Novel Fluoropolymer-Based Emulsion Technology

Striving For "Green" Coatings
Never stringent regulations by local and federal authorities are pushing the acceptable VOC level in paints down to much lower levels, in some cases as low as 50-100 g/liter. Advances in waterborne coatings technology have provided the market with new “green”, environmentally friendly and economical coatings options, many of them based on acrylic emulsions and polyurethane dispersions. According to the 2005 Chemical Economics Handbook, waterborne systems for architectural and industrial maintenance coatings comprised approximately 40% of the total coatings market globally in the year 2004.1More recently, advances in emulsion polymerization techniques have enabled us to develop new fluoropolymer-based waterborne materials for coatings. Traditional solventborne architectural coatings based on poly(vinylidene fluoride) (PVDF) homopolymers and copolymers have a proven track record of superior exterior durability and performance.2 In this paper we discuss novel waterborne systems based on PVDF polymers and copolymers, with durability and performance comparable to solventborne analogs. Products based on this new technology platform have now been commercialized under the KYNAR Aquatec® tradename.
In the first section of the paper the morphology of the fluoropolymer-based
waterborne materials is reviewed, and justification is provided for the chosen
structure. Also, data from South Florida-exposed test panels are presented,
which demonstrate that the influence of latex particle morphology on durability
is the same for both accelerated test cabinet and outdoor exposures. Later
sections of the paper deal with the formulation of this product for
after-market roof coatings. The important performance edge of the fluoropolymer
system over conventional binder systems is discussed, along with some of the
challenges for future developments.
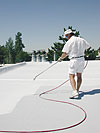
Latex Morphology-Its Effect on Weathering Performance
In order to understand the rationale for the morphology of the new fluoropolymer-based waterborne materials, it is useful to review the final film morphology of conventional solvent-based PVDF coatings. In a typical PVDF solventborne dispersion formulation, PVDF resin is dispersed in a latent solvent along with the right choice of pigments, co-resins and cosolvents. Typically, acrylic copolymers are chosen as co-resins since their miscibility with the PVDF resin is thermodynamically favorable, and the blend gives excellent final film properties. A PVDF:acrylic weight ratio of 70:30 seems to be a “magic” proportion that provides the right balance of excellent durability, along with physical properties such as gloss and adhesion. Since PVDF is a semicrystalline polymer, and the polymer is in a dispersion form, the coating needs to be baked above the PVDF melting point (170 °C) in order to obtain a homogenous, strong film. Upon baking at elevated temperatures, the PVDF crystallites melt and form a homogenous alloy with the acrylic resin. Upon cooling, large-scale PVDF crystalline structures reform, with the interlamellar regions consisting of an amorphous PVDF-acrylic phase.3 In principle, solvents are not required for this process to occur at high temperature, but in practice, latent solvents such as isophorone are often used to assist the film formation process, so that baked PVDF coatings are also usually relatively high in VOCs (500-700 g/L).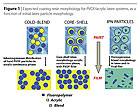
In the absence of heat to aggressively promote polymer mixing, the general principles of latex film formation suggest that a cold blend of the two latices will generate, in the film, a heterogeneous dispersion of the hard PVDF particles in the softer (better film forming) acrylic matrix.4 Starting from a core-shell morphology, the final film will have a more homogeneous dispersion of PVDF particles compared to a cold blend, but it will still have a continuous acrylic phase. The film morphology closest to that of the baked solventborne system would be predicted to arise from an IPN-type structure, where each particle already contains an intimate blend of PVDF and acrylic.
Since PVDF polymers are extremely resistant to photochemical attack, it was anticipated that hybrid latex particles with an IPN internal structure, generating a continuous network of PVDF polymer after film formation, would have much better exterior weatherability than core-shell hybrid latex particles generating only an acrylic continuous phase. To test this expectation, hybrid latex samples were generated with a range of particle morphologies, from core-shell to fully IPN. Coalesced clear coat films were formed from these samples, and the weatherability of the films was tested both in South Florida and in a UV fluorescent cabinet with UV-B (313 nm) bulbs - the latter conditions chosen specifically because they are known to accelerate the acrylic degradation rate, relative to the rate in Florida.

