Comparing Powder and Liquid Coatings for Pipeline Applications

Pipeline anticorrosion coating applications represent what is probably the largest worldwide volume market for coatings products. This market’s potential naturally draws the focus of every coatings manufacturer, distributor and applicator, and for such companies the successful penetration into this market segment represents a large product sales volume increase over an extended period of time. Since large sales volumes are the primary goal of every manufacturer, the end user is bombarded with product marketing information, all claiming their product alone is best suited for pipeline anticorrosion applications. The sheer volume of information available on this subject can be overwhelming for the end user making a decision on a suitable coating system that is more prone to corrosion resistance and also has a long coating lifetime.
The situation is further compounded by typical factors inhibiting new technology acceptance, which include:
- Time required for new technologies to achieve a standardization recognized by the end user;
- End user reluctance to try new products prior to a lengthy historical performance of the product in field applications;
- Resistance of large pipeline coating corporations to adopt new products prior to recapturing initial investments in equipment utilized to apply traditional coatings.
In addition to the reasons defined above, there are many other nontypical factors that delay the wide acceptance of new coatings technology in pipeline anticorrosion applications. All multinational oil and gas companies, which represent the first step in product acceptance, are not only conservative regarding new product technologies, but are also large in structure and require time-consuming product technical approval and field trials. Due to the size of the investment required for pipeline installations, the conservative approach and requirement for field trials is not surprising, but when this lengthy approval process is combined with a plethora of coatings companies bombarding decision makers with marketing and sometimes unsubstantiated information regarding the product offerings, reaching a decision can be very difficult.
This article was prepared in an effort to assist in this decision-making process and to help end users understand the newer technology and to know the common differences between the traditional three-layer coating system and the latest polyurethane coating technology. The information contained was compiled from published data that is readily available to the public on the internet or from other resources, and includes information and comparisons of products such as three-layer PP or FBE and polyurethane coating systems. A valid attempt has been made to show product strengths and weaknesses for the products supplied by the author’s company and by other companies.
In today’s market, anticorrosion coatings must generally be environmentally friendly, safe during application, durable, provide chemical resistance and be capable of isolating the substrates they are protecting from oxidation. In addition, qualified coatings should be resistant to mechanical damage from the coating process stage, through installation procedures and over the designed service life, as well as fall within industry standards on a cost basis. When used as a pipeline coating system, other requirements such as cathodic disbondment, bendability, temperature resistance (both high and low) and other factors are critical as well.
From the information available it is apparent that no traditional coating product is completely meeting the needs of the end user at the moment. Traditional products are specified because they are deemed to be the best that are available. The author contends that this may have been the case five or 10 years ago, but it is not the case now, and that use of many traditional coatings products is being maintained due to a lack of information for the end users to properly research and investigate the newer technologies available. The facts are that newer coating technologies can provide end users with anticorrosion coatings for pipeline systems with:
- Much higher physical properties, providing longer investment maintenance and reduced costs over time;
- Reduced instance of damage during installation, higher oxidation resistance;
- Higher process temperature ranges;
- Faster application and installation;
- Better field jointing methods.
There are companies representing these newer technologies that are reputable, have invested the time and resources to formulate products specifically for pipeline applications, provide representative product information and are capable of delivering completed coating systems inclusive of the required technical expertise, production capacity, and quality assurance and control required for projects of this nature.
Time and effort should be expended to carefully review the potential of these newer coating technologies, with the goal of achieving industrial standards, application standards and other criteria to reach worldwide acceptance of the products as at a minimum an alternate system to traditional coating systems commonly in use.
Powder Coatings (Fusion-Bonded Epoxy)
Fusion-bonded epoxy (FBE) is an epoxy-based powder coating that is used to protect various sizes of steel pipes used in pipeline construction and on a wide variety of piping connections, valves, etc. from deterioration due to corrosion. FBE coatings are thermoset polymer coatings. They come under the category of ‘protective coatings’ in paint and coating nomenclature. The name ‘fusion-bonded epoxy’ is derived from the way of resin crosslinking and the method of application. FBE coatings are in the form of dry powder at normal atmospheric temperatures. The resin and hardener parts in the dry powder remain unreacted at normal storage conditions. At typical coating application temperatures, usually in the range of 180 °C to 250 °C (360 °F to 480 °F), the contents of the powder melt and transform to a liquid form. The liquid FBE film wets and flows onto the steel surface on which it is applied, and soon becomes a solid coating by chemical crosslinking, assisted by heat. This process is known as fusion bonding. The chemical crosslinking reaction taking place in this case is irreversible, which means once the curing takes place, the coating application of further heating will not “melt” the coating, and thus it is known as a “thermoset” coating.
Chemistry of FBE Coatings
An epoxy or oxirane structure contains a three-membered cyclic ring - one oxygen atom connected to two carbon atoms - in the resin molecule. This part is the most reactive group in the epoxy resins. Most commonly used FBE resins are derivatives of bisphenol A and epichlorohydrin (Figure 1). However, other types of resins (for example bisphenol F) are also commonly used in FBE formulations to achieve various properties, combinations or additions. Resins are also available in various molecular lengths, to provide unique properties to the final coating.
