Factors of Weather and Climate
“Why Test?”
This paraphrased question was asked in a marketing survey several years ago to determine why companies sent specimens to independent testing laboratories and/or purchased artificial weathering equipment. The results were not surprising. Nearly two-thirds of those surveyed indicated that they test simply “because we have to.” This means that, to sell their material, they were required by their buyer to prove the performance of their material upon exposure to outdoor conditions. Most of this testing is done to exacting corporate, industry or government specifications. Without data to support the performance of a new formulation or process, the buyer simply would not agree to purchase the material. This demonstrates the importance of test standards and specifications, and also underlines the importance of understanding how to properly conduct these tests. Other responses to this survey included the need to compare materials to competitive products, which may provide data for sales and marketing campaigns for a company. While almost all testing is in some way related to research and development, about 10% of the testing done was strictly for these purposes, while another 10% tested to ensure that their material would meet the warranty claims, or other requirements.
Answers Expected from Weathering Tests
By understanding weathering test applications and evaluation techniques, one should be able to accurately rank materials by performance. In other words, “Is this new formulation/process better than the old one?” in terms of durability, cost, etc. This ranking technique is commonly performed by comparing a new formulation to a “known” or control material. These are materials that have been tested and characterized so that performance is well known and documented. This can include standard reference materials developed by an industry, such as polycarbonate reference chips or blue wool materials, or it may be an old formulation of a specific material developed internally by an organization.In research work, testing may allow one to understand and identify the likely failure mechanisms that occur as a result of weathering. Understanding these processes may lead material scientists to develop new formulations and processes that inhibit these mechanisms. While new formulations may be the primary factor for testing, new surface preparation methods or cost saving processes may also be evaluated during a weathering test. For all accelerated testing, the primary concern is how well the test compares to what happens in the actual end use environment of a material. This is often called a correlation factor. This factor relates very closely with predicting service life, which is the ultimate goal of any weathering test. Unfortunately, no “universal” correlation factor exists for all materials, and most experts agree that correlation (or acceleration) factors can only be determined empirically.
The three main factors of weather that cause degradation to materials are light energy (solar radiation), water and temperature. However, it must be understood that is not just a matter of “how much” light, “how much” moisture, and “how much” temperature is required to break down a material. In fact, the quality of the light (or light source) is an extremely important factor in the design and evaluation of weathering tests. Water contact may be in several forms or phases, and these different phases can contribute to different degradation processes. While the temperature of a material can significantly influence the rate of degradation, temperature cycling can cause mechanical stress, particularly in composite systems, which may cause more types of degradation than just the long durations of high temperatures. The synergistic effects of temperature with moisture and radiation make the study and predictability of weathering tests extremely difficult.
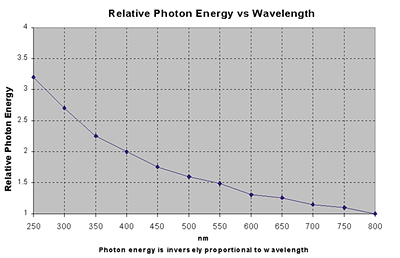
Light (Radiant Energy)
Terrestrial daylight is commonly separated into three main wavelength bands. According to Commission Internationale E’clairage (CIE) Publication 85, daylight nominally consists of wavelengths of energy between 295 and 3,000 nanometers (1 x 10-9 meters). Wavelengths between 295 and 385 nm are considered the ultraviolet (UV) region of the solar spectrum, making up between 4% and 6% of the total energy. It is common practice to further separate the UV region of the spectrum into the UV-A (between 315 and 385 nm), and the UV-B (between 280 and 315 nm) areas. Ozone absorption in the stratosphere eliminates all radiant energy below 295 nm. Visible radiation (the energy the human eye can detect) is between 385 and 780 nm, making up approximately 45% of the solar spectrum. Nearly half of the energy from the sun is contained in the near-infrared (N-IR) portion of the solar spectrum beyond 780 nm.The cut-on wavelength of terrestrial sunlight and the percentages of each wavelength band reported will vary slightly depending on latitude, altitude, and atmospheric conditions. Also, the defined break between the UV and visible portions of the spectrum may be different depending on the source of the information. Some consider the UV portion of the spectrum to be between 295 and 400 nm. While this may be considered a trivial point, it must be understood when calculating radiant dosages for exposure, whether in outdoor or artificial conditions. The variance between the 295-385 range and 295-400 may be as much as 10%, which could be extremely important when attempting to predict service life estimations for a material.