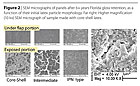
It is interesting also to note that, because of the superior weatherability of the PVDF polymer compared to the acrylic, the coating surface for all of these PVDF-acrylic blends becomes enriched in PVDF at the surface over time, as can be measured by attenuated total reflection IR spectroscopy. This occurs both in Florida and in the UV-B fluorescent cabinet, approaching 100% surface PVDF after long exposure times.6
These results show that the UV-B accelerated weathering is able to
reproduce much of the essential physics of the outdoor degradation occurring in
these clear coats in Florida.
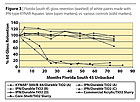
Given this demonstration of outstanding performance, we have now fully scaled up this new technology platform, under the KYNAR Aquatec trademark. The first commercial product, designated RC-10,206, has a minimum film formation temperature (MFFT) of about 30 ºC. To avoid any potential future health or regulatory issues, the product is completely free of any fluorosurfactants or alkylphenol ethoxylate (APEO) surfactants.
Applications in After-Market Roof Coatings
In North America today, a wide variety of materials is used to protect roofs. Important roofing substrates include metals such as aluminum or Galvalume®, concrete or composite tiles, modified bitumen, and flexible polymeric substrates such as EPDM (Ethylene Propylene Diene Monomer), flexible PVC, and TPO. The use of such polymer membranes is increasing for low-slope applications, both for residential and for commercial buildings.The “Cool Roof” concept can be understood by considering the energy balance at the roof surface.7 Some fraction of the total amount of solar radiation impinging on a building surface is reflected back into the atmosphere; the rest is absorbed. The absorbed energy, both from UV and visible light, but also from the infrared, which contains about half the total energy in the solar spectrum, heats up the surface above ambient temperature. Part of the absorbed energy is lost through thermal conduction, mainly into the building, and the rest of it is re-emitted back into the atmosphere through black body radiation. The dimensionless numbers characterizing the reflectance and thermal emittance material properties are called the Total Solar Reflectance (TSR) and the Emissivity (E) respectively.
As shown by studies conducted at the Lawrence Berkeley National Laboratory (LBNL),8 the most efficient way to reduce the heat build-up inside buildings is for the roof surface to have large values for both the solar reflectivity and the thermal emissivity. Many of the commonly used roofing materials are poor candidates for Cool Roof applications. Dark-colored polymeric roofing substrates typically have low TSR and moderate emissivity; uncoated metals have a high solar reflectance, but have low emissivity. The surface temperatures of such materials can rise 40 to 50 ºC above ambient on a sunny summer day at mid-latitudes. The cumulative effect in urban areas is to contribute to a rise in the average outdoor temperature, the so-called “Heat Island Effect”.
A simple and economical way of converting in-service and new roofs to Cool Roofs is to apply a high-TSR coating to the surface. Often these are white or off-white in color, although cool-roof metal oxide pigments (having a low reflectivity in the infrared) are also commercially available and have opened up broader regions of color space with reasonably high TSR values. In the LBNL study, typical temperature rise values for white paints under similar summer conditions were only about 10 ºC above ambient. For white coatings, appropriate choice of pigments and binder is necessary.
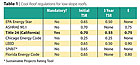
Of course, roof coatings must meet other performance requirements in addition to meeting Cool Roof standards. The coating is required to protect the underlying layers from UV, moisture degradation and other elements of weather. It should easily contract and expand with the roof substrate during hot and cold seasons. It should have excellent dirt pickup and stain resistance. Biological growth should not be supported and in some parts of the United States, some degree of fire resistance may also be necessary.
One commonly used low-VOC approach for aftermarket roof coatings is based upon low Tg elasto-meric acrylic copolymer emulsion polymers. The physical performance requirements for these acrylic coatings are detailed in ASTM D 6083. This standard thus can be used as a benchmark for the performance of other systems.
These elastomeric acrylic coatings have often been reported to have poor
dirt retention over time, leading to a decline in total solar
reflectance.9 For some white acrylic-based
coatings in the field, with an initial TSR of over 70%, drops in the TSR down
to as low as 20-30% in 2-3 years have
been reported.10 The causes for poor TSR
retention are multifold, including a pickup of dirt due to the low Tg,
a tendency of formulation components or photooxidation products to serve as a
nutrient source for dark-colored fungi and algae, and the ability for the
spores of microorganisms to gain a foothold on the coating surface once the
coating surface gets rougher due to weathering. Weathered and discolored roofs
can often be restored and recoated, but this option is cost intensive and
requires scheduled cleaning and washing.

The TSR retention of these coatings is enhanced by several other mechanisms.
The paints typically have much better dirt pickup resistance than elastomeric
acrylics. Also, since PVDF fluoropolymers do not support biological growth, and
since the coating surface remains smoother after long-term exposure, with an
enrichment in PVDF content at the surface over time, the coatings retain TSR by
means of their superior mildew and fungal resistance - even at long exposure
times when organic mildewcides would no longer be expected to be present in the
coating.

