Liquid-Impregnated Surface Coatings
The Science and Technology Behind “Slippery” Surfaces

Lubricant-impregnated surfaces, generally referred to as liquid-impregnated surfaces (LIS), are slippery surfaces that effectively eliminate the no-slip boundary condition, allowing viscous liquids, gels and emulsions to slide freely. Their superior nonwetting behavior and self-cleaning properties make LIS coatings attractive as a slippery surface for a wide variety of applications, including consumer packaged goods, agrochemical, oil and gas, manufacturing, medical, pharmaceutical, energy and utilities.
In addition, LIS coatings can provide significant sustainable benefits. They can reduce waste, increase product yields, improve production efficiency, provide consistent dosage and enable product innovation. In fact, a recent LiquiGlide survey1 confirmed that LIS coatings have the potential to address consumers’ concerns for waste. For example, 100 million gallons of lost product and billions of dollars of associated waste costs per year occur within processing tanks for the paint manufacturing industry alone.2 LIS coatings are currently being applied to these paint manufacturing tanks to recover these costs through the reduction of yield loss, waste water and cleaning time.
In this article, we will detail the science and application of LIS for the coatings industry, examine how LIS coatings compare to the traditional paint and coating technology, as well as review fundamental LIS concepts and coating design features.
About LIS Technology
LIS is a platform technology that uses dynamic and thermodynamic frameworks, which informs the design of stable, slippery coatings for specific applications. While traditional coatings create a dry, solid surface, LIS coatings are comprised of solid and liquid materials that are formulated to generate a long-lasting wet and slippery surface (Figure 1). The liquid layer is further designed to spontaneously wick into the textured (or porous) solid and last for the duration of the product’s lifetime. Therefore, while paint must coalesce and dry into a thin film, LIS coatings become functional as soon as the liquid is delivered.
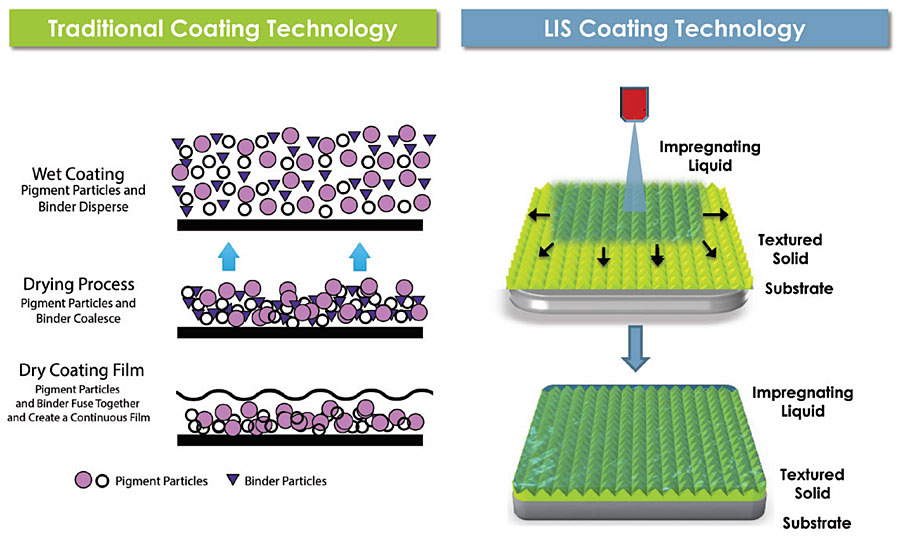
FIGURE 1 » Comparison of traditional and LIS coating technology regarding coating film formation. Traditional coating technology relies on dry film formation to achieve desired film properties while LIS coating technology utilizes a wet film to achieve slipperiness. (Schematic depicting the dry film formation was adapted from http://www.raincoating.com/system.html.)
Additionally, while there have been recent advances to incorporate “smart” functionality into traditional paint and coatings, many of these properties are already inherent to LIS coatings. The term “smart” functional coatings refers to a coating being engineered to respond to specific environmental stimuli, such as temperature, light or moisture, typically for the purposes of self-healing, self-assembling and self-cleaning.3 However, these traditional coatings rely on infusing chemical functionality or texture into the existing dry films to produce the desired “smart” functional effects. Self-healing coatings4 depend on the release of chemical payloads from microcapsules upon coating damage, while self-cleaning coatings5 usually depend on nanotechnology approaches to texture hydrophobic films or superhydrophobic chemistry to generate their nonwetting properties.
