Troubleshooting Metal Catalyzed Urethane Systems
The reaction of isocyanates with hydroxyl groups is very slow in the absence of a catalyst. Metal catalysts are often used to achieve sufficient reaction rates and cured properties for a variety of systems including coatings, elastomers, sealants and potting compounds. Systems crosslinked with aliphatic isocyanates are particularly dependent on catalysts to accelerate the reaction. Aromatic isocyanates are generally more reactive than aliphatic types and only require the use of catalysts under certain conditions.
Tin compounds have served the industry well. Dibutyltin dilaurate (DBTDL) can be considered the workhorse catalyst for urethane coatings. It is efficient, e.g., a very low level of catalyst will greatly increase the NCO/OH reaction rate. However, as with any catalyst, certain problems can be encountered with DBTDL, including issues of stability-reactivity, hydrolysis of ester groups, catalysis of the water/isocyanate reaction and environmental concerns.
It would be preferable to use a two-component urethane catalyst that could provide fast dry times of cast films, but allow good pot life under ambient conditions. The catalyst should remain active as the catalyzed component is aged for extended periods and have minimal effect on resistance properties. Also, the catalyst should be environmentally acceptable. Organotin compounds are currently being scrutinized in the area of antifouling systems. Tributyltin compounds are of particular concern for the marine industry. The International Maritime Organization is calling for a complete ban of tin-based antifouling coatings1 with some affects now being felt beyond marine coatings. Some manufacturers are taking precautionary steps to eliminate organotin catalysts in expectation of possible future legislation. An apparent trend is developing in segments of the European market with certain U.S. export companies also feeling its affect.

Factors to Consider When Selecting a Catalyst
The process of selecting a catalyst depends on several factors. Addition of metal catalysts in concentrations that can be measured in ppm has a profound effect on the reaction rate, yet very low concentrations of impurities can also effect the reaction rate. Often resins, additives and pigments contain impurities that can interact with catalysts and deactivate them, or the impurity itself can act as a catalyst. Catalyst deactivation can also be a function of water content or acid number of the resin. In addition, impurities have different effects on different metal catalysts.Furthermore, catalyst selection will also depend on the desired coating properties and pot life requirements. Certain catalysts can provide superior pot life while others can provide fast, selective acceleration of the primary reactants when moisture is present. It is difficult to find a universal catalyst that meets all requirements for every application.
DBTDL and dibutyltin diacetate (DBTDA) are very versatile catalysts for the NCO/OH reaction and are commonly used as urethane catalysts. Many of the reactions thought to be typical for isocyanate reactions are actually the reactions of isocyanates under DBTDL catalyzed conditions.
A range of organotin-free catalysts has been developed that are based on a variety of metals, including bismuth, aluminum and zirconium. These catalysts, marketed under the K-KAT® tradename, are not only environmentally more acceptable than organotin compounds, but they can also offer performance advantages over DBTDL catalysts. K-KAT catalysts can be selected that have advantages compared to DBTDL with regard to reduced water reaction, improved pot life, faster cure, improved catalysis in cationic electrocoating, and reduced hydrolysis of polyester resins.
Usually lower catalyst concentrations are used in low-solids systems that use high-molecular-weight resins. The resins in these systems can sufficiently form films with little or no crosslinking while the excess solvent contributes to maintaining acceptable pot life. However, catalysts are generally required in high-solids systems that use low-molecular-weight resins that are often required to achieve stringent VOC regulations. These low-molecular-weight systems require extensive crosslinking and molecular weight increase to achieve formed films. Pot life extension of metal-catalyzed high-solids systems can be achieved with addition of volatile diketone chelating agents, such as 2,4-pentanedione.2
The catalytic activity of DBTDL and most other catalysts in a high-solids coating is a linear log time/log catalyst concentration relationship, as shown in Figure 1. At the typical pot life for this high-solids formulation (pot life being defined as time to double the initial viscosity) about 6% of the free isocyanate is consumed.3 Based on the graph, a metal catalyst concentration of 0.01% tin can provide a pot life of 10 minutes and a through dry time of 100 minutes. Decreasing the metal concentration to 0.001% tin will increase the pot life to 100 minutes and the through dry to 1,000 minutes. It can be seen that without a volatile chelating agent it is difficult in such a system to balance pot life with acceptable reactivity.