Photon energy increases with decreasing wavelengths of solar energy (see Figure 1). These shorter wavelengths may contain enough energy to break chemical bonds in the polymeric structure of a coating. As the chemical structures change, the appearance and physical properties of a material will change, resulting in physical or chemical degradation. This explains why the higher energies contained in the UV portion of the spectrum are the most important in our discussion of material degradation. While the study of photochemistry is in itself a complex subject, there are two important laws that must be remembered.
1. A material may only be affected by the wavelengths of radiation it will absorb, and
2. Photochemical changes can only occur if the radiant energy absorbed is great enough to break (or alter) chemical bonds.
These concepts serve as the foundation for understanding the photochemical processes that occur as a material is being weathered and, more importantly, how to protect materials from these processes.

Direct and Diffuse Radiation
Direct radiation is radiant energy that reaches the earth’s surface without being scattered by any component of the atmosphere. For radiometry measurements, this is defined as radiant energy within a 6º field of view of the sun itself. Diffuse radiant energy is radiation that has been scattered by the atmosphere, and therefore reaches exposed surfaces at all angles (other than that defined as direct) above a 180º plane. It can therefore be assumed that for an exposed horizontal surface, both direct and diffuse solar radiation is received. This is sometimes referred to as total, or global, solar radiation. As will be discussed, it is common to expose specimens in natural conditions with some kind of tilt, which will increase solar radiation received, or better simulate end-use conditions. Under these conditions, the surface of a specimen still receives the direct and diffuse radiation as discussed previously. In addition, there is that radiation that reflects off the earth’s surface (sometimes referred to as albedo radiation) that also reaches the surface of a specimen. The amount of radiation reflected off the earth’s surface is dependent upon the ground covering. Bare rocks, sand or gravel will reflect much more radiant energy than a grass-covered surface. Water or snow will reflect an even greater amount of radiant energy. The ratio between direct and diffuse energy reaching the earth’s surface is strongly influenced by atmospheric conditions. Water vapor (humidity) and pollution will increase the amount of radiant energy found in the diffuse component. Figure 2 depicts the amount of direct radiant energy measured at three locations. Note that the desert location of Phoenix has a much higher percentage of radiant energy in the direct component. This is due to the fact that there is much less humidity in the desert than in a subtropical environment such as south Florida. By contrast, for a site with higher levels of pollution such as Los Angeles, the amount of direct radiant energy dramatically drops. Also shown in this table is the difference in direct component between total solar radiation (including all regions of the solar spectrum) and only the UV region. Shorter wavelengths of energy are more likely to be scattered. Therefore, the percentage of UV in the direct component will always be less than the total solar radiation.

Figure 3 shows a portion of the radiometer rack at DSET Laboratories in Phoenix. There are two types of radiometers shown to measure solar radiation. Those that measure total solar radiation (UV, visible, and IR) are called pyranometers. They measure radiation in a 180º field of view. The other type of radiometer shown is a total ultraviolet radiometer (TUVR). Because of the direct and diffuse components, different exposure angles will receive varying amounts of radiant energy. Therefore, radiation is commonly measured at angles that correspond to common angles of exposure.
