Crosslinking Technology for Fast-Curing, High-Performance, Low-VOC Coatings

With the variety of crosslinking methods and chemistries available today, coatings manufacturers should scrutinize their processes to ensure they are using the optimal method for their application. Selecting the right crosslinking technology empowers the manufacturer to improve operational efficiency, reduce production costs and manipulate the finished coating's characteristics.
Crosslinking, or curing, is the process during which a multifunctional molecule participates in polymerization and is incorporated into polymer chains. Typical methods of crosslinking include: air-drying, condensation reactions, free radical curing (such as ultraviolet/electron beam or peroxides), and amine curing using Michael Addition reaction chemistry. Although all of these methods will ultimately crosslink and successfully form a coating, only ultraviolet/electron beam (UV/EB), peroxide, and amine systems produce fast-curing, high performance, low-VOC coatings.

A Common Thread: Acrylic and Methacrylic Esters
The three highly efficient crosslinking technologies presented in this article -- UV/EB, peroxide and amine curing -- use different methods to initiate crosslinking, but all result in similar high-performance coatings. This is because all three include acrylate and methacrylate monomers and oligomers in the coating formulation. These acrylic and methacrylic esters offer numerous improvements over conventionally formulated and cured solventborne coatings. Benefits range from faster cure times to zero VOC emissions.One of the key benefits for coatings manufacturers using UV/EB, peroxide or amine cure methods is increased speed. With acrylate monomers and oligomers, cure times are reduced to nanoseconds (in the case of UV/EB curing) or to a few minutes or hours (in the case of peroxide or Michael Addition reaction chemistry). Fast cure times translate into increased profits, because more product can be manufactured in less time.
A sense of responsibility for the natural environment is another reason many manufacturers choose coatings technologies that use acrylic and methacrylic esters. The coatings industry is known for taking a proactive stance on issues affecting public health and the environment, and pollution is no exception. Consider the industry's initiative to eliminate lead content in paint - decades before the Consumer Product Safety Commission's 1978 ban. Unlike traditional solventborne coatings that emit VOCs when the curing and solvent evaporates into the air, UV/EB-, peroxide-, and amine-cured coatings are incorporated into the cured material, keeping the air clean.
Additionally, acrylate- and methacrylate-terminated monomers and oligomers (the general structure for acrylate and methacrylate components is provided in Figures 1 and 2) provide coatings formulators with a variety of building blocks. These blocks enable them to "tailor-make" coatings systems to achieve specific properties, such as hardness, chemical resistance and flexibility. Although acrylate and methacrylate monomers differ only slightly in structure, the variation is enough to yield different performance properties. Formulators can exploit these variations, selecting from the range of available specialty (meth)acrylic esters to achieve the appropriate performance properties for almost any application.
An example of the differences between TMPTA and TMPTMA, two of the most widely used monomers in UV curing and peroxide curing, respectively, are provided in Figure 3. These differences generally hold true for most acrylate and methacrylate counterparts.
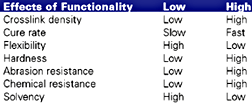
Demystifying the Components of the Crosslinking System
MonomersMonomers, the simplest building blocks found in synthetic organic materials, are generally low-viscosity acrylates and methacrylates. Monomers primarily function as reactive diluents, lowering the viscosity of the uncured coating to facilitate application. Monomer viscosities can range from 5-25,000 centipoise. They are lower in molecular weight than oligomers and vary in functionality. Mono-, di-, and tri-functional monomers, shown schematically in Figure 4, are the most common, but functionalities of six and even higher are available to formulators.
The functionality of the monomers or oligomers used in formulating high-performance coatings is only one of the factors that influences final coating performance. Monofunctional monomers (containing only one reactive group) typically act as diluents, improve flexibility, and reduce shrinkage. Multifunctional monomers (containing more than one reactive group) crosslink and also act as diluents, increase film tensile strength, enhance chemical resistance, and increase the cure rate.