The rate of the reaction can also be followed by infrared spectroscopy with the disappearance of the isocyanate band at 2272 cm-1 wave number as a simple measure of the reaction rate. For a known polymer system, the pot life and tack free times are a function of isocyanate conversion. For example, a doubling of viscosity of a high-solids polymer system might be attained at 6% isocyanate conversion, and the cast film might require 30% conversion of the isocyanate to become tack free.


Catalyst Dosage
Figure 3 provides a guideline to non-tin catalyst concentration in comparison to DBTDL. Tests have shown that the effect of catalysts of different composition can be dependent on polyol and isocyanate structure.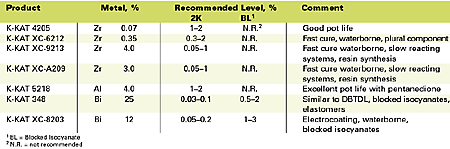
Catalyst Description and Applications
The catalysts that will be discussed in the troubleshooting section are summarized in Table 1. The table includes metal contents and recommended dose levels. In general, the K-KAT catalysts can be recommended for general industrial, automotive, appliance, maintenance, wood and refinish applications. K-KAT 348 can also be considered for coil, cathodic electrocoat, adhesives, elastomers, foams, sealants and roofing applications. DBTDL contains 18-19% tin and is usually used in applications and concentrations similar to K-KAT 348.K-KAT 4205
Based upon a zirconium chelate catalyst dissolved in 2,4-pentanedione, this catalyst offers improved reactivity and stability in two-component polyol/isocyanate coatings. By selectively catalyzing the hydroxyl versus the water reaction, more urethane linkages are formed. This catalyst is not suited for castings, moldings or elastomers since it is important that the 2,4-pentanedione can evaporate. The active catalyst content in this product is too low for slower reacting systems such as isophorone diisocyanate (IPDI)-trimer and slow reacting polyols. It is best suited for coatings that use <0.1% of DBTDL.K-KAT XC-6212
K-KAT XC-6212 is a zirconium chelate catalyst ideally suited for fast-curing high-solids and waterborne coatings. This catalyst must be added to the isocyanate component. Addition of 2,4-pentanedione permits pot life extension of solventborne formulations. Pot life of waterborne 2K systems does not suffer when catalyzed with XC-6212. The catalyst is recommended for plural component high-solids spray and waterborne applications.K-KAT XC-9213
K-KAT XC-9213 is a high-concentration zirconium catalyst with about 10 times more metal than K-KAT XC-6212. This product may crystallize at lower temperatures, but warming to 40ºC will re-dissolve crystals. It is best suited for formulations that are slow curing such as IPDI crosslinked coatings or formulations that require high catalyst levels.K-KAT XC-A209
This catalyst is a high concentration zirconium catalyst with about eight times more metal than K-KAT XC-6212 that has been dissolved in t-butylacetate to prevent crystallization. It is recommended for formulations that are slow curing such as IPDI crosslinked coatings.K-KAT 5218
K-KAT 5218 is an aluminum chelate catalyst formulated for extended pot life. Since aluminum is a less active metal, about 10 times the metal concentration is required compared to DBTDL. In the presence of 2,4-pentanedione, however, a very long pot life can be obtained with equal dry time compared to DBTDL. This catalyst should be incorporated after the 2,4-pentanedione has been added.K-KAT 348
K-KAT 348 is a bismuth carboxylate catalyst that provides properties similar to DBTDL with less environmental concerns. It is particularly effective in blocked isocyanate and elastomer systems. A moisture scavenger is sometimes required with this catalyst.K-KAT XC-8203
