
Micas are sheet silicates, historically significant for their ability to be split into large, thin sheets that are uniquely useful for their electrical, thermal and mechanical properties. They have high electrical and thermal insulating properties, are resistant to chemical attack, can be split into transparent or optically flat films, and can be cut or stamped to shape. Most mica used today, however, is in ground form, although the mineral's platy nature, inertness and optical properties are still primary attributes. There are two commercial forms of mica: muscovite, a potassium aluminum silicate KAl2(AlSi3O10)(OH)2, and phlogopite, a potassium magnesium aluminum silicate KMg3(AlSi3O10)(OH)2. The coatings industry uses muscovite nearly exclusively, primarily because it has better color. Compared to platy talc, ground mica plates are generally higher in aspect ratio, more flexible, more hydrophilic, higher in binder demand and inferior in color. Because mica plates are generally stronger and less brittle than the particles of certain other high aspect ratio fillers, such as talc, clays, and glass flake, they are more resistant to breakage and reduction in aspect ratio under high-intensity mixing or milling.

Structure
Phlogopite is a trioctahedral mica with a structure similar to that of talc (see “Talc: Form Follows Function,” PCI January, 2003). The more commonly used muscovite is the pyrophyllite-like dioctahedral analogue, with two of every three octahedral positions filled by Al3+, with the third vacant. For both phlogopite and muscovite about one of four tetrahedral Si4+ is replaced by Al3+. The resulting charge imbalance is compensated for by K+ located between the opposing hexagonal openings in the silica sheets of adjacent platelets. The structure of muscovite is illustrated in Figure 1.
Mica is well-known for its ready delamination, even in the form of large sheets. This is due to the relatively weak bonding effect of the univalent counterion. Figure 2 is a photomicrograph of muscovite plates.
Mica Grades
Fillers
Most filler and pigment grade micas are derived from flake mica, which is collected by the centrifugation and/or flotation of mica ores from which quartz and feldspar are also recovered, or as a floated byproduct during the beneficiation of feldspar, kaolin, or lithium-bearing ores. Dry-ground mica is made by milling dried flake mica concentrates. Coarse-milled products (>100 mesh), used mainly for oil well drilling muds and asphalt roofing, are processed with hammer mills and screens or air separators. Fine-ground products, -100 mesh to -325 mesh, are processed in fluid energy mills, usually with superheated air. Micronized mica is milled to -20 or -10 micrometers in fluid energy mills using superheated steam. Wet-ground mica is produced from flake mica concentrates containing 25% to 35% moisture using Chaser or Muller mills. These mills are designed to effectively delaminate the mica flakes, providing products with optimized aspect ratio, sheen and slip.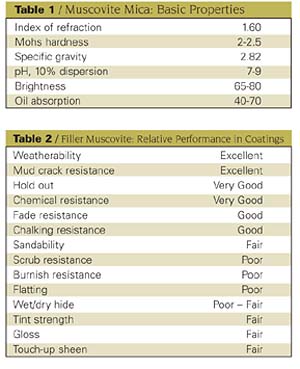
Pigments
Wet-ground, high aspect ratio mica is used as-is or coated with metallic oxides to form nacreous pigments. Nacreous pigments are thin transparent platelets of high refractive index, which partially transmit and partially reflect light, imparting a pearlescent or metallic effect. The pearlescent effect is due to specular reflection from the broad surfaces of the transparent platelets and the parallel orientation of those platelets at various depths within the binder film. Light transmitted through platelets near the film surface is partially reflected by deeper platelets. A pearly luster is produced by the dependence of reflection on viewing angle and the sense of depth created by reflection from many layers.White nacreous pigments are made by forming a uniform coating of TiO2 on delaminated mica. The mica serves as a transparent template so that the high refractive index TiO2 can assume the required platy shape. Pearlescence is optimized when the pigment’s optical thickness (platelet thickness times refractive index) is in the 100nm (blue white) to 140nm (yellow white) range. Thicker platelets are used to make interference colors. Light interference is created by interaction of the reflections from the upper and lower surfaces of the platelet. A reflection maximum occurs at the wavelength of light for which these two reflections are in phase. A reflection minimum occurs at the wavelength for which they are exactly out of phase, canceling each other out. A transparent, colorless nacreous pigment platelet acts as a filter, separating light into two components: the reflected color and the complementary transmission color.
When coated on a white surface, the nacreous pigment provides a two-tone metallic effect. The reflected (specular) color is seen as a highlight. The background color at nonspecular angles is created by the diffuse reflection (from the white surface) of the transmission color. The complementary reflection/transmission colors are a function of pigment’s optical thickness, ranging in a continuous spectrum from blue-white/yellow-white at 100nm, to magenta/green at 250nm, to green/red at 370nm.
The color effects of nacreous pigments are supplemented by over-coating the TiO2-mica platelets with a thin transparent film of a light absorbing colorant, e.g., iron oxide for yellow, ferric ferrocyanide for blue, chromium oxide for green, and carmine for red. The combination pigments thus produced can have the same reflectance and absorption colors, or they can be two different colors. When the colors are the same, it is seen from all viewing angles, with bright (metallic) highlights provided at specular angles. With different colors a two-color effect is achieved. Combination interference/absorption pigments are made in the gold to red range by coating Fe2O3 directly onto mica.