This new technology can be easily adapted to existing roofing systems. Because of the excellent property retention, we envisage that a thin coating of 1-2 mils (25-50 microns) would be sufficient to meet or exceed the performance criteria for ASTM D 6083, replacing much thicker conventional coatings while at the same time easily meeting long term “Cool Roof” requirements. Such a thin layer could be used either as a top coat over a thick primer layer of elastomeric acrylic, or could be used as a stand-alone layer on top of elastomeric membranes such as EPDM and modified bitumen.
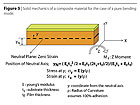
Coatings for EPDM Membranes
In the face of the new Title 24 requirements, a particularly urgent need is to have low-VOC coatings with the property balance of this new latex technology, which can be factory-applied on EPDM membranes. The use of EPDM has been growing rapidly and along with asphalt, it comprises almost 70% of the roofing substrate used today. Since the EPDM membranes are highly flexible, the coating should also be extremely flexible and able to respond along with the membrane during installation operations, and as the membrane contracts and expands during seasonal thermal variations. In working to adapt the new KYNAR Aquatec waterborne technology for this kind of application, we have encountered some unexpected challenges. Particular RC-10,206 coatings formulations, which easily passed a T-0 ductility test when applied on chromated aluminum, showed poor flexibility when applied on a highly flexible substrate such as EPDM - i.e., the flexibility of the system was not as good as the flexibility of each of its components.In order to understand the issue, we have undertaken some solid mechanics calculations to investigate the stress-strain distribution in the RC-10,206 topcoat on EPDM or aluminum, while the system is in a pure bending mode. The schematics of Figure 5 show the essential features of the calculation. The strain and stress in the coating are calculated in relation to the neutral axis, i.e., the plane at which strain is zero.11 In Figure 6 the stress-strain distributions of the two different systems are compared. As the system is bent, regions above the neutral axis, including the coating, are under tension. The strain increases linearly across the cross-section, relative to the neutral axis. The analysis shows that for the same radius of curvature, the final strain in the coating is similar for the two systems. In fact, the strain in the coating on aluminum is slightly higher than in the coating on EPDM, yet there is failure upon bending only in the latter case.

Mechanical analysis suggests that two different routes are available to improve the cracking resistance of the topcoat on flexible substrates. Either an improvement in the interfacial adhesion, or a reduction in the stress differential at the interface, should greatly reduce the cracking tendency. To improve the interfacial adhesion, both the use of a suitable tie-layer, and giving the system a force bake at about 60-80 °C, have proven to be effective solutions.
We have also recently demonstrated the ability to reduce the cracking tendency
through the route of reducing the stress differential at the interface. The
stress differential arises because the RC-10,206 topcoat has a much higher
modulus at room temperature than the EPDM. However, since the MFFT of the
RC-10,206 material is just slightly above ambient, and the MFFT is often very
close to the onset Tg (for single composition
latices), we reasoned that the addition of a small amount of plasticizer, or a
very slow coalescent such as Texanol® (which could function as an
effective plasticizer after two weeks ambient drying), might drop the effective
Tg enough to cause a significant drop in the
room temperature modulus of the topcoat.

Conclusion
This paper discusses a novel PVDF-based waterborne technology that provides a low-VOC, durable and environmentally friendly coating alternative to conventional binder systems. The KYNAR Aquatec technology is based on latex particles with intimately blended PVDF copolymer and acrylic, in an interpenetrating network-type morphology. South Florida weathering results, now at 5-7 years, confirm the importance of the latex morphology to the final film performance and durability. The new technology can be used in after-market roof coatings to provide long-lasting, colorfast low-VOC coatings for low-slope commercial roofs. With superior resistance to dirt pickup and biological growth, relative to elastomeric acrylic coatings, the new technology is ideal for Cool Roof applications where color retention is key to maintaining the solar reflectivity. The stain-blocking properties of the KYNAR Aquatec materials also contribute to color retention when modified bitumen is used as a roofing substrate.Over flexible substrates such as EPDM, mechanical analysis shows that good results can be obtained either by achieving excellent substrate adhesion by baking or use of a tie coat, or by using a small amount of a plasticizer or slow coalescent like Texanol to reduce the stress mismatch between the layers.
Acknowledgement
We would like to thank Wayne Skilton, Cynthia Hess and Segolene De Robien from the coatings group in Technical Polymers for their technical support. This work would not be possible without the latex synthesis expertise of Dr. Lotfi Hedhli and Dr. Ramin Amin-Sanayei from the fluoropolymer synthesis group. Last but not least our special thanks to business development managers Kevin Hanrahan and Jerry Petersheim for bringing new opportunities and applications.
This paper was presented at The Waterborne Symposium, Advances in Intelligent Coatings Design, February 2007, New Orleans, LA.
Looking for a reprint of this article?
From high-res PDFs to custom plaques, order your copy today!