Effect of Coating Composition on UV-Curable Powder Coatings

UV-Curable Powder Coatings
The references describe several examples of binder systems suitable for UV-curable powder coatings, including unsaturated polyacrylates1, mixtures of unsaturated polyesters and acrylates2, methacrylated polyesters3, acrylated hyper branched polyestersiv and specially formulated solid epoxy resinsv. DSM Coating Resins has developed a binder system based on a blend of an unsaturated polyester and a vinyl ether urethane component.6,7 The polymerization mechanism of the latter system proceeds via formation of a maleate-vinyl ether (MA-VE) donor-acceptor complex followed by radical homopolymerization of this complex yielding a 1:1 alternated MAVE copolymer.8
Like conventional powder coatings, the UV powder coating formulation is processed by extrusion. Due to the semi-crystalline nature the extrusion temperature may not exceed 70 deg C, and the extrudate temperature may not be higher than 75 deg C. This temperature is above the glass transition temperature, but below the melting range of the crystalline regions. Above the melting temperature the melting of the crystalline regions leads to a dramatic reduction in viscosity, which makes extrusion virtually impossible.
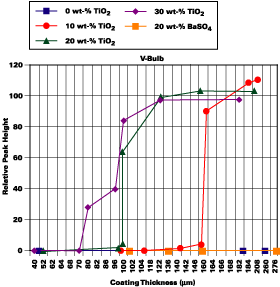
For the application of UV powder coatings on MDF tribo charging is very suitable, but also corona can be used. These techniques are well described in literature.9 For poorly conducting materials, the substrate is sometimes preheated to improve the uniformity in the powder layer thickness.10
The curing of the applied powder coating occurs in two steps (see Figure 1). In the first step the powder coating particles are heated by IR radiation. This method heats the surface very efficiently, while the temperature of the substrate will stay low.
In practice the IR power is controlled via a pyrometer that measures the temperature of the surface and is set on a maximum of 100 deg C in the case of MDF. During the melting of the powder the particles will flow together to form a continuous and smooth film. Depending on the substrate and the heating power, the whole process of melting and flowing takes 1 to 3 minutes.


Effect of Pigmentation
For UV powder coatings the optical process determines to a large extent the curing of the film. In an earlier publication optical processes in UV-curable powder coatings were very well described in terms of reflection, scattering (forward or backward), absorption, and transmission.11 The pigment, filler and binder system play a role in these processes. In earlier studies we examined the effect of the UV dose and lamp type for a clear coating and a 20 wt% pigmented system.12 In this paper the effect of pigmentation is studied in more detail to reveal where the limits of cure are for UV-curable powder coatings. The coating formulations used for this study are shown in Table 1.In the formulations only the pigment concentration is varied in order to study the effect of these on the coating properties. Also a formulation containing only filler (BaSO4) was used in this study. Two different UV-curing conditions were applied using the H-bulb (mercury type of lamp) and the V-bulb (gallium doped lamp).

ATR-FTIR
To study the efficiency of UV-curing, ATR(Attenuated Total Reflection)-FTIR was used. The ATR unit consists of two 45? fixed incident angles and a horizontal plate with a Silicium crystal. A full description of this technique can be found in several literature references.13 The FTIR system is a Biorad FTS-60 configured with a liquid nitrogen cooled MCT detector. In these measurements the backside of the coatings was placed on the crystal with a pressure of 1 kg. The coatings were analyzed at 4 cm-1 resolution and averaged over 16 scans. The vinyl ether peak at 1,648cm-1 was used for analysis of throughcure. The vinyl ether peak was normalized with respect to a peak with constant value in the spectra at 1,018cm-1. In this way the relative amount of vinyl ether, on the coating backside, was studied with respect to coating thickness. This study was done for the H-bulb (mercury type of UV-lamp) and the V-bulb (gallium doped type of UV-lamp). The results are shown in Figures 2-3.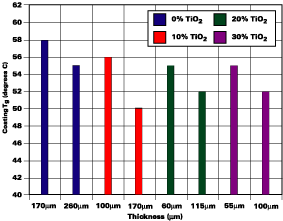
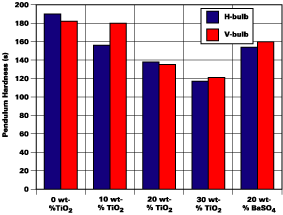
UV Transmission Measurements
For proper cure throughout the coating there is a certain amount of UV-light required to reach the coating-substrate interface. The UV-light reaching the bottom of the film can be the result of transmission or forward scattering. To determine this amount of UV-light, a cured film under a quartz plate was placed on the eye of the Power Puck PP2000. The amount of transmitted light is calculated with respect to the amount of UV light measured by the power puck with only the quartz plate on the eye. The results of the total amount of transmitted light for several coatings of varying thickness are shown in Figure 4 for the H-bulb, and in Figure 5 for the V-bulb.Figure 4 shows that only for the clear coating and the coating with 20 wt% BaSO4 a substantial amount of UV light is transmitted through the coating. For the coating with TiO2 the transmission as measured with the Power Puck is very limited. The detection limit of the Power Puck is around 50 mJ/cm2. From the ATR-FTIR measurements it was found that no residual double bonds were found for coatings at 10 wt% TiO2 at 68?m thickness, 20 wt% TiO2 at 53?m thickness, and 30 wt% and 50?m thickness. It can be concluded that limited transmission does not necessarily mean a bad throughcure of the coating. A very low amount of UV light of less than 50 mJ/cm2 is sufficient to cure the coating at the bottom. The same picture is presented in Figure 5 for the V-bulb. The amount of transmitted light is higher compared to the H-bulb. This can be expected, since the V-bulb is a gallium-doped lamp, which emits longer wavelength UV light. This UV light is able to penetrate better through the coating. At the low film thickness at 10 wt% and 20 wt% TiO2 still some UV light is transmitted. At the other films, at higher thickness and higher TiO2 levels, also limited transmission was found.