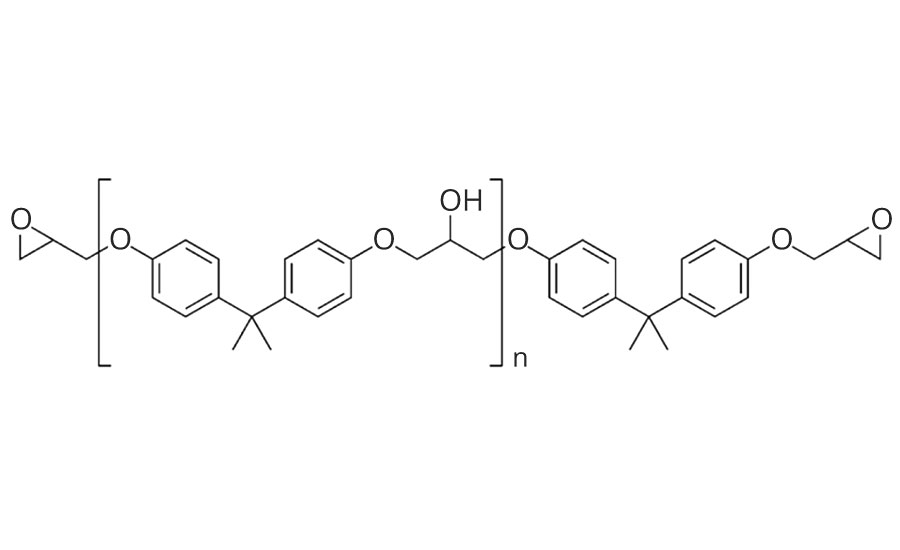
FIGURE 1 » Structure of unmodified bisphenol A-type epoxy pre-polymer. n denotes the number of polymerized subunits and is in the range from 0 to about 25.
The second most important part of FBE coatings is the curing agent or hardener. Curing agents react either with the epoxy ring or with the hydroxyl groups, along the epoxy molecular chain. In addition to these two major components, FBE coatings include fillers, pigments, extenders and various additives, to provide the desired properties.
Key Features
This system has been widely used since 1970. The system is comprised of powder-coated epoxies. The product is typically specified in low-abuse, low-temperature applications such as trunk lines. The product is also commonly specified for use as the anticorrosion layer beneath concrete weight coatings in subsea applications (Table 1).
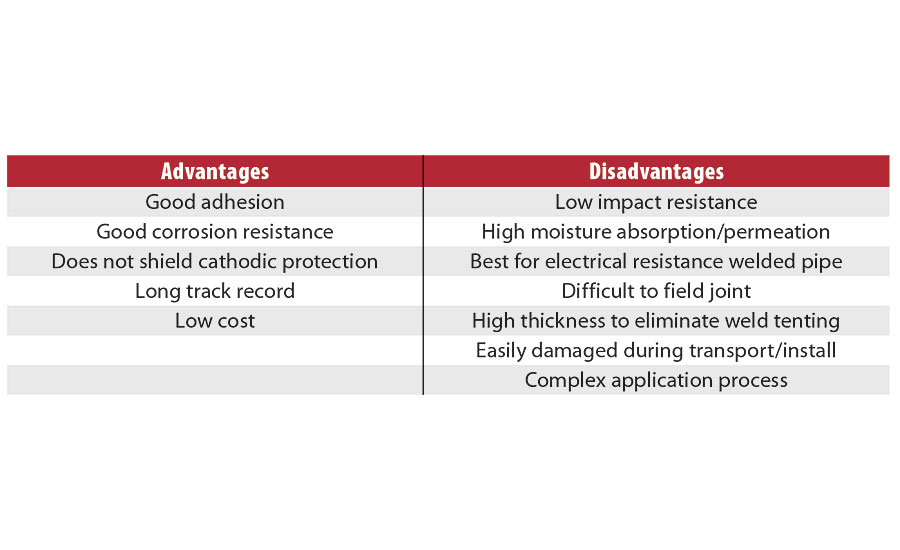
TABLE 1 » Advantages and disadvantages of FBE coatings.
Summary of FBE Coatings
FBE, amongst various powder coatings, has been utilized by major end users for more than three decades and still remains the predominant low-cost coating system. The most important issue with FBE coating is field jointing, which is typically accomplished using shrink wrap sleeves. These are slow to apply, expensive and prone to failure over time. The product has certain acceptable physical properties, but with the latest trends and recent developments in coatings systems there are now many alternative options for end users to look into. Most importantly, end users look for a balance between the price and quality of the product. As of today there are many better options compared to FBE in terms of service life.
