic IR Black 10C909 moves lighter in the masstone and lower in TSR, both of which are undesirable.
Many additives can be used to produce stable dispersions. In some systems no additives are needed due to the inherent dispersion properties of the pigments. Due to their high specific gravity, careful screening of finished paints for pigment settling should be conducted.
Blending Pigments
Very few colors are single-pigment dispersions. In order to match a color, care must be taken when more than one pigment is mixed together to make a color. As seen above, any Arctic pigment mixed with white will provide a higher total solar reflectance than the Arctic pigment by itself. Two Arctic pigments with different absorption areas, when mixed together, will have a lower reflectance than the pigments have individually. A good example of this is a mixture of Arctic Blue 211 and Black 10C909. While both have about 25-30% TSR, when combined they will have a lower TSR than a weighted average of the individual pigments, as seen in Figure 4.
An examination of the spectral curves in the infrared (Figures 5 and 6) shows that the black's reflectance comes just as the cobalt absorption band of the blue starts. This is to be expected since pigmented films don't really reflect; they either absorb, scatter or transmit. The absorbance of the pigment over-powers the scattering. This battle between absorbance and scattering is predominate over transmittance in thick objects. But in the relatively thin world of paint films, transmittance can also play a factor.
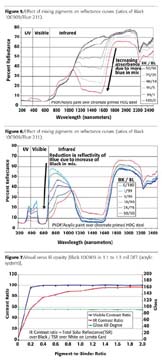
Opacity
CICPs, such as Arctic pigments, are known for their high visible opacity. What is harder to see is that since they don't absorb in the IR region, the only two mechanisms left are scattering and transmission. Thin films may not completely scatter and reflect the sun's energy back out of the coating, so the IR energy may continue through to the substrate.Figure 7 shows how a paint film can be visually opaque, while still semi-transparent in the IR. A solventborne air-dry acrylic was used to make coatings with varied pigment-to-binder ratios. The paints were then drawn down over the black and white portion of Leneta cards. The visible contrast ratio was read, and a "TSR contrast ratio" was determined by dividing the TSR reading over the black part of the card by the TSR over the white portion of the card. For each pigment-to-binder ratio, P/B, the respective contrast ratio was plotted, along with the 60 degree gloss of the coating. As can be seen, the films obtained visual opacity much sooner than IR opacity. Along with P/B ratios, a similar behavior can be seen if the P/B were held constant and the film thickness increased.
This difference in visual and IR opacity leads to many issues. A pigment's and a coating's TSR can depend on the substrate and film thickness. This makes it difficult to predict TSR for a pigment or coating without knowing particulars about its application and use. A gray primer with carbon black in it will cause a greater loss in TSR than if a similar color primer is made with IR black, other non IR absorbing pigments, or left white.
Along with these negatives, the positive is that an IR-reflective substrate can help to keep a coating's TSR higher. A different take on the graph in Figure 7 is the graph that is shown in Figure 8, which illustrates the visible contrast ratio along with the TSR readings of the coating containing the Black 10C909. The red and blue lines represent the TSR over white and over black respectively. Not surprisingly, the TSRs differ while the film is not visually opaque, but the TSR is also higher over the white when visual opacity is reached. As the P/B increases and hides the black and white portions of the Leneta card, the TSR numbers start to converge. In the range of about 0.4 to 0.8, the Black 10C909 film shows good visual opacity and the ability to maximize the coating's TSR over reflective substrates.
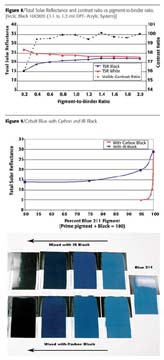
Contamination
One last area of concern is contamination. Figure 9 shows the curve previously shown that demonstrates the decline in TSR when Black 10C909 and Blue 211 are mixed together. Even more damaging to TSR is the inclusion by design or contamination of a non-IR-reflecting black, like carbon black. The carbon black greatly affects the TSR of the mix when as little as 0.10% is included, and before the color starts to change dramatically, as seen in the picture. The two lessons that this shows is that mills and handling equipment must be clean to make sure that cross-contamination doesn't occur and that using even small amounts of non-IR colors to shade a batch can have drastic effects on TSR.Conclusion
The formulation of IR-reflective coatings for various applications depends on many factors, some of which cannot be seen with the naked eye. There are two main keys to formulating these coatings. The first has to do with the physical characteristics laid out in this article.
The second key is to work with a partner with the products, research, and most importantly, technical support to allow you to formulate, test and validate your IR-reflective coatings. The IR range is invisible to the human eye, not covered by standard spectrophotometers, and measurable only by expensive and specialized equipment. A partner who can shepherd you in pigment selection, color matching and testing, along with guidance in the different regulations and programs can be an invaluable aid in formulating, marketing and supporting differentiated IR-reflective coatings.
References
1 EPA Energy Star Roofing: www.energystar.gov/ index.cfm?c=roof_prods.pr_roof_products.
2 California Energy Commission: www.energy.ca.gov/.
3 Devices and Services: 10290 Monroe Dr., Ste. 202, Dallas, TX 75229; 214/902.8337;
www.devicesandservices.com.
Arctic® is a Registered Trademark of The Shepherd Color Company.
IR-Reflective Coatings Benefits
General Benefits
Roofing Benefits
Looking for a reprint of this article?
From high-res PDFs to custom plaques, order your copy today!