How Light and Pigment Interactions Affect Hiding, Tint Strength and More

Credit: Davizro, iStock/Getty Images Plus, via Getty Images
In previous "Formulating with Mike" articles, I covered pigment additives such as dispersants, surface tension modifiers and control agents. This month I will discuss how light and pigment interactions affect hiding, tint strength and other properties of a coating. Stay tuned, as later in this series, we will go into depth on the pigment dispersion process.
Pigments, unlike dyes, are finely ground insoluble particles, and pigments are used in most coatings because they provide critical properties for the intended application. The pigments used depend upon the specific application. Pigments are divided into three main types: primary, extender and specialty.
Primary pigments give the coatings color and hiding, but they tend to be expensive. Extender, or "filler" pigments, are added to control gloss and influence other physical properties, but at a lower cost. Specialty pigments are added for a specific purpose and include anti-corrosive, effect pigments and nanoparticles.
Since primary pigments tend to disproportionally affect the cost of a coating, using them optimally is necessary. They can be organic or inorganic (natural or synthetic), or carbon black, as it has distinct properties that are neither inorganic nor organic. Primary pigment particles interact with light, and the eye receptors send a signal to the brain, which it interprets as color.
The human eye perceives colors by what frequencies are not absorbed. We see the frequencies that enter your eye from the object, also known as subtractive color. As the light interacts with the coating, particularly the primary pigments, some of the light in the 400-700 nm range (the visible light range) gets absorbed at different efficiencies. As more light is absorbed, the object becomes darker until it becomes black — the result of absorption of all frequencies in the visible spectrum. If only some frequencies are absorbed, we see the color of the non-absorbed frequencies. If no frequencies in the 400-700 nm range are absorbed, we see white.
If light passes through a film and reaches the substrate and then exits the film, the color of the substrate and not the film will be visible. As less of the light reaches the substrate before exiting the film, the color of the film starts to dominate what we see. If no light reaches the substrate and leaves the film, we only see the color of the film, and have full hiding. Therefore, we must prevent light from getting to the substrate and then exiting the film. To do this we use pigments, particularly primary pigments, to scatter light. From Figure 1 you can see the difference in hiding between high- and low-scattering pigments. While pigments tend to absorb only some wavelengths to generate color, with every interaction with a pigment or other item in the film, some energy of all wavelengths is lost, leading to eventual extinction of incident light, if the light does not leave the film quickly enough. This torturous route of moving through the film means that pigments with prominent levels of scattering, reflection or absorbance will prevent light from reaching the substrate and exiting, providing complete hiding.