Liquid Coatings (Polyurethanes)
Polyurethanes are the fastest growing technology within the coatings industry as a whole. Generally, this chemical group is broken down into two general groups, polyurethane and polyurea. Some studies further divide these two groups down into elastomeric and rigid subgroups. This subdivision is frankly not relevant, as the rigidity of either product is determined by formula modification, with degrees of flexibility and rigidity easily modified as part of a specific design to meet specific applications requirements. In different applications, polyurethane has this tendency to be modified chemically to suit the requirements of a particular project.
Chemistry of Polyurethane
A polyurethane (PU) coating system is basically a two-component coating system with no solvent in it. So one can say it is a solvent-free, plural-component coating system. The simplest PU is linear, in which the hydroxyl compound and the nitrogen compound each have a functionality of two. This can be represented by the structure in Figure 2.
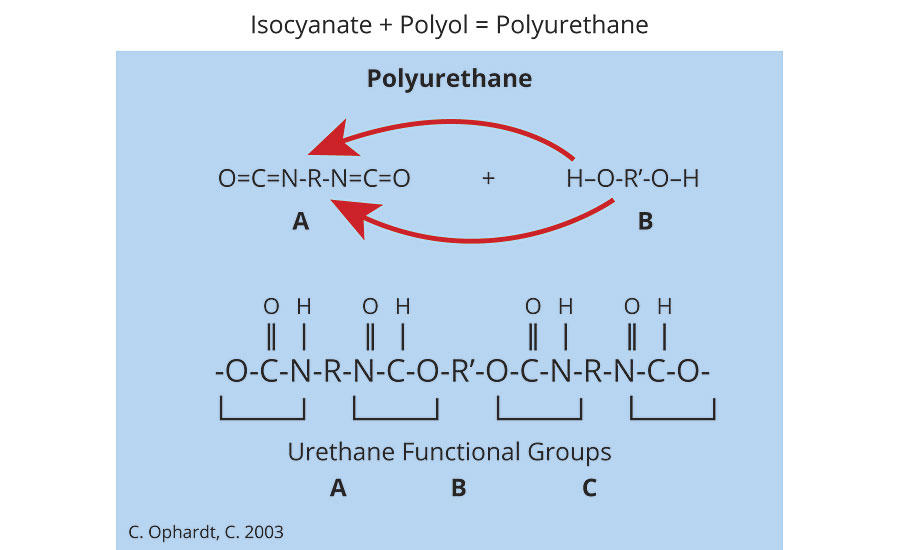
FIGURE 2 » Chemical structure of polyurethane.
Polyurethanes are made from a di-alcohol and di-isocyanate monomers. The isocyanate compounds contain the functional group (O=C=N-). A rearrangement reaction leads to the formation of the urethane linkage. A hydrogen moves from the alcohol to the nitrogen, while the oxygen links to the carbon. The urethane functional groups are similar to the amide group. In some applications the urethane polymer chains are further reacted to make crosslinks.
The isocyanate can react with different chemical groups, so the final properties of the polymer will vary according to the reaction route taken. Therefore the formulation of a PU must take into account every possible reactive constituent. PUs may have a very widely varying structure depending on the type of isocyanate and the type of reactive hydrogen components present in the formulation. The presence or otherwise of the various groups along the urethane linkage will control the end properties of the polymer. The curing of a PU can be regarded as the formation of a network, also called crosslinking.
Key Features
In 1930 Otto Bayer and his associates discovered di-isocyanate addition polymerization procedures, which resulted initially in the development of polyurethane foams and coatings. Since the 1980s polyurethane was not considered as one of the mainstream anticorrosion coating systems, but recently the trend has changed, and now end users are more inclined to use a system that has more advanced technology to support their product in a better way and will serve the purpose for longer as compared to traditional coatings. Additionally, there are many other factors that are changing the minds of end users to switch to this new technology (Table 2).
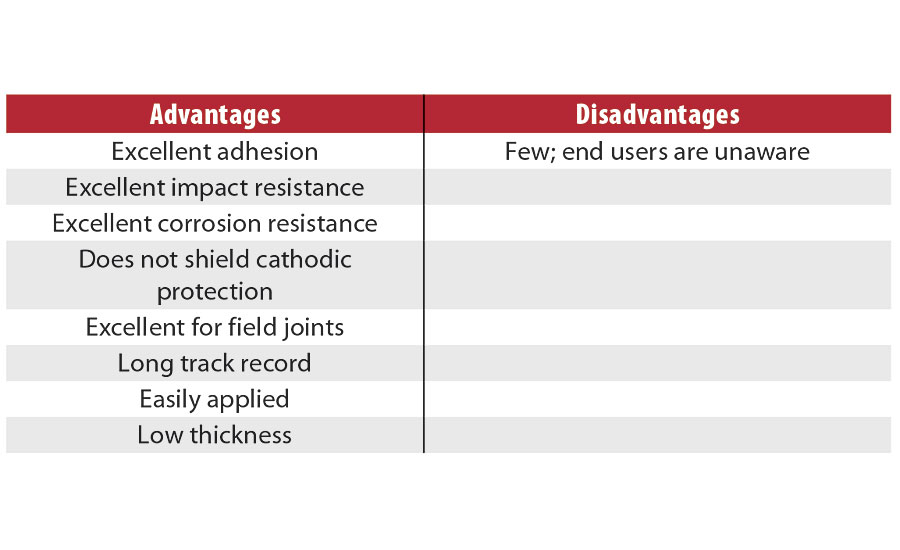
TABLE 2 » Advantages of polyurethane systems.