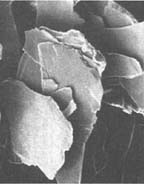
Properties and Uses
The United States is the leading supplier of ground mica, producing about 112 kilotons/year, which is roughly 40% of world production. The U.S. produces only ground muscovite, and imports its phlogopite needs from Canada and Finland. Approximately 85% of U.S. ground mica output is dry-ground. Nearly 45% of domestic dry-ground mica is used in joint compounds where fluid energy milled muscovite contributes to consistency and workability, smooth surface finish, and resistance to shrinkage and cracking. The second largest use of dry-ground mica, around 30%, is in coatings. The primary use of wet-ground mica, unmodified or as nacreous pigment, is in automotive paint.The basic properties of muscovite mica are given in Table 1. Mica does not delaminate quite as readily as talc, so it is somewhat harder, although still softer and less abrasive than most other fillers, minimizing its contribution to abrasion resistance. Its high aspect ratio and platy shape provide excellent film reinforcement, but at the expense of optical properties. The relative performance of mica as a coatings filler is given in Table 2.
Fine-ground and micronized mica is used as a functional filler in a variety of coatings formulations. As a platy mineral, it has beneficial rheological properties in liquid coatings, and also helps reduce sagging once the coating is applied. Mica reinforces the paint film during drying and curing, and as the film ages it reduces the internal stresses from oxidation, thermal expansion, and contraction. Mica increases the film's flexibility and reduces cracking and checking, resulting in improved adhesion and better weathering. Film reinforcement therefore accounts for mica’s utility in a range of coatings, from texture paints to exterior coatings.
Because its flakes align parallel to the substrate, mica is used in roof coatings, exterior wood stains and exterior paints to control moisture and gas permeability, and to reduce the penetration of light into the coating film. This makes the film more resistant to fading, weathering and UV degradation. Mica’s barrier properties are also exploited in primers, anti-corrosion paints and marine coatings. The ability of mica plates to overlap and to bridge openings is used for penetration control in sealers for porous surfaces. Other typical filler uses are in floor and porch paints, heat-resistant paints, and traffic paints.
Mica-based pigments are commonly used in powder and automotive coatings to impart pearlescent or metallic effects. These pigments are available with silane or other compatibilizing coatings to maximize pigment-resin adhesion. To avoid pigment fracture and subsequent loss of optical effect, delaminated mica and nacreous pigments are incorporated into powder coatings using a method different from the usual compounding/extrusion/milling procedure. The preferred method of incorporation is to carefully dry blend the finished powder coating with the pigment. The bonding method uses the powder resin’s softening point to create a tacky environment for pigment attachment.
Conclusion
Filler grade mica is available in a variety of grades, from coarse dry-ground to micronized to highly delaminated, offering a range of aspect ratio, particle size, color and cost to suit the reinforcement and barrier requirements of most coatings applications. Mica is also uniquely suited to the production of pearlescent and metallic effect pigments because of the high aspect ratio and optical clarity of its delaminated plates.For more information on mica, contact R.T. Vanderbilt Co. Inc., phone 800/243.6064; fax 203/853.1452; e-mail pciullo@ rtvanderbilt.com or srobinson@rtvander_bilt.com; or visit www.rtvanderbilt.com.
Bibliography
Braun, Jergen. H. Introduction to Pigments, FSCT, Blue Bell, PA, 1993.Carr, D.D. (Ed.), Industrial Minerals and Rocks, 6th Ed., Society for Mining, Metallurgy, and Exploration, Inc., Littleton, CO, 1994.
Ciullo, P.A., (Ed.) Industrial Minerals and Their Uses, Noyes Publications, Westwood, NJ, 1996.
Hare, Clive et al. “Extenders.” PCI, March 2001.
Hawley, George C. “White Opacifiers in Paint.” PCI, March 2001.
Hedrick, J.B., Mica, Minerals Yearbook, USGS, Reston, VA, 2001.
Hertz, Sanford. “A Guide to Selecting Mica.” APCJ, January 13, 1999.
Hurlbut, C.S., Klein, C., Manual of Mineralogy, 21st ed., Wiley, NY, 1993.
Lavallee, Carolyn et al. “Surface Treatment Effects.” PCI, May 2002.
Lewis, P.A. (Ed.), Pigment Handbook, John Wiley & Sons Inc., NY, 1988.
Madson, W.H., White Hiding and Extender Pigments, Unit 7, Federation of Societies for Paint Technology, Blue Bell, PA, 1967.
Rochard, Stephane. “Using Special Effect Pigments in Powder Coating Systems.” PCI, January 2002.
Tolle, Thomas. “The Selection of Extender Pigments for Use in Interior Wall and Exterior Flat House Latex Paints. Burgess Pigments Coatings Society