By contrast, LIS coatings directly incorporate slippery functionality into the liquid layer of the coating. LIS coatings are self-healing due to the mobile liquid layer that spreads throughout the textured or porous solid. LIS coatings are also self-cleaning because of their extremely low contact angle hysteresis and ability to repel a wide variety of liquids. In addition, LIS coatings can incorporate functionality to tune product sliding speeds via appropriate selection of liquid viscosity, coating thickness and underlying solid texture.
LIS Coatings versus Alternatives
LIS coatings are distinctly different than alternative slippery coatings, such as superhydrophobic surfaces or excess liquid approaches, because LIS coatings can maintain their slipperiness over time. Traditional superhydrophobic surfaces rely on the combination of low-energy materials and nanoscale/microscale texture to stabilize air pockets beneath a water droplet.6 The resultant surface is slippery as the droplet initially rests on top of the asperities of a rough surface and displays apparent contact angles that are greater than 150 degrees.7 However, under certain conditions, the air pockets can collapse and the droplet can become impaled, resulting in a nonslippery surface (Figure 2a). These conditions can include vibrations, squeezing, impact, rapid deceleration, application of an electric field or evaporation. Abrasion also reduces the slipperiness of these superhydrophobic coatings through mechanical degradation of the delicate nanoscale and microscale features that support these air pockets. Thus, superhydrophobic coatings have limited durability because they depend on stable air-liquid interfaces to maintain their slipperiness.
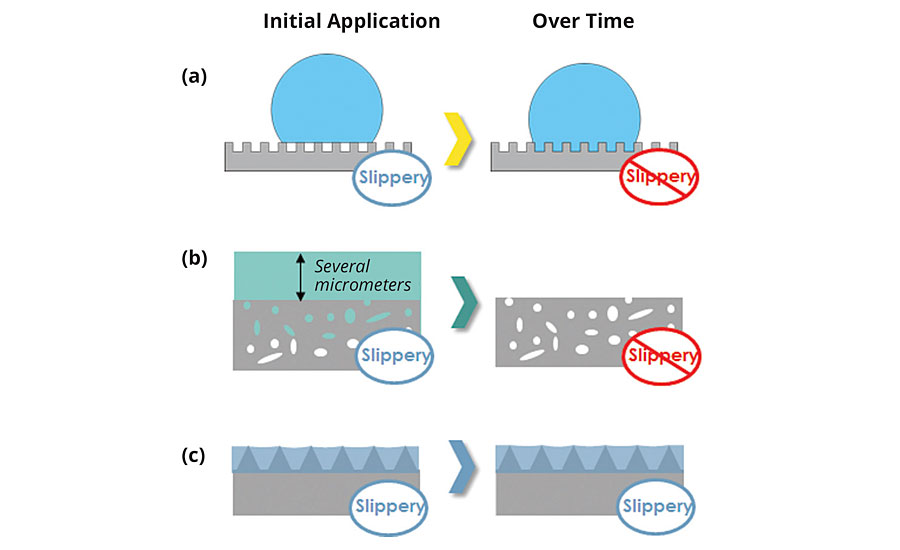
FIGURE 2 » Schematic depiction of the stability of alternative slippery coating technology as compared to LIS coatings. (a) Superhydrophobic coatings have limited durability due to an unstable air layer. (b) Excess liquid approach loses its slipperiness as the excess liquid drains over time. (c) LIS coatings maintain their slipperiness due to capillary forces.
Excess liquid approaches, such as slippery liquid-infused porous surfaces (SLIPS), have been shown to provide ice repellency, pressure stability and enhanced optical transparency.8 The excess liquid approach is predicated upon an excess lubricating film being “locked” in place by a micro/nanoporous substrate, where the lubricating liquid is applied to the surface with enough volume to completely submerge the micro/nanoporous substrate. Although an excess lubricating film can effectively eliminate contact line pinning for droplets, the liquid that is above the micro/nanoporous film is not stabilized by capillary forces and can readily drain by gravity and other forces, thereby compromising slippery properties9 (Figure 2b). Thus, excess liquid approaches will lose their slipperiness over time as the excess lubricating film drains from the micro/nanoporous substrate.