Summary of Polyurethanes
In summary, polyurethane was developed almost more than 70 years ago. Over the past 50 years research and development have made this product group a widely accepted product in a variety of applications. The facts are that newer coating technologies can provide end users with anticorrosion coatings for pipeline systems with much higher physical properties, providing the end user with longer investment maintenance and reduced costs over time, as well as reduced instances of damage during installation, higher oxidation resistance, higher process temperature ranges, faster application and installation, better field jointing methods and lower costs over time. Another very important advantage of polyurethane coatings over other traditional coatings is their faster curing time, as most polyurethanes set up very quickly, within seconds or minutes of application, reach a 75% cure in 12 hrs and reach full cure within 24 hrs.
Additionally, when comparing traditional coatings with polyurethanes, a major advantage is that there are no primers or adhesives used with polyurethane coatings. They are applied directly to the steel substrate, giving rise to more adhesion and fewer chances of delamination.
Comparing Coating Systems
Typical Properties
Tables 3 and 4 compare some typical properties of three-layer PE or FBE with polyurethane coatings.
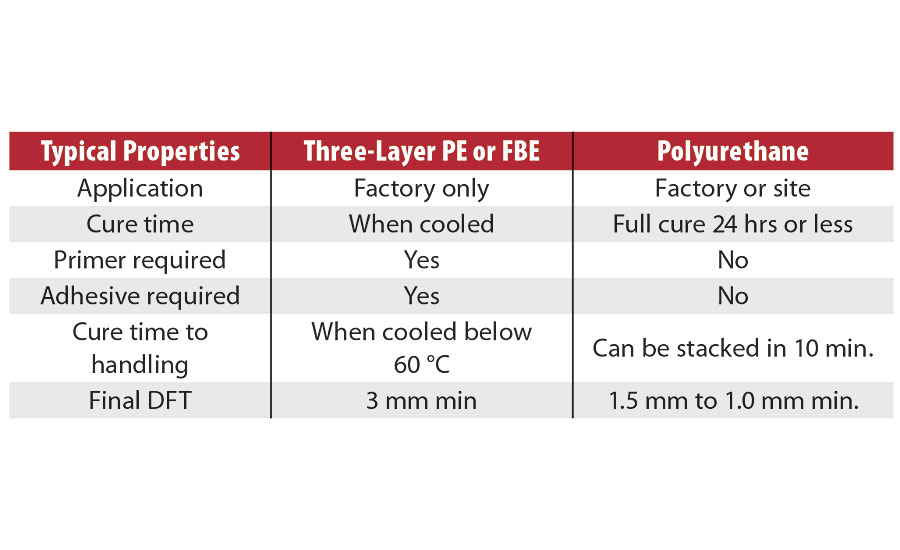
TABLE 3 » Comparing typical properties of three-layer PE or FBE with polyurethane.
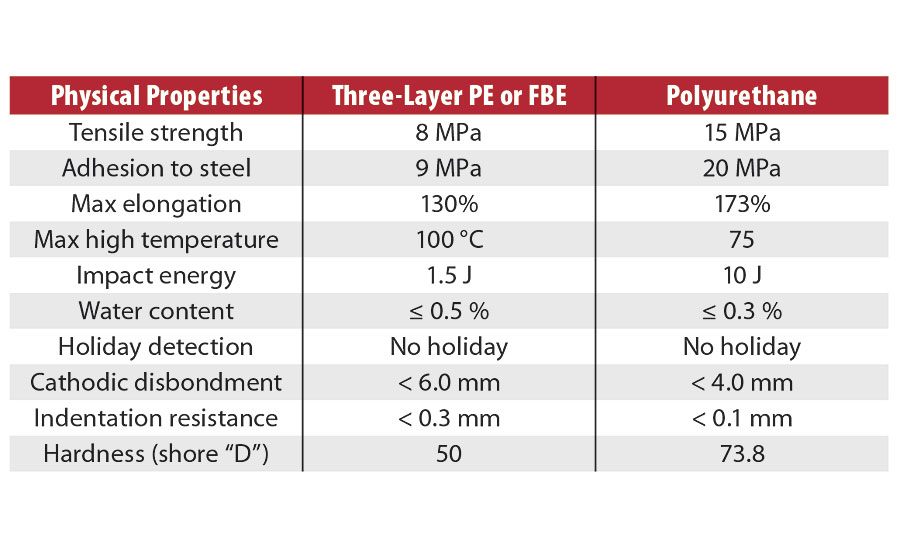
TABLE 4 » Comparing physical properties of three-layer PE or FBE with polyurethane.