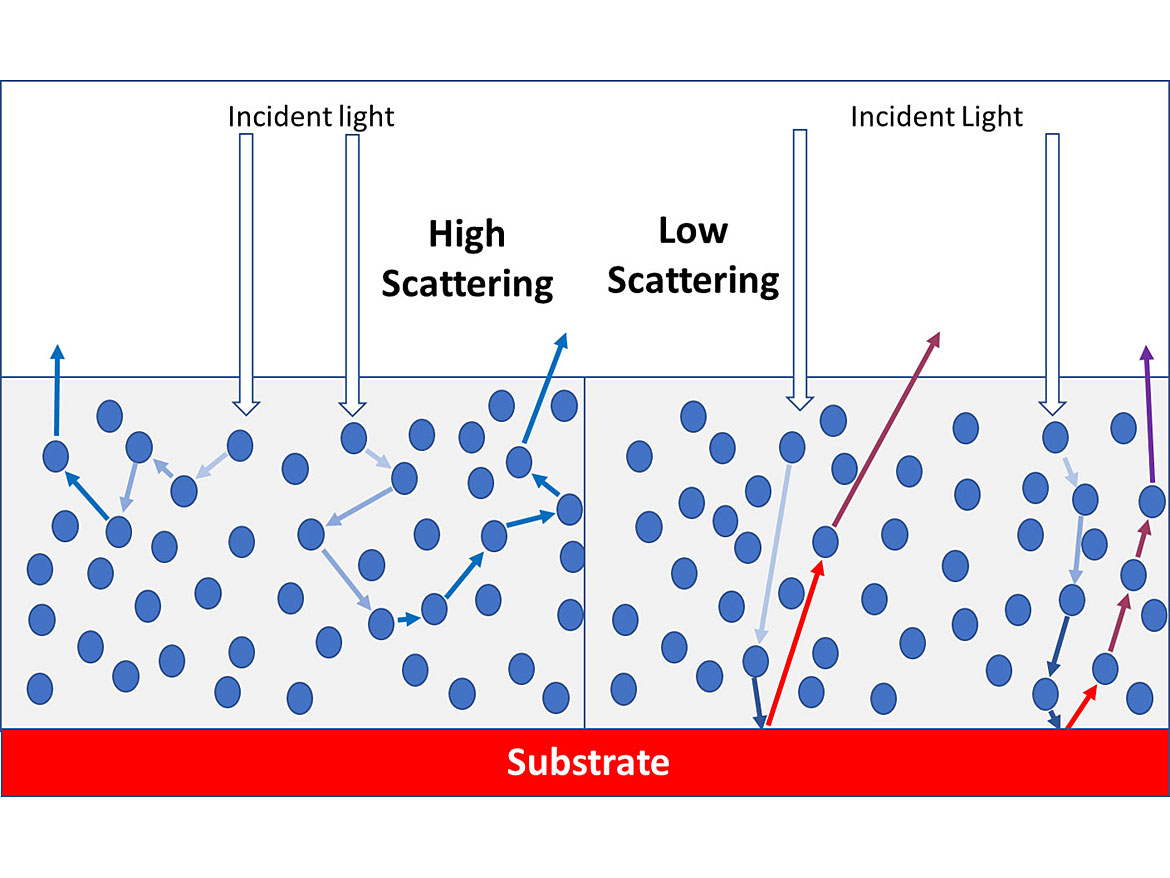
As the light enters the film, we want to prevent it from reaching the substrate and exiting; the pigments do this by interacting with the light. When light interacts with a particle, there are five possible outcomes: refraction, diffraction, absorption, reflection or no change. All of these are considered scattering, except ‘no change’. Refraction is when the light path is changed as it passes through the boundary of the particle and surrounding media. Diffraction is when the light is bent by interacting with the edge of the particle. Absorption is when the energy of the incident light is attenuated by the particle, but not necessarily all frequencies equally. Reflection is when the light that hits the film returns to the source. We want to maximize scattering and minimize ‘no change’ to optimize hiding and color. Figure 2 Illustrates this.
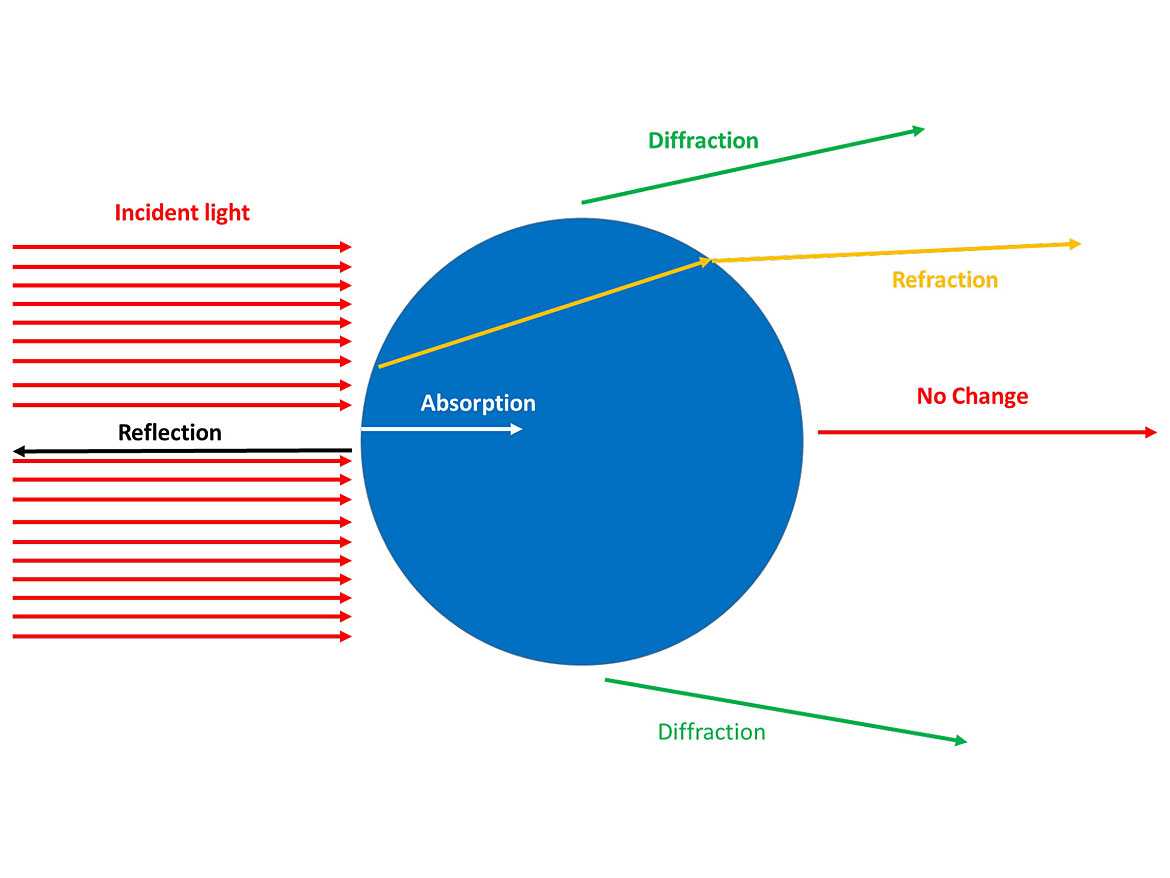
Light scattering through diffraction falls into several theories. Raleigh, Fraunhofer and Mie scattering are when light interacts with a small spherical particle. Mie theory covers spherical scattering independent of particle size, and while much more complicated, it is easily calculated by current computers, so Raleigh and Fraunhofer approximations are no longer necessary. When an electromagnetic wave (light, for example) encounters an object, obstacle or other non-homogeneity in the media, the result is the scattering of light. When the wave interacts with the particle, the orbits of the electron cloud of the particle are disturbed. This change in the electron cloud has the same frequency as the incident electromagnetic wave. The oscillation of the disturbed electron cloud leads to an induced dipole moment that emits radiation, the majority of which has the same frequency as the incident electromagnetic wave. In the case of pigments, the incident electromagnetic wave of concern is visible light. This scattering of light is unique because it is not electromagnetic waves bouncing off the surface, but an interaction of the light and the particle.
One unique class of pigments are fluorescent pigments. They absorb wavelengths outside the visible range (such as ultraviolet) and re-emit light in the visible range. The ratio of scattered light to undisturbed incident light depends on many factors including the refractive index of both the particle and media around the particle, size of the particle, wavelength of incident light, angle of observation, or polarization of the incident light. Mie theory calculations do have limitations though — the particle must be spherical, smooth and optically homogeneous.
Unfortunately, the term ‘absorbance’ is often misused for all scattering phenomena, and instead, ‘extinction’ should be used. Extinction is the cumulative effect of absorbance and other scattering, resulting in complete loss of light within the film. For example, the extinction method for blue is mostly diffraction, while the extinction for carbon black is mostly due to absorption.