The chemical nature and molecular weight of the monomers also affect the coating's performance properties. This includes the alkane vs. ether nature of the molecule as well as the linear or cyclic nature of the molecule. Each of these and their effect on coating performance are shown in Tables 2-5.
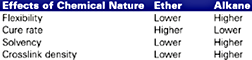
Oligomers are higher molecular weight (1,000-30,000) crosslinkable coating components used as the base material in a coatings formulation. The primary job of the oligomer is to impart the major physical properties of the finished coating.
Oligomers are based on a variety of chemistries, including acrylated urethanes, epoxies, polyesters and acrylics. This article will discuss the two major types used in coatings -- epoxy acrylates and urethane acrylates. The general structures of these types of oligomers are shown in Figure 5.

Urethane acrylate oligomers provide excellent weatherability in the case of aliphatic products, as well as abrasion resistance, scratch resistance, impact resistance and flexibility. The performance of aliphatic and aromatic products is similar to that of monomers.

A Closer Look at Free Radical, Cure-in-Place Technology
While the main components of acrylated and methacrylated systems used in UV/EB, peroxide and amine chemistry are similar (see Table 1), crosslinking initiation varies. In free-radical crosslinking, an initiator is required for monomer combination or addition to take place and for polymerization to occur. The type of initiator differs depending on the cure method (see Figure 6).Monomers and oligomers with functionality greater than one will result in crosslink formation. Figure 7 depicts a more specific reaction using a difunctional acrylate, which results in a crosslinked polymer.
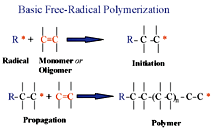
Radiation Curing
In UV-cured coating systems, a free radical-generating photoinitiator is required to initiate crosslinking of the coatings directly on the substrate. Radicals are typically produced by hydrogen abstraction upon exposure to UV light. Common photoinitiators include benzophenone, benzil dimethyl ketal, and 2-hydroxy-2methyl-1-phenyl-1-propanone. The reaction mechanism of the free radical formation by photoinitiators is shown in Figure 8.In EB curing, the coating is exposed to a stream of electrons. Sufficient energy is produced from electron bombardment to generate free radical polymerization without an initiator.
Both radiation curing methods offer 100%-reactive, single-component systems, fast cure speeds, increased line speeds (up to 1,000 fpm), and the ability to coat heat-sensitive substrates -- making them increasingly popular for a variety of applications. In fact, the U.S. radiation-cured coatings market is growing rapidly at an estimated annual rate of 10%.11
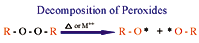
Applications
UV/EB curing is currently used in a variety of high-performance coating applications, such as topcoats for vinyl and wood flooring, metal and wood furniture, overprint varnish (OPV) for plastic and paper packaging and coatings, circuit board coatings, and coatings for plastic and metal containers. Emerging applications include indirect food packaging and high-end automotive finishing.Thermal Cure
Free radical crosslinked coatings using specialty acrylic esters can also be formulated using temperature-sensitive initiators such as peroxides or azonitrile compounds. In these systems, radicals are generated from decomposition of the initiator by heat, or by reducing agents like metal driers at ambient temperature, as shown in Figure 9.
Peroxide selection is dependent on the temperature at which the coating will be cured as well as the required shelf life of the coating. The key property is its half-life temperature, or the temperature at which half of the radicals are generated in a certain period of time.
Commonly used peroxides include methylethylketone peroxide, cumene hydroperoxide, t-butyl perbenzoate, and 1,1-di- (t-butylperoxy)-3,3,5-trimethylcyclohexane. The selection of peroxide determines whether the coating will be a single-component or a plural-component system.