Production Processes
Steps involved in the FBE production process are as follows:
- Visual inspection
- Preheating
- Blast cleaning
- Internal dust removal
- Visual inspection
- Chromate treatment
- Pipe coating heating
- FBE coating, 1 or 2 layer
- Quenching
- Holiday inspection
- Pipe end cleaning
- Final inspection
The steps involved in polyurethane production are as follows:
- Visual inspection
- Blast cleaning
- Internal dust removal
- Visual inspection
- Polyurethane coating
- Holiday inspection
- Pipe end cleaning
- Final inspection
- Identification and storage
From the above production processes, it is obvious that polyurethane coating applications have less-complex requirements. What is not apparent, are the additional quality assurance steps that must be performed in association with each of these additional steps. For example, FBE application requires preheating, chromate treatment, reheating, FBE application and quenching steps that all require detailed production steps for the process to be completed correctly. If heating temperature limits are exceeded, weakening of the pipe wall structure is possible. If chromate application processes are not exact, a nonconforming system will result. Each step in the process has further potential for error and thus failure.
The lower complexity in the production process of polyurethane not only reduces the chances of error but also reduces the complexity of a laborious process and the steps involved in quality assurance.
Field Jointing Methods
Field jointing and associated costs and time requirements are an often overlooked, but are a very relevant feature of any pipeline coating system. Here we will have a look at the field jointing methods being applied with both of these coating systems.
Field Jointing Methods for FBE
FBE systems typically utilize epoxy compounds that are mixed and applied to the joint area, providing an epoxy enamel-based field joint without the fusion bonding that provides the system with some of its integrity (Figure 3). Without fusion bonding, epoxy enamels simply do not hold up to the design life for which the original coating was intended.
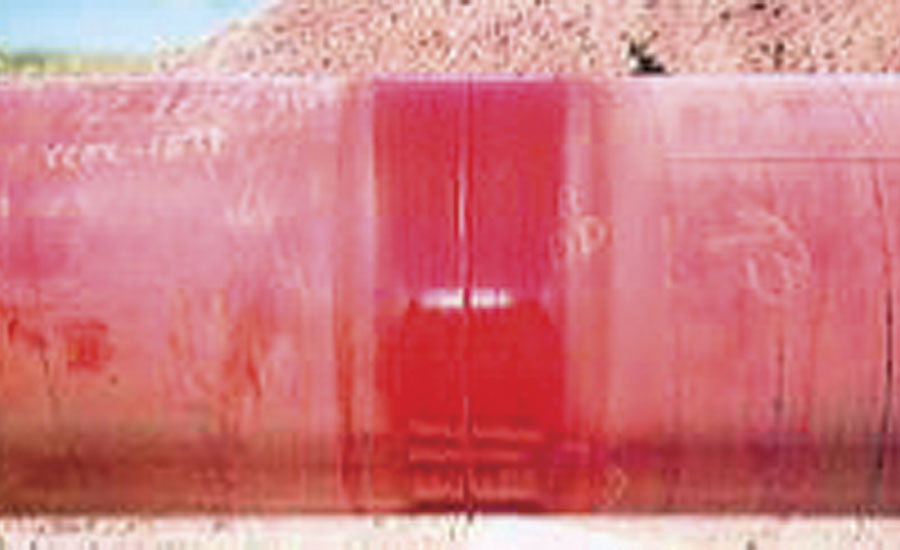
FIGURE 3 » Blistering in FBE joint field coating due to moisture absorption.
Apart from this, jointing of three-layer coating systems is typically accomplished using shrink sleeves, and results in a less-qualified product with two seams for every pipe joint. This method provides a two-layer field joint with seams to protect each end of the tri-lam system, and there is no fusion-bonded epoxy beneath the shrink sleeve. Shrink sleeves are the apparent product of choice for tri-lam and FBE since there is no better method of closure readily available; it is relatively simple to perform and requires little equipment to complete (Figure 4). This process is typically three to four times more expensive than the production cost of applying the base system, and is nowhere near as qualified from a performance standpoint. The process also takes an extensive amount of time to complete.
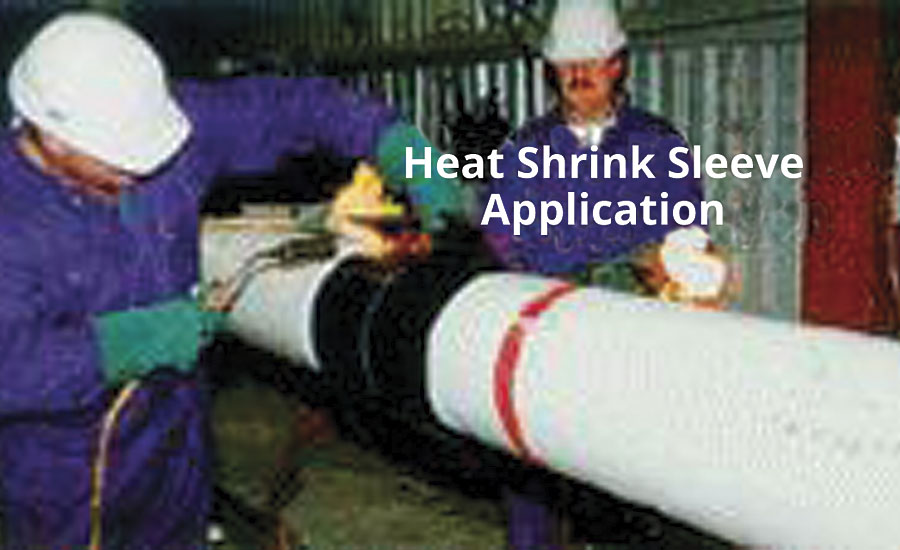
FIGURE 4 » Adhesion failures.