Also a bismuth carboxylate catalyst, K-KAT XC-8203 provides improved hydrolytic stability compared to K-KAT 348. It is more effective in high temperature applications.Troubleshooting
Catalyst deactivation in isocyanate systems is a common problem. It is often not recognized as such because of the high activity of most catalysts and is usually overcome by over catalyzing the formulation. Part of the catalyst is used in a sacrificial fashion. Potential sources for catalyst deactivation are water, high acid number resins, anions and pigments. Problems associated with pigments are often not directly related to the pigment itself, but rather to the water that the pigment carries into the coating or to the pigment surface treatment. These interactions can become apparent in various film properties such as gloss, cure response and appearance (yellowing). Many isocyanate catalysis problems are related to deactivation of the catalyst. In replacing DBTDL with K-KAT catalysts, additional considerations will apply. K-KAT catalysts are formulated at a different metal concentration than DBTDL, and replacement of DBTDL with a K-KAT catalyst based on equal weight of the catalyst as supplied, therefore, is usually not sufficient. As a first approximation the K-KAT catalysts can be used at an equal metal level to DBTDL, with the exception of K-KAT 5218 in which the aluminum should be approximately 10 times the level of tin. Another approach is to test the K-KAT catalysts in a formulation at levels recommended in the summary section.
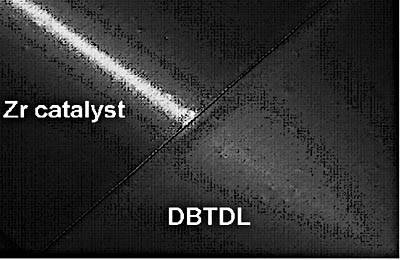
Variations in Gloss
The problem of gloss variation is often related to inconsistent moisture content in the formulation. Gloss can also be affected by the formation of insoluble reaction products, primarily hydrolysis products from the catalyst. Moisture can be incorporated into the system with solvent, pigments or the surrounding atmosphere. In many systems, particularly aqueous systems, the gloss can decrease as the paint is aged after the two components have been combined. Reduced gloss is an indication of moisture reacting with the isocyanate to form insoluble polyureas yielding carbon dioxide, which will result in gassing that can also reduce gloss. Lewis acid type catalysts, such as DBTDL, initially associate with the isocyanate to form a more electrophilic reactive site.4 Catalysts that follow an insertion mechanism initially associate with the polyol component and activate the hydroxyl groups. Certain zirconium complexes follow the insertion catalysis mechanism.5 In the presence of moisture, these zirconium catalysts selectively accelerate the reaction of the isocyanate with the polyol hydroxyl rather than with water resulting in a higher percentage of urethane linkages.2 Among the advantages that can be realized with this type of catalyst is higher gloss. To illustrate this point, a pot life study was conducted with K-KAT 6212 and DBTDL in a clear aqueous 2-component formula based on a polyester polyol6 and HDI (NCO/OH = 1.6). The coatings were applied four hours after mixing the two components. Reflection of a light across the clear urethane films over a black substrate demonstrates the improved gloss with the zirconium catalyst, shown in Figure 4. Although the catalysts provided equal cure response, bottles of gelled material photographed after overnight storage at room temperature (see Figure 5) clearly demonstrate decreased foaming with K-KAT 6212 compared to DBTDL. The volume of material in each container was initially equal. K-KAT XC-6212, K-KAT XC-9213 and K-KAT XC-A209 will perform similarly when catalyst concentration is based on equal metal content.