In contrast, LIS coatings are thermodynamically stable, durable coatings that can maintain their slipperiness (Figure 2c). They do not rely on air pockets nor excess lubricating films to generate their slipperiness. Instead, LIS coatings use capillary forces arising from microscopic texture to create pockets of lubricating liquid rather than air, and employ careful texture design to minimize the amount of solid material exposed, thereby ensuring extremely low levels of contact line pinning.10 Although capillary forces hold liquid tightly against the surface, the entrapped lubricating liquid is mobile within the textures, yielding excellent self-healing properties (provided the lubricating liquid preferentially wets the solid). In addition, LIS coatings remain slippery even if portions of the underlying solid texture are exposed. The thermodynamic framework (as described in the next section) is built upon the ability of LIS coatings to maintain their slipperiness at different equilibrium states without any excess liquid layer.
Fundamental LIS Concepts
LIS coatings are comprised of a textured or porous solid layer with appropriate surface chemistry and a subsequent liquid (or lubricant) layer that spreads throughout the solid texture and is stabilized by capillary forces. The working fluid (i.e., water droplet or product) slides on the liquid layer because the liquid layer effectively eliminates the no-slip boundary condition.
The key to engineering LIS coatings is the proper combination of surface chemistry and topology such that the lubricating liquid preferentially wets the microscopic textured solid. Therefore, the selection of the appropriate solid and liquid raw materials is crucial to the LIS coating design process. First, the liquid should be selected to be immiscible with the working fluid. It should also remain on the surface over the lifetime of the working fluid. Therefore, liquids with high boiling points and low vapor pressures are usually chosen to minimize liquid evaporation over time.
Second, LIS coatings use an established thermodynamic framework (Figure 3) as a guide to coating design. Dave Smith, co-founder and CEO of LiquiGlide, derived the thermodynamic framework that characterizes 12 different wetting states of a working fluid, such as water droplets, on a liquid layer contained within a textured solid.11 The wetting states have distinctly different interfacial configurations, both outside and underneath the drop, and are contingent on the ability of the liquid to spread over a working fluid. The configuration depends on the material properties of the working fluid, lubricating liquid, solid material and surface morphology, and the surrounding environment.
Figure 3 separates the 12 wetting states into two subsets of six different thermodynamic states based upon the spreading behavior of the liquid on the working fluid. When the spreading coefficient Sow(a) of the liquid (o) on the working fluid (w) in the presence of air (a) is greater than zero (Sow(a) > 0), the liquid will spread on the working fluid, forming a “cloak.” The cloak thickness is estimated to be on the order of nanometers, which may cause minimal entrainment, but is unlikely to affect the properties of the working fluid.12 For example, LIS coatings have demonstrated no adverse effects (taste, smell, color change, etc.) on mayonnaise products when in the cloaking regime.13 In the case where Sow(a) < 0, the liquid does not spread over top of the working fluid, and thus, no cloaking effect is observed. Noncloaking LIS coatings tend to be more robust and have greater longevity because the liquid layer is not depleted due to liquid entrainment.