Field Jointing Method of Polyurethane
Polyurethane is field jointed in the same manner as the production process. The same high-pressure airless spray unit and heated equipment are used for field jointing that are used in the pipeline application process. The girth welds are cleaned of oxidation using garnet for grit blasting, bringing the surface profile to a pristine state. Polyurethane is applied in the same way, giving excellent adhesion and corrosion resistance to the field joints as it does for the original pipeline.
This process provides a continuous, monolithic (no layering) application of the pipeline from one end to the other, regardless of length (Figure 5). The system integrity of the joints is identical to that of the production-coated pipes. No other coating system can provide equivalent quality at field joints, or for that matter, a seamless, monolithic system in total.
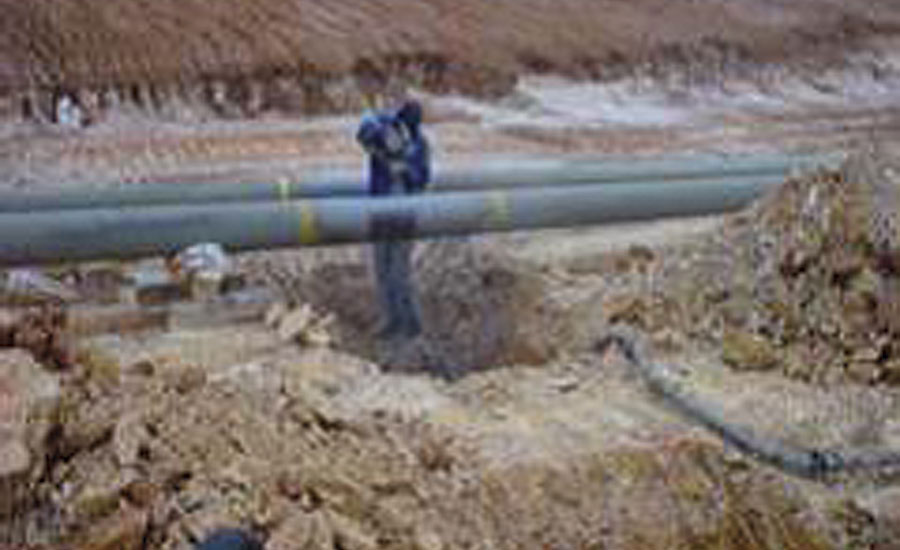
FIGURE 5 » Corrosion under a three-year-old three-layer PE coating.
Coating Failures
The principal causes of traditional pipeline coating failures, with the definition of failure being a leak, include external corrosion, internal corrosion, mechanical damage, design flaws, equipment malfunction, and maintenance and weld failure. The leading factor for pipeline failures in all studies was by far attributed to external corrosion. As stated previously, the different studies include a wide range of variables, but in all cases external corrosion was the number-one cause of failure (Figure 6).
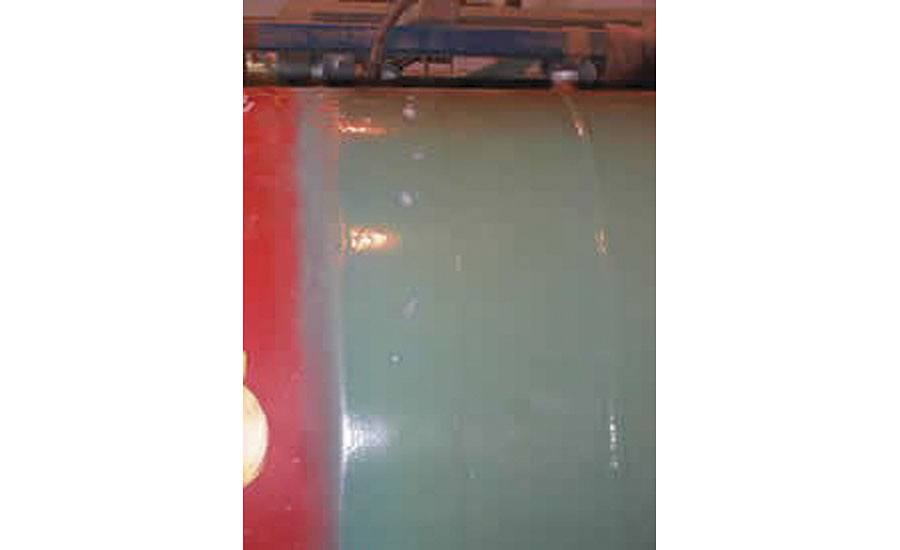
FIGURE 6 » Heat shrink sleeve failure.