While discussing the measurement of radiant energy, it is necessary to define some terms used in measuring and calculating radiant energy dosages received by specimens on exposure. The radiometers shown in Figure 3 are used to measure instantaneous levels of radiation received, called irradiance. Irradiance can be defined as the amount of radiant flux striking a defined surface area, commonly expressed in W/m2. For this parameter, it is necessary to indicate the spectral range in which the measurements were taken or for which the values were calculated, such as 295-3000 nm (total solar) or 295-385 nm (total UV). If we turn our attention to narrow wavelength intervals, then we obtain the spectral irradiance, measured in W/m2•nm. For weathering tests, the concept of radiant exposure, which is the time integral of (spectral) irradiance, may be more important, and is stated in J/m2. Most radiant exposure dosages are measured in either kJ/m2 or MJ/m2. Another unit of measurement, the langley, is sometimes used. It can only be applied to total solar radiation and not artificial light sources. A langley is equal to one gram-calorie per square centimeter One langley is equal to 0.04184 MJ/m2 (295-3000nm). By definition, a “langley of UV” does not exist.
Water (Moisture)
Moisture in different phases can become deposited or incorporated in a coating on exposure. Moisture can take the form of humidity, dew, rain, snow, frost or hail, depending on the ambient temperature. Moisture can have both physical and/or chemical effects on materials.Water absorption by synthetic materials and coatings from humidity and direct wetness is a diffusion controlled process. This hydration of the surface layers produces a volume expansion, which places mechanical stress on the dry subsurface layers. A subsequent drying out period signifies a desorption of water. The drying out of the surface layers would lead to a volume contraction; the hydrated inner layers resist this contraction, leading to surface stress cracking. This oscillation between hydrated and dehydrated states may result in stress fractures. Because of diffusion rates in organic materials, it may take weeks or months to reach moisture equilibrium.
The freeze-thaw cycle may cause other physical effects. Because water expands when it freezes, absorbed moisture in a material, especially coated hardboard systems, causes expansion and stresses that cause peeling, cracking, and flaking in the coating. Rain, which periodically washes dirt and pollutants from the surface, has an effect upon the long-term rate of deterioration that is determined by its frequency. When rain strikes an exposed surface, the evaporative processes cool the surface of a specimen down rapidly, which may cause further physical degradation to a material. Frozen rain, or hail, may also cause physical degradation to coatings because of the strong kinetic energy associated with its impact.
Moisture, as H+ and OH-, is important in weathering from several points of view. The chemical effects of moisture can be seen in the chalking of titanium dioxide (TiO2) pigmented coatings and polymers. The structure of a polymer is changed photochemically, either with or without chemical participation of hydrogen or hydroxide ions, but the actual physical release of material on the surface is enhanced, if not caused, by the cyclic action of chemically absorbed moisture. Moisture may also act as a pH adjuster, especially when considering the effects of acid rain, which may cause an etching of many coatings.
Temperature
The temperature of materials exposed to solar radiation has a significant influence on the effect of the radiation. The destructive effects of light are usually accelerated at elevated temperatures as a result of the increased rate of secondary reactions because reaction rates tend to double with each 10ºC rise in temperature. This may not be true of all materials and may not be seen when measuring physical or appearance changes. At high temperatures molecules have greater mobility. Reactions may take place at higher temperatures that occur at a very low rate or not at all at lower temperatures.In the presence of sunlight, the surface temperature of an object is usually considerably higher than the temperature of the air. Solar absorptivity is closely related to color, varying from about 20% for white materials to 90% for black materials; thus samples of different colors will reach different on-exposure temperatures. Because the thermal conductivity and heat capacity of plastic substrates are generally low, much higher temperatures can be obtained on the painted or coated surface than in the bulk of the material. Therefore, both the surface temperatures of the sample, produced largely by infrared radiation absorption which varies by material color, and ambient air temperature and its fluctuations during exposure do play a role.
Diurnal and seasonal variations occur in solar radiation. Temperature cycling can cause mechanical stress, particularly in composite systems consisting of materials with widely differing temperature coefficients of expansion. Temperature and its cycles are also closely linked with water in all of its forms. Drops in temperature can cause water to condense on the material as dew, a rise in temperature causes evaporation, and sudden rainfall can cause thermal stress. Finally, the combination of heat and absorbed solar radiation may cause constituents in a polymer (such as a plasticizer) to volatilize out of the system. One example of this relates to the exposure of instrument panel systems. The vinyl skin covering an instrument panel contains plasticizers that make the polymer pliable and bendable around the shape of the panel. As a result of exposure, this additive may exude out from the polymer and be deposited on the cover glass of the exposure box. In time, this volatilization process causes the vinyl skin to become brittle. Extreme temperature cycling may result in physical degradation such as cracking.
The important fact to understand when considering the roles that light, moisture and temperature play on an exposed specimen is that these factors work together to degrade materials. For example, if a material is exposed only to one of these factors, it is very unlikely that the degradation incurred will look anything like that of a material exposed to outdoor conditions, where all three of these factors play a role in the degradation processes.
One must not underestimate the secondary effects of weather or the atmosphere that may cause degradation. Gases and pollutants in the atmosphere, especially in the form of acid rain, may cause entirely new reactions to be initiated. In highly industrialized areas, where long range transport of airborne acidic particles is not a consideration, acid rain is the primary element driving the weathering process affecting a wide range of materials. Dirt and dust may have non-interactive effects on the weathering process itself. These include the screening of ultraviolet radiation from the materials by ultraviolet-absorbing dirt, airborne grease, etc., and undesirable, semi-permanent “varnishes” that may be photo-polymerized on the surface of exposed materials. Mold, mildew and other microbiological and botanical agents may play a significant role in material degradation, particularly in tropical and subtropical climates, although they may not be generally thought of as weathering factors. Acts of nature may not directly cause weathering processes to occur. However, acts of nature such as El Niño, La Niña and volcanism may affect climatic conditions, which in turn result in different degradation rates.
Climate
In viewing a climatological map of the world (see Figure 4), it is obvious that depending on latitude, weather patterns, topographical and geographical features, etc. a wide range of climates exist. The World Meteorological Organization (WMO) has identified seven major climates around the world. The two most important climates (or benchmarks) for weathering tests, are the subtropical environment, such as south Florida, and the desert environment, such as northern Mexico and the southwest United States. Other climates, such as polar, tropical rain forests, humid meso thermal, humid micro thermal, and undifferentiated highlands, are sometimes used as weathering test sites for specific applications. In most cases, the subtropical and desert environments are recognized around the world as the most severe climates for materials exposed outdoors in their expected end-use application.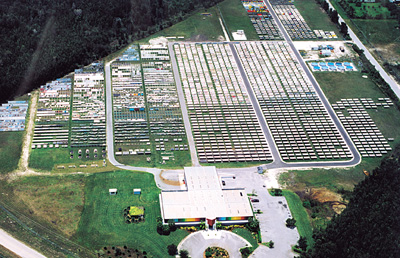
“Why Test In These Benchmark Climates?”
Exposures in the moist, subtropical climate of south Florida (see Figure 5) were first conducted in the 1920s and 1930s as the paint industry became more concerned about the weathering resistance of their products, making this area an important historical outdoor testing region. The largest independent test sites are located in areas that are considered an inland location, and therefore do not see any adverse corrosive coastal effects.
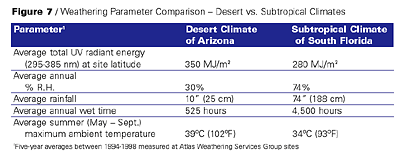

Figure 8 shows the average annual radiant energy received at common exposure angles in Florida and Arizona. Although higher amounts of UV radiant energy are received by exposures in Arizona, one should not assume that Arizona is a harsher climate to expose materials, because of the dramatic increase in moisture received in south Florida. This addition of moisture has shown to be very effective in the degradation of coatings and, in general, more coatings are tested to this environment than the desert of Arizona. However, the dry climate of Arizona, in conjunction with the dramatic diurnal temperature cycling, has proven to be very effective in the degradation of interior automotive materials and many other polymers. It is common for many companies to test their materials in both the subtropical and desert climates (and others, for that matter) because the effects of these extremes will result in different types of degradation processes.
Weathering test fields are required to monitor and store all major climatological data. The enclosure used to house the ambient temperature and relative humidity devices is specified by WMO. The enclosure must have a double roof; in other words, there is essentially two pieces of wood over the top of the enclosure, with a small air space between. This is to inhibit any direct radiant energy from striking the roof of the enclosure. The enclosure must also be naturally aspirated to allow wind to flow through the housing. Also, the enclosure must be mounted a specific height above the ground so the reflected solar radiation does not affect either the ambient temperature or relative humidity. Wind speed and wind direction sensors, relative humidity sensors, rain gauges, wet time sensors, and special radiometric devices are all connected to a data acquisition system for continuous monitoring of the major weather parameters.
It is widely accepted that black panel temperature devices are used to control temperatures inside artificial weathering instruments. To study some kind of correlation to temperatures reached in outdoor exposures, black panel (and white panel) temperatures are also commonly measured. Because of the high solar absorptance of the black paint, these devices are used as a reference temperature, that may be thought of as the highest temperature that specimens on exposure may reach. These panels are usually thin metal (stainless steel or anodized aluminum) and are coated with a primer and a quality black paint. Standards organizations are in the process of writing a specification to define the properties of the paint, as well as developing methods to monitor and attach temperature measuring devices to the panel.
Acknowledgments
While much of the information is based on first-hand experience of the author, the concepts presented closely follow work done by other experts in the field of material degradation and weathering. I would like to acknowledge the following associates for their invaluable contributions to this script.For more information on weathering tests, contact Atlas Electric Devices Co., 4114 N. Ravenswood Ave., Chicago, IL 60613; phone 773/327.4520; fax 773/327.5787; www.atlas-mts.com;
e-mail mmcgreer@atlas-mts.com.
Sidebar: Atlas Material Testing Solutions
Atlas Material Testing Solutions offers “solutions” to testing needs by manufacturing several types of testing devices to meet a variety of testing applications, and to providing services for independent material testing. Atlas can be separated into two groups: the manufacturing, or Test Instruments Group, and the Weathering Services Group. The Test Instruments Group has three locations where various testing devices are developed and manufactured.Atlas Electric Devices Co. is located in Chicago and serves as the corporate headquarters for the company. Testing devices such as the Weather-Ometer, Launder-Ometer and UVCON are manufactured there. Xenotest GmbH, located in Linsingericht-Altenhauslau, Germany, is the manufacturing location for air-cooled xenon-arc weathering devices such as the Alpha and Beta, and the smaller units such as the Suntest CPS and Suntest XLS. K.H. Steurnagel is another entity of Atlas Material Testing Solutions. This division develops both high-speed lighting equipment and full-scale solar simulation devices.
The Atlas Weathering Services Group (AWSG) has three main locations where specimens are exposed to outdoor weathering conditions. The two main U.S. locations are South Florida Test Service (SFTS), located in Miami, and DSET Laboratories, located 30 km north of Phoenix. Other non-staffed sites across the United States are also controlled and administered by the AWSG. Atlas MTT operates a benchmark northern European location in Lochem, The Netherlands. An indoor accelerated laboratory is operated near Duisburg, Germany. Several other outdoor weathering sites are controlled by Atlas MTT in Europe.
The Test Instruments Group has recently been separated into four manufacturing divisions. The Weathering Instrument Products Division develops all devices used for artificial accelerated weathering of materials except for the full-scale and metal halide units. This includes units using light sources such as the carbon-arc, fluorescent, and xenon-arc devices. The Textile Testing Products Division, as the name implies, develops the devices used for testing textile products, primarily for colorfastness. This includes instruments such as the Launder-Ometer, which tests for wash fastness; the crockmeter, Universal Wear Tester, Accelerotor, Bean Bag Snag Tester, and Random Tumble Pilling tester, which test fabrics’ resistance to wear; and several other textiles laboratory instruments. The Polymer Evaluation Products Division manufactures instruments designed to test plastic materials, using several widely known methods, such as heat distortion, melt flow, impact, and flammability. The Analytical Division, located in Germany, manufactures products such as the Chemilume and VIEEW, which are used to evaluate materials during or after weathering. These are sophisticated instruments that are technically more advanced than commonly used evaluation methods, but have been shown to accurately determine rates of degradation and objectively evaluate surface defects.
Links
Looking for a reprint of this article?
From high-res PDFs to custom plaques, order your copy today!