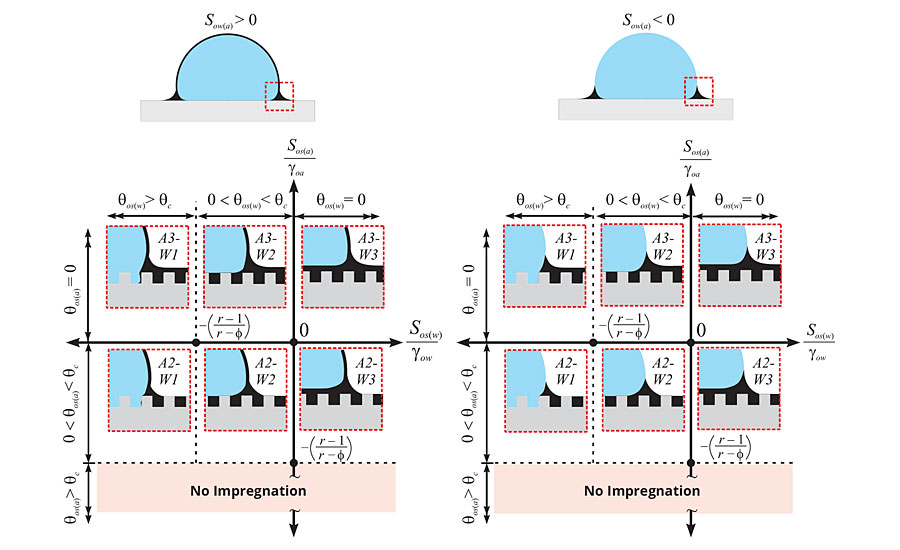
FIGURE 3 » Thermodynamic framework consisting of 12 different wetting states used to design LIS coatings. The top two schematics separate the wetting states into two subsets based upon the spreading behavior of the liquid on the working fluid (i.e. water droplet). The six possible states in each subset depend on how the lubricating liquid wets the underlying textured solid in the presence of air (vertical axis) and the working fluid (horizontal axis). (Reproduced with permission from ref. 11 from the Royal Society of Chemistry.)
Within these two subsets, there are three distinct morphologies underneath the working fluid that characterize the stability of the LIS coating. The first case (see states A3-W1 and A2-W1) is where the working fluid displaces the liquid layer and makes direct contact with the solid texture. The droplet becomes pinned to the solid texture and cannot move, thus, the resultant surface is not slippery and makes for a poor LIS coating. The second case (see states A3-W2 and A2-W2) depicts a wetting state where the liquid layer remains within the textured solid and the working fluid contacts only the exposed solid features. These surfaces can be very slippery when the exposed solid features are minimized in the texture geometry. The third case (see states A3-W3 and A2-W3) exhibits the most slipperiness as the working fluid makes no contact with the solid texture. The working fluid rests on a thin thermodynamically stable liquid film. While it is advantageous to design LIS coatings to achieve this third case, it may severely restrict the choice of lubricants available to meet this wetting criteria.
The solid surface chemistry and underlying texture play an important role in dictating the distinct morphology underneath the working fluid. The solid surface is characterized by a roughness (r) and a solid fraction (ϕ) parameter. The roughness is a measurement of the total surface area of the solid over the projected area, while the solid fraction denotes the fraction of solid that is exposed to the working fluid. The combination of roughness and solid fraction can be used to calculate a critical angle qc and establish the hemi-wicking criteria for the solid texture (where cos qc = (1- ϕ)/(r- ϕ)14). The liquid will spontaneously wick into the solid texture (in the presence of air) if its contact angle qos(a) on a smooth substrate of the same material is below the critical angle. However, stable LIS coatings need to satisfy the hemi-wicking criteria in the presence of the working fluid in order to maintain their slipperiness. To achieve wetting states in which the liquid preferentially wets the solid underneath the working fluid (A3-W2, A2-W2, A3-W3, and A2-W3), qos(w), must also be less than the critical angle qc.
The underlying texture is also an important parameter to control the sliding speed of the working fluid or product. For example, it was observed that water droplet sliding speed was reduced upon increasing the exposed solid fraction of the underlying texture.11
Product sliding speed can also be tuned via the viscosity of the lubricating liquid. Water droplets have been shown to have a much slower velocity on a higher viscosity 1000 cSt silicone oil lubricating liquid than a lower viscosity 10 cSt silicone oil lubricating liquid (when using the same underlying solid texture).11 It was determined that the sliding speed was inversely proportional to the lubricating liquid viscosity for a given solid texture.