Principal Causes of Three-Layer FBE Coating Failures
Three-layer PP or FBE coatings show problems. After a couple of years (and sometimes after only a few months), some buried pipes that are coated with these systems show complete delamination between the steel substrate surface and the coating system, which results in a complete failure. The key to achieving good adhesion is a fully cured epoxy. If the epoxy material is not appropriate, or if it is not fully cured and is coming in contact with water or humidity, it can lead to disaster since uncured or incorrect epoxy is very hydrophilic. As a result, this water can lead to corrosion (Figure 7).
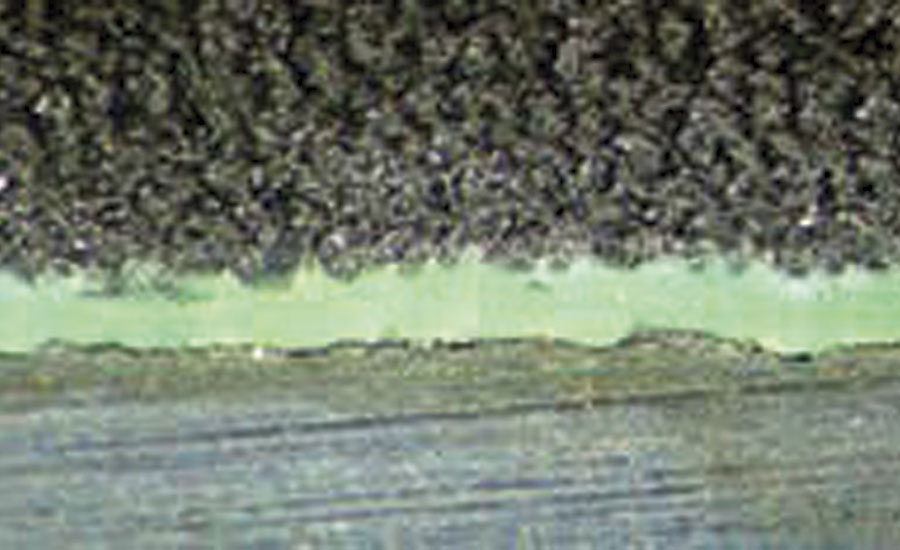
FIGURE 7 » FBE field joint.
A second drawback with three-layer PP or FBE is the mechanical damage that can take place while transporting the pipeline or while laying it down. The chances of damage are very high, as the coating has very low resistance to mechanical damage. To reduce failure instances caused by impact damage during the transportation, the most obvious solution is to eliminate as many transport and handling steps as possible. Many pipe manufacturers now include coating facilities within the plant to eliminate transportation to and from a pipe coating facility at another location. Traditional pipe coating facilities, however, are not mobile, have high power consumption levels and require a high level of environmental control to work effectively. This condition is typical of FBE and tri-laminate products.
Another important cause of coating failure in traditional three-layer coating systems is failure at the girth welds at the field joints. These FBE coatings cannot be used at the field joints or girth welds, and results in the need for shrink sleeves tapes (Figure 8). When installed properly, shrink-wrapped joints perform fairly well, but again the process of properly installing them requires diligent installers and is time consuming. Time constraints on projects, weather and other factors result in a high percentage of the shrink-wrapped joints being completed to less than ideal conditions. Even in the best of conditions, shrink-wrapped joints present two circumferential seams at every girth weld, and seams are prone to failure (Figure 9).
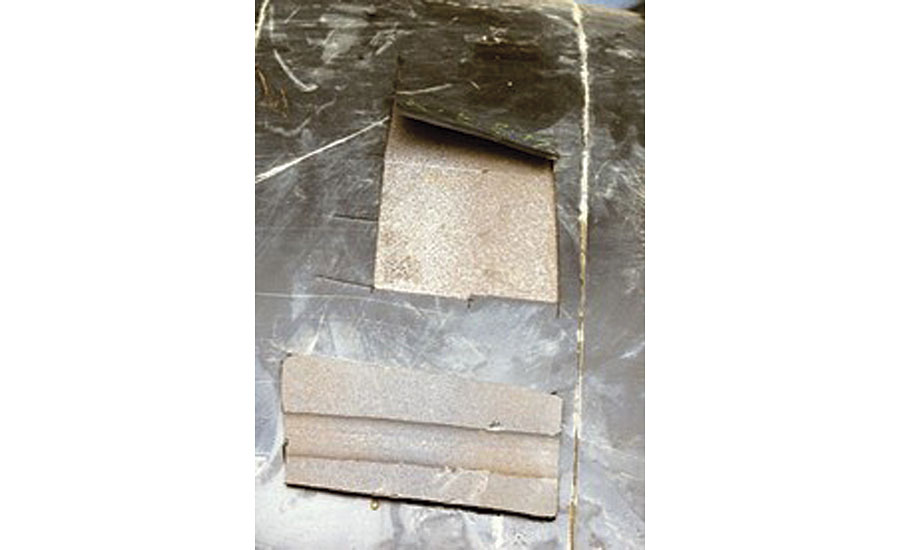
FIGURE 8 » Heat shrink sleeve application.