As the refractive index difference between the media and particle increases, the amount of refraction increases. The apparent bending of an object (such as a straw as it enters water) is an example of refractive index differences of water and air. Most resins used in coatings have a refractive index of around 1.4 - 1.6, versus 1.33 for water and 1.03 for air. The greater the absolute difference from this value, the greater the bending of light and the greater the change in appearance. Rutile titanium dioxide has a refractive index of 2.7. This difference explains how this pigment interacts with light and produces greater hiding than anatase titanium dioxide with a refractive index of 2.5. Since titanium dioxide emits all visible light frequencies equally, we see it as white.
For the refractive index, the difference is truly absolute. Air voids in dried paint will also have refractive scattering, which leads to opacity, due to the 0.4-0.6 lower refractive index of air versus resin. This “dry hiding” is used in inexpensive flat paints when you are above critical pigment volume concentration (CPVC) to lower primary pigment cost. In this situation the resin does not fully coat all the pigment in the formula. The disadvantage is that performance properties of the coating drop off dramatically once you exceed CPVC.
With three-dimensional particles, all five interactions between light and the particle take place. Which one dominates and how much interaction takes place is based on the relative size of the particle compared to the wavelength of the incoming light, the angle of observation relative to the angle of incident light, and the transparency of the particle.
As the diameter of the particle approaches the wavelength of the incident light, absorption and refraction become increasingly more important. Many smaller particles generally have better light scattering than fewer large particles due to increased light/particle interactions as the light goes through the film. There is a point where the particle becomes too small and there is little light/particle interference, resulting in loss of opacity.
All information contained herein is provided "as is" without any warranties, express or implied, and under no circumstances shall the author or Indorama be liable for any damages of any nature whatsoever resulting from the use or reliance upon such information. Nothing contained in this publication should be construed as a license under any intellectual property right of any entity, or as a suggestion, recommendation, or authorization to take any action that would infringe any patent. The term "Indorama" is used herein for convenience only, and refers to Indorama Ventures Oxides LLC, its direct and indirect affiliates, and their employees, officers, and directors.
Looking for a reprint of this article?
From high-res PDFs to custom plaques, order your copy today!