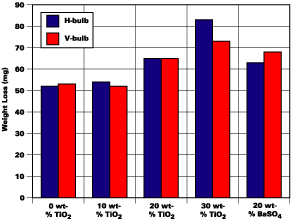
Glass-Transition Measurements
The effect of coating thickness and lamp type on the coating glass transition temperature was studied. The measurements were done using Modulated DSC (MDSC) at 1 deg C per minute heating rate in heat only mode. Theoretical details about MDSC can be found in the references.14 In Figure 6 the effect of coating glass transition temperature with respect to pigmentation with TiO2 at different layer thickness can be found. In case of good UV curing (see columns with shading) a mean glass transition temperature of 55 deg C with a standard deviation of 1 deg C was found. The coatings with which double bonds of the vinyl ether were found on the backside of the coating (dotted columns) show a clear lower glass transition temperature. For the V-bulb, shown in Figure 7, the same trends were observed. The results for the H-bulb and V-bulb were comparable.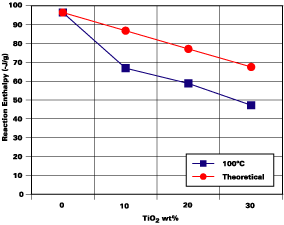
Coating Properties
Two coating properties were evaluated in relation to the ATR-FTIR results: transmission measurements and glass-transition temperature. The effect of pigmentation on pendulum hardness can be found in Figure 8. The hardness measurements were done on MDF at a coating thickness of approximately 120Km, and is averaged over three measurements. A clear decrease was found for increasing pigmentation level. From the ATR-FTIR measurements the curing at 10 wt% TiO2 and 120?m coating thickness should be comparable and complete for both light sources. The decrease from 0% to 10 wt% TiO2 is larger for the H-bulb, than for the V-bulb.The coating filled with BaSO4 is better in hardness than the equivalent at 20 wt% TiO2. This could be expected from ATR-FTIR and transmission measurements, due to worse throughcure and transmission for the coating with 20 wt% TiO2.
The effect of pigmentation on abrasion resistance can be found in Figure 9. The abrasion resistance15 was averaged over two measurements with a Taber abraser with CS 17 wheels with a load of 1kg on glass panels and layer thickness of approximately 100?m. The results indicate a decrease in abrasion resistance at a pigment level of 20 wt% TiO2 and higher. The results of the clear coating and 10 wt% TiO2 are comparable. The differences between 20 wt% TiO2 and 20 wt-% BaSO4 are small, also when the different light sources are compared. It can be concluded that the pigment or filler is determining the abrasion resistance, and that this is not directly related to the penetration of UV-light or cured coating Tg.
In general the abrasion resistance of these UV-curable powder coatings can be marked as good.
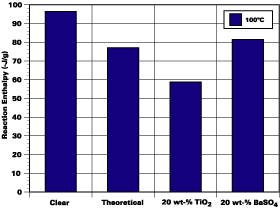
Differential Photocalorimetry
Differential scanning calorimetry was used to study the UV-curing of the formulations. The layout of a differential scanning calorimeter can be found in literature.16 The DPC used is a DPA-7 from Perkin Elmer equipped with a 450W Xenon UV-lamp. Isothermal measurements were performed at 120 deg C under nitrogen, and the average value of three measurements is reported.The results for UV curing at 100 deg C expressed in reaction enthalpy as a function of the TiO2 content can be found in Figure 10 together with a theoretical calculation. In the (theoretical) calculated line it is assumed that the curing of the clear coating reaches full conversion, and that with increasing TiO2 content the enthalpy decreases proportional with the resin content. A decrease in reaction enthalpy was found with curing at 100 deg C with increasing TiO2 content. This decrease is not completely due to the fact that binder is replaced by pigment, as can be seen from the theoretical line. Two other factors reducing the enthalpy are competition between the pigment and the photoinitiator regarding UV absorption and a possible effect of viscosity. Remarkable is the fact that the difference between the theoretical line and the results found is almost constant around 20 J/g. An increase of the difference with increasing pigment amount was expected, since an increasing pigment level would lead to an increase in UV absorption by the pigment.