When formulating LIS coatings for commercial applications, it is important to identify (a) an appropriate combination of solid and liquid materials to ensure preferential wetting and (b) a viable method for creating an underlying solid texture that will minimize pinning and produce a stable LIS coating over the product’s lifetime. For example, it can be difficult to match a suitable liquid and solid to satisfy both hemi-wicking conditions (qos(w) < qc, qos(a) < qc) in the presence of a complex product (e.g., mayonnaise) and ensure that the product does not displace the liquid. The precise understanding of the interactions among the product, liquid, solid texture and surrounding environment is nontrivial, but is necessary to determine the morphology of the contact line. Significant effort is taken to measure or predict these interactions to formulate LIS coatings. Common texture fabrication techniques, such as photolithography, wet etching, sol-gel synthesis and layer-by-layer, also tend to be too expensive, complex, or inefficient to produce a commercial LIS coating. Therefore, commercial LIS coatings must be designed to meet the thermodynamic wetting criteria as well as customer requirements such as their desired degree of “slipperiness,” longevity (e.g., over a product’s shelf-life) and regulatory requirements.
Future Directions
LIS coatings have untapped potential because they can be applied to a wide variety of industries to advance sustainability by reducing waste, increasing product yields, improving production efficiency, providing consistent dosage and enabling product innovation. LIS coatings can be used for moving viscous liquids through a pipe, on an external surface, from a tank or dispensing from a package. Moreover, LIS coatings are durable and can be formulated to maintain their slipperiness over the product’s lifetime. And while the LIS coating design parameters are complicated, they are reducible to commercial applications, such as those discussed for the coatings industry, which should continue to shift from dry film formation coatings to long-lasting wet surfaces.
References
1 LiquiGlide survey of 1,000 customers on their opinions about waste http://liquiglide.com/wp-content/uploads/
2014/11/141119-LiquiGlide-Consumer-Survey-Press-Release.pdf
2 https://www.environmentalleader.com/
2016/12/tackling-manufacturing-waste-inside-the-tanks/
3 Cain, R.; Krebs, R.; Lalgudi, R.; Risser, S. The Science of Smart Hydrophobic Coatings, Paint and Coatings Industry Magazine, 2017, Feb issue.
4 Thakur, V.K.; Kessler, M.R. Self-Healing Polymer Nanocomposite Materials: A Review, Polymer, 2015, 69, 369-383.
5 Gasman, L. Emerging Opportunities in Smart Coatings, Paint and Coatings Industry Magazine, 2016, Feb issue.
6 Zhang, X.; Shi, F.; Niu, J.; Jiang, Y.; Wang, Z. Superhydrophobic Surfaces: from Structural Control to Functional Application, J. Mater. Chem., 2008, 18, 621-633.
7 Zhang, D.; Wang, L.; Qian, H.; Li, X. Superhydrophobic Surfaces for Corrosion Protection: A Review of Recent Progresses and Future Directions, J. Coat. Technol. 2016. Res., 13, 11-29.
8 Wong, T.S.; Kang, S.H.; Tang, S.K.T.; Smythe, E.J.; Hatton, B.D.; Grinthal, A.; Aizenberg, J. Bioinspired Self-Repairing Slippery Surfaces with Pressure-Stable Omniphobicity, Nature, 2011, 477, 443-447.
9 Solomon, B.R.; Subramanyam, S.B.; Farnham, T.A.; Khalil, K.S.; Anand, S.; Varanasi, K.K. In: “Lubricant-Impregnated Surfaces,” Non-wettable Surfaces: Theory, Preparation, and Applications, Chapter 10, 285-318, 2016.
10 Quere, D. Non-sticking Drops, Rep. Prog. Phys., 2005, 68, 2495-2532.
11 Smith, J.D.; Dhiman, R.; Anand, S.; Reza-Garduno, E.; Cohen, R.E.; McKinley, G.H.; Varanasi, K.K. Droplet Mobility on Lubricant-Impregnated Surfaces, Soft Matter, 2013, 9, 1772-1780.
12 Schellenberger, F.; Xie, J.; Encinas, N.; Hardy, A.; Klapper, M.; Papadopoulos, P.; Butt, H.J.; Vollmer, D. Direct Observation of Drops on Slippery Lubricant-Infused Surfaces, Soft Matter, 2015, 11, 7617-7626.
13 For mayonnaise, LiquiGlide’s expert taste tester has observed no adverse effects (taste, smell, consistency) for coated bottles of commercial mayonnaise products.
14 Quere, D. Wetting and Roughness, Annu. Rev. Mater. Res., 2008, 38, 71-99.
Looking for a reprint of this article?
From high-res PDFs to custom plaques, order your copy today!