An important consideration when formulating peroxide-cure coatings is that two competing cure mechanisms occur. At the coating surface, where oxygen is present, aerobic cure takes place; below the surface, where no oxygen is present, anaerobic cure occurs. Below the surface, curing occurs much faster than above since oxygen is a good free radical scavenger. Therefore, key to achieving uniform and complete cure is the elimination of oxygen at the surface either by mechanical means, such as inerting the atmosphere with nitrogen, or by chemical means, through the use of metal driers and monomers that are good oxygen scavengers (e.g., allylic functional monomers).
Peroxide crosslinked coatings offer 100%-reactive systems with higher film builds; isocyanate-free, formaldehyde-free systems, which ultimately allow low toxicity; low-temperature curing (as low as to 0degC); and the use of conventional application and drying equipment.
Applications
Peroxide crosslinked coatings using acrylic esters are currently used in high-performance automotive primers and topcoats, coatings for concrete bridge decks and industrial flooring, and specialty coatings for wood and paper.
Amine/Michael Addition Cure-in-Place Technology
Acrylic esters can also be used as reactive diluents and modifiers in other crosslinkable coating technologies, such as two-component epoxy systems cured with polyamines. In these systems, the amine-curing agent functions more as a co-reactant than as an initiator or catalyst. Acrylate esters can react with an amine through a Michael Addition reaction, as described in Figure 10. The resulting secondary amine-acrylate adduct can then react with another acrylate ester or, preferably, with the epoxy resin, forming a highly crosslinked polymer (see Figure 11).
Acrylate esters can be reacted with a number of amine curing agents, such as aliphatic amines, cycloaliphatic amines, amidoamines and polyamides. However, aliphatic amines are preferred due to their abundance of primary amine hydrogens. The most effective amine curing agents are based on diethylene triamine, triethylenetetraamine and tetraethylenepentaamine. Addition of an acrylate ester to an epoxy cure-in-place coating offers the benefits of 100%-reactive systems, viscosity reduction without loss of properties, fast ambient, sub-ambient cure without use of mercaptans, and reduced amine blush.
Applications
Michael Addition reaction crosslinking technology using acrylate esters is currently used in epoxy traffic paints; epoxy coatings for concrete, such as secondary containment and industrial flooring; and epoxy-based protective coatings, such as tank linings and corrosion-resistant primers.
The Future of Crosslinking Technology
Bottom-line conscious manufacturers are turning a critical eye inward to assess their cure processes and technologies. Many are finding that traditional cure methods are no longer a viable option, and are choosing to incorporate UV/EB, peroxide, and amine systems into their operations.
These advanced cure technologies enable coatings manufacturers to increase process efficiency, achieve faster dry times at lower temperatures, and boost production rates. In addition, the use of acrylic and methacrylic esters allow them to develop 100% reactive, solvent-free systems, reduce viscosity without sacrificing performance, and improve the overall quality of the coating.
For more information on crosslinking technology Circle Number 134.
A Crash Course in Crosslinking Technologies
Although the three environmentally kind coating technologies discussed here (UV/EB, peroxide, and amine/Michael Addition reaction) are rapidly growing in terms of both acceptance and use, traditional curing methods are still predominant in the coatings industry. Let's take a look at two conventional technologies to fully appreciate the alternate methods.Most coatings are air-dried, conventional coatings. These are typically formulated using a polymeric coating resin dissolved in an organic solvent; when the solvent evaporates a film is formed on the substrate. However, high levels of VOCs are emitted when evaporation occurs, making these hazardous to the environment. Additional drawbacks to air-dried coatings include long process times and high energy costs.
Condensation reactions (polymerization) provide an alternative to air-dried coatings. Usually initiated under moderate conditions of temperature and pressure, condensation reactions are completed at high temperature and low pressure, resulting in long process times.
The basic formulating differences between these crosslinking methods and the high-performance, low-VOC methods discussed in this article are shown in Table 1. The function of each component is also provided.
Reference
1 "Still Misunderstood But Making Progress: Rad-Cure Coatings," Coatings World, April 2001, p. 38.Looking for a reprint of this article?
From high-res PDFs to custom plaques, order your copy today!