The comparison between the coating with 20wt% TiO2, 20 wt% BaSO4 and the clear coating can be found in Figure 11. Also the theoretical calculation was included, which is a correction of the value of the clear coating for the decrease in binder content due to pigmentation. The value of the coating with 20 wt% BaSO4 corresponds with the theoretical value. The value of 20 wt% TiO2 is clearly lower. This result is in line with the ATR-FTIR measurement, where the coating with BaSO4 showed no vinyl ether double bonds at the backside of the coating, even at high film thickness.
Conclusion
Several methods are presented to evaluate the UV curing of powder coatings. ATR-FTIR gives information about the relative amount of double bonds present in the cured coating. After a study of the backside of the coatings a clear relation was found between coating thickness and curing performance. The difference between the H-bulb and the V-bulb at lamp power 144 W/cm was only found at 10 wt% TiO2. At higher pigmentation level the differences are small. The limits for complete cure with regard to coating thickness found are >250Km for clear and 20 wt% BaSO4, 140-150Km for 10 wt% TiO2, 80Km for 20 wt% TiO2, and 70Km for 30 wt% TiO2.Transmission measurements can also be used to evaluate throughcure, although very limited penetration of UV light does not necessarily mean incomplete throughcure. The V-bulb has a higher transmission as was expected due to the longer wavelength emission of this lamp.
Glass transition temperature measurements also provide a good measure of curing. A mean glass transition temperature of 55 deg C with a standard deviation of 1 deg C was found for well-cured films. With coatings of higher thickness or higher pigmentation level a clear decrease in coating glass transition temperature was found. No large difference between the H-bulb and the V-bulb was found with respect to the coating glass transition temperature.
Coating properties like pendulum hardness and abrasion resistance clearly relate to the amount of pigment used. At 20 wt% TiO2 level and higher a clear decrease in properties was found. No direct relation was found between throughcure and abrasion resistance.
In the differential photo calorimetry measurements a clear decrease in reaction enthalpy was found. The differences between a theoretical calculation from the clear coating and the measured values were found constant for all pigment levels.
Methods like ATR-FTIR, transmission, coating glass transition measurements and differential photo calorimetry can be very useful for interpretation of the curing of UV-curable powder coatings.
For more information on resins, contact DSM Coating Resins B.V., Ceintuurbaan 5, 8022 AW Zwolle, PO Box 615, 8000 AP Zwolle, The Netherlands; visit www.dsmcoatingresins.com; or Circle Number 126.
Acknowledgement
The authors would like to thank Sandra van der Flier and Wim Kaptijn for providing help in doing part of the tests described in this article.
This article is based on a paper presented at RadTech 2001 Conference in Basel, Switzerland.
References
1 a. BASF EPA 650978, 650979 (1994), and 678562 (1995); b. Bayer EPA 350730 (1990)2 Hoechst EPA 585742 (1994)
3 a. Zune, C.; Buysens, K. Eur. Coat. J. 2000, 20-30; b. UCB EPA 739922 (1995), WO 98/18862 and 98/18874
4 Hult, A.; Johansson, M.; Jansson, A.; MalmstrEm, E. Proceedings RadTech Berlin 1999, 634-639
5 a. Reisinger, M. Procedings RadTech Berlin 1999, 628-633; b. Ciba-Geigy EPA 667381 (1994)
6 DSM Coating Resins EPA 636669 (1995)
7 For more information see www.dsmcoatingresins.nl
8 a. Zahora, E.P.; Lapin, S.C.; Noren, G.K.; Wehman, E. Modern Paint Coat. 1994(Oct), 120-124; b. Lee, C.; Hall, H.K. Macromolecules 1989, 22, 21-25
9 Misev, T. Powder Coatings, Chemistry and Technology. John Wiley & Sons, N.Y. 1991, 324-361
10 Karlsson, L. Proceedings Powder Coating Europe 2000, 47-57
11 Jahn, R.; Lahaye, J.; Laver, H.; Schmid, O.; Walvaart, C. te. RADNews Issue 33, summer 2000, 16-21
12 Baijards, R.A.; Antonisse, M.M.G.; Udding-Louwrier, S. Proceedings of the Radcure Coatings and Inks: Formulation & Performance, PRA; Harogate, 2000, Paper 15, 1-8
13 Coleman, P.B. Practical Sampling Techniques for IR Analysis (1993) CRC Press Inc. Krishnan, K.; Ferraro, J.R.; Fourier Transform Infrared Spectroscopy, Vol.3, Ch.5, Academic Orlando 1985
14 Modulated DSC Compendium. Basic Theory & Experimental Considerations. TA Instruments 1996
15 ASTM D 4060 - 95. Standard Test Method for Abrasion Resistance of Organic Coatings by the Taber Abraser.
16 Rabek, J.F.; Fouassier, J.P.; Radiation curing in polymer science and technology. Volume 1. Fundamentals and Methods. Chapter 7. Elsevier Science Publishers, London and N.Y. 1993
Links
Looking for a reprint of this article?
From high-res PDFs to custom plaques, order your copy today!