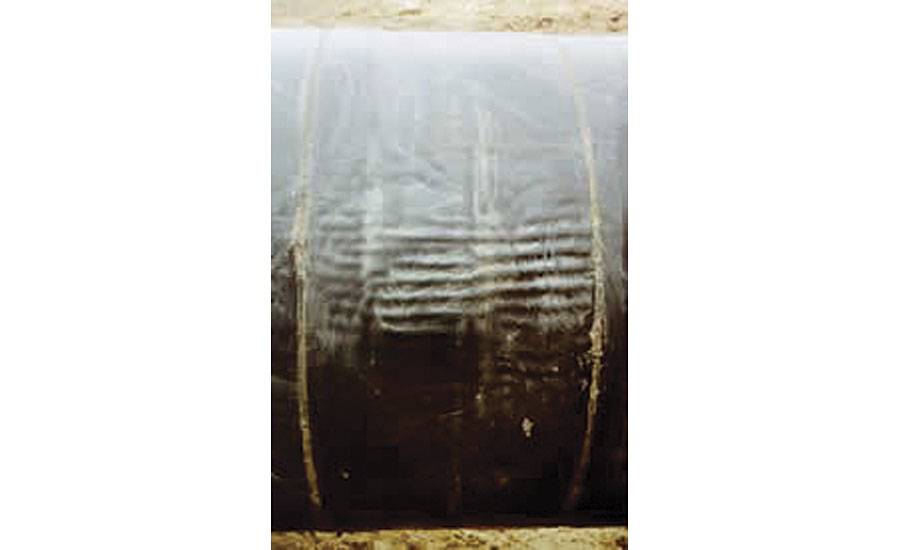
FIGURE 9 » Field jointing with polyurethane.
How Polyurethane Coatings Can Reduce Common Causes of Failure
From comparing the physical properties of both of these coating systems, it is apparent that polyurethane coatings meet, and in most cases exceed, the properties exhibited by both FBE and tri-laminates. These physical factors can make the use of polyurethane coatings feasible from the critical aspect of performance.
Damage from impact abuse during transportation and construction is reduced because the impact and puncture resistance is significantly higher than that provided by either FBE or tri-laminates. Damage related to transportation and handling can be virtually eliminated by performing production coating at a location that best suits the end user.
Failures of girth weld field joint coatings are reduced because polyurethane coatings are applied to field joints, meeting the same durable specifications as those applied in the production facilities. There is no difference in the quality of the field joint compared to the main pipe section coating.
Failure of cathodic disbondment systems is reduced because polyurethane coatings are equal in field applications to the production applications. The coating at anode placement will be equal to the main pipe coating. In addition, better physical properties and local production coating mean fewer instances of damage and fewer potential failure sites due to disbondment at damaged locations. Higher levels of durability and better field applications translate to less damage attributable to cathodic disbondment features.
The most important aspect is polyurethane coatings are less prone to moisture absorption, leading to fewer chances of external corrosion due to water absorption.
Conclusion
The use of many traditional coating products has been discontinued in recent years for a variety of reasons, including common failures, durability, environmental concerns, etc. Though FBE and tri-laminate products are presently still being considered by some end users as anticorrosion systems, most have now started using the new polyurethane technology in most pipeline coating applications. This is because this technology is more prone to corrosion resistance and mechanical damage, is easy to apply, has fewer chances of failure, and offers reduced cost over time. Another major advantage is that the product’s production and field joining process is simple, consistent, seamless and durable as compared to that of three-layer coating systems.
When comparing physical properties of both coating systems, polyurethanes deliver better hardness, tensile and impact values, as well as better cathodic disbondment and adhesion-to-steel standards.
References
This document was prepared in part by using published data from published reports or websites. The primary resources utilized in the preparation of this document are as follows:
1. Anticorrosion Protective System. (n.d.). Retrieved from APSDUBAI: www.apsdubai.com.
2. David Norman Corrosion Control. (n.d.). Retrieved from David Norman Corrosion Control: www.davidnormancorrosioncontrol.com.
3. Hornbaker, M. (2013). The Basics of Liquid and Powder Coatings. Retrieved from www.thefabricator.com.
4. Munger, C.G. (1984). Corrosion Prevetion by Protective Coatings. In C. G. Munger, Corrosion Prevetion by Protective Coatings.
5. powdercoatguide. (n.d.). Retrieved from Powder Coat Guide: www.powdercoatguide.com.
6. Shweitzer, P.A. (2005). Paints and Coatings: Applications and Corrosion Resistance.
Looking for a reprint of this article?
From high-res PDFs to custom plaques, order your copy today!