Loss of Cure on Aging
Loss of cure on aging can be related to the catalyst solubility or catalyst deactivation by acid groups, phosphate anions, pigment interaction and hydrolysis. Each of these effects may not be initially evident but can occur over a time period of days or weeks after adding the catalyst to the polyol component.Solubility of many catalysts, including DBTDL, can be limited. Finding the correct solvent combination is sometimes time consuming. In the case of K-KAT 348, polar solvents, in particular methyl ethyl ketone (MEK), can be troublesome. Storing prediluted catalyst for an extended period will increase the possibility of precipitate formation and catalyst hydrolysis. Minimizing MEK in a K-KAT 348 catalyzed system is recommended. However, K-KAT 348 solubility, or more precisely compatibility, could be related to hydrolysis of the bismuth carboxylate as the bismuth oxide/hydroxide that is formed will precipitate. Therefore, an apparent solubility problem can also be approached by minimizing moisture and preventing hydrolysis of the catalyst. Unlike other metal catalysts, K-KAT 348 is more stable under acidic conditions. In fact, adding equal parts of neodecanoic acid and K-KAT 348 will improve the hydrolytic stability and improve its compatibility in the system. Using a polyol with an acid number of greater than 10 can also improve the catalysts hydrolytic stability. Another approach to preventing hydrolysis is to add a moisture scavenger like para toluene sulfonyl isocyanate before the catalyst is added. Using polyols with low moisture content is also recommended.
In some instances, hydrolysis of the catalyst can be avoided by adding the catalyst to the dry isocyanate component. This approach should not be taken with Lewis acid type catalysts as they will accelerate the side reaction of isocyanate with contained impurities. Moisture may be incorporated during the process of mixing the catalyst with the isocyanate or while opening and closing the container. The only catalysts that are stable in the isocyanate component are the zirconium-based K-KAT XC-6212, K-KAT XC-9213 and K-KAT XC-A209. These catalysts should always be incorporated into the isocyanate component with mechanical agitation, preferably under nitrogen.
Figure 6 illustrates the stability of HDI trimer stored at 50°C for four weeks with and without K-KAT XC-6212 and DBTDL. Viscosity, as monitored with a Brookfield DV-II7 viscometer, was notably higher with DBTDL, particularly after three weeks of storage.
Poor Cure Response (Catalyst Deactivation by Acid Groups)
Catalyst deactivation can also occur if there is an excess of acid functional groups present. These acid groups can partially or completely displace ligands on the metal complex forming inactive salts. Specific remedies for prevention of metal catalyst deactivation depends on the catalyst. Deactivation by a high acid value system can be overcome by addition of a tertiary amine, for example dimethylethanol amine, to prevent deactivation of zirconium and aluminum catalysts. As mentioned before, higher acid conditions will actually improve hydrolytic stability of the bismuth catalyst, K-KAT 348, therefore, amine modification is not recommended with this catalyst. The incorporation method can also affect catalyst deactivation. Interaction of the aluminum catalyst with carboxyl groups is reduced by adding 2,4-pentanedione to the polyol component before the catalyst is added. Preblending K-KAT 5218 with 2,4-pentanedione, however, will result in the formation of poorly soluble precipitates. Figure 7 demonstrates the affect of 2,4-pentanedione on the activity of K-KAT 5218 in an acrylic/HDI system. The K-KAT 5218 catalyzed polyol components used in this study were stored at 50°C for seven days. Acid values of the polyol components were monitored along with surface dry of cast films. A slight decrease in acid value was noted (from 4.5 to 3.7) as the dry time increased (see Figure 7) in the system without 2,4-pentanedione. Acid value of the system with 2,4-pentanedione remained constant while only a slight increase in dry time was noted. This result indicates that the diketone reduces interaction of the catalyst with acid groups and prevents formation of inactive aluminum compounds.
Poor Cure Response (Catalyst Deactivation by Anions)
Impurities left over from the manufacturing process of polyol resins is an often overlooked factor in catalysis of isocyanate crosslinked coatings. These impurities can either accelerate the crosslinking reaction or inhibit the reaction by deactivating the catalyst. Polyether polyols may contain phosphate anions that are used to neutralize residual catalyst used in manufacturing the resin. These phosphate anions will also deactivate certain catalyst systems, particularly zirconium catalysts. This problem can be resolved by adding calcium octoate or zirconium octoate to the formula.
Poor Cure Response (Catalyst/Pigment Interaction)
A simple procedure to determine if the catalyst is deactivated by the pigment is to evaluate the system with and without pigment. Usually pigment absorption is assumed if a drastic decrease of cure response is observed in the pigmented coating. However, recent studies indicate other scenarios that should be considered.Certain catalysts are more susceptible to deactivation by the surface treatment of the pigment. Silica and silica treated pigments can react with 2,4-pentanedione and affect the activity of K-KAT 4205.8 This problem can be avoided by using one of the other zirconium complex catalysts that are incorporated into the isocyanate component.
Depending on storage conditions, pigments will contribute varying degrees of moisture to a paint. Often the resulting moisture content is enough to partially or completely hydrolyze and deactivate the catalyst. Again, dehydration of the system is recommended, which can be accomplished with a moisture scavenger (see Table 2). The data in the table represent a titanium dioxide pigmented acrylic crosslinked with a methyl ethyl ketoxime blocked HDI that was cured for 20 minutes at 140°C. In this case, a trimethyl orthoacetate moisture scavenger was used at 1.0% of total formula weight and the system was catalyzed with DBTDL.
Poor Pot Life/Dry Time Relationship
A constant challenge for formulators of two-component isocyanate crosslinked systems is to increase pot life without sacrificing cure response. After the two components are mixed, there is a limited amount of time before the material will develop a viscosity that prohibits proper application of the product. The preferred method for extending pot life will vary depending on the application. Solvent- and waterborne coatings and elastomer type applications each require different approaches to extending pot life.Volatile chelating agents can inhibit the metal catalyst from accelerating the crosslinking reaction in a container. When surface area is greatly increased by casting a film, the volatile chelating agent is released leaving the metal free to catalyze the reaction. This phenomena can occur with DBTDL, zirconium and aluminum catalysts when 2,4-pentanedione is added. It has been demonstrated that the combination of K-KAT 5218 with 2,4-pentandione provides superior pot life in solventborne two-component coatings.
The limiting factor in waterborne two-component coatings is the reaction rate of water with isocyanate. Often a large excess of isocyanate is used to ensure that maximum urethane formation is achieved. Inevitably urea will be formed contributing hardness and brittleness to cured films. Pot life is usually determined by the rate of urea formation rather than viscosity increase. As the paint is aged, formation of insoluble ureas will cause phase separation and lower gloss of cast films. Therefore, the pot life of a waterborne two-component system can be extended by increasing the rate of urethane formation relative to urea formation. This can be accomplished with a selective catalyst that will more efficiently accelerate urethane formation by way of the isocyanate/polyol hydroxyl reaction. Isocyanate content can potentially be reduced when a selective catalyst is used. As discussed in the “low gloss” section, the zirconium complex catalysts are recommended. Each of the K-KAT zirconium catalysts can be considered except for K-KAT 4205. The amount of 2,4-pentandione in K-KAT 4205 prohibits its use in waterborne coatings.
Increasing the pot life of a 100%-solids elastomer continues to be a challenge. Theories have been proposed, including the use of catalyst blends, to simulate the catalytic activity of mercury compounds in these systems. Certain mercury compounds have the unique characteristic of providing an initial period of latentcy followed by rapid catalysis. However, the toxicity of mercury compounds is a major concern.
Along these lines, recent studies have revealed some interesting characteristics of bismuth. In some polymer systems the hydrolysis reaction of the K-KAT 348 catalyst is reversible and can be used to achieve a delayed reaction. K-KAT 348 was added to a polyester elastomer and blended with methylene diphenyl diisocyanate (MDI). Water was added to the system to promote hydrolysis of the catalyst. The formulation was aged for two and seven days, and a delayed reaction was observed as shown in Figure 8. In the absence of catalyst hydrolysis the formulation viscosity increased immediately. Cure response of this elastomer was measured with a durometer. The time to reach an equal maximum durometer reading was about 67% longer with the aged sample compared to the sample that was not aged. However, the time to gel (time to reach 200,000 cPs) was approximately 10 times longer with the aged sample, suggesting that the active carboxylate is reformed after a period of deactivation.
Yellowing
Yellowing with metal catalysts may be caused by the chelation of the metal compound with benzotriazole UV absorbers. The aluminum catalyst, K-KAT 5218 is most affected by this interaction and zirconium the least. The increased tendency to interact with benzotriazole is likely due to the higher metal content required with K-KAT 5218. If a UV stablizer is required, and yellowing is noticed prior to UV exposure, a triazine type UV absorber such as TINUVIN 4009 is recommended. Yellowing can also develop in systems that include 2,4-pentanedione in the presence of amines or silica. Tertiary amines are less likely to cause a problem than primary and secondary amines.
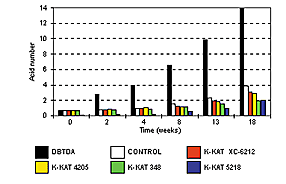
Poor Exterior Durability
Residual catalyst from the NCO/OH reaction can potentially catalyze the hydrolysis of ester groups. Exterior durability will decrease as the polymer film degrades due to ester group hydrolysis. A method used to examine the affect of catalyst on ester hydrolysis involved monitoring the acid value of a homogeneous aqueous polyester polyol solution. The solution consisted of 60% of a low molecular weight polyester polyol (OH number = 240), 30% methyl ethyl ketone and 10% water. The catalyzed solutions (0.01% metal on TRS) were stored at 50°C for 18 weeks and acid values were monitored weekly. In this case the more hydrophilic DBTDA was used instead of DBTDL to assure a homogeneous solution. Figure 9 illustrates increased hydrolysis of the polyester with a marked increase in acid value of the solution containing the tin catalyst.
Poor Cure of Cationic Electrocoating and Thermoset Waterborne Coatings
Bismuth carboxylate catalysts, including K-KAT 348 and K-KAT XC-8203, will initially hydrolyze when added to a waterborne coating. In this case, as with the 100%-solids elastomer, reforming the active catalyst plays a beneficial role in the catalysis of these blocked isocyanate systems. However, the path to hydrolysis is critical. In waterborne coatings the incorporation method is essential to the activity of this catalyst, requiring that the catalyst must be added to the polymer before water addition. The water should be added slowly under high speed agitation. During water addition the bismuth carboxylate slowly hydrolyzes forming a nano particle dispersion of bismuth oxide/hydroxide. During cure the bismuth oxide/hydroxide reverts to the catalytic form.Factors Affecting Catalyst Selection and Performance
The following charts were designed to assist formulators in troubleshooting metal catalyzed urethane problems. Table 3 is based on factors that can lead to catalyst deactivation.Table 4 lists possible causes and provides suggestions for common problems from a catalyst perspective.
Conclusion
With the growing choices of metal catalysts for NCO/OH reactions, it is important to understand that all catalysts are not created equal. Each catalyst can contribute unique characteristics to a coating or elastomer system. The expanding list of potential catalysts allows the formulator new opportunities to solve curing problems from a catalyst perspective. Rather than adjusting a resin system or changing environmental conditions, a formulator can change a catalyst, for example, to reduce gassing when conditions are humid. It is more practical to use a catalyst that will provide a pot life of an entire work shift rather than to absorb the cost of lost man hours and materials using a paint with a short pot life. A consequence of having a variety of catalysts to select from is the inherent selection process that is more involved. However, in the long run, with proper selection guidelines and troubleshooting techniques, the final product can become more cost efficient, easier to handle and have superior properties.Acknowledgements
The author would like to acknowledge Werner Blank and Dr. Leonard Calbo for their contributions to this article.For more information on urethane catalysis, contact King Industries Inc., Science Road, Norwalk, CT 06852; phone 203/866.5551; fax 203/866.1268; e-mail coatings@kingindustries.com.
Looking for a reprint of this article?
From high-res PDFs to custom plaques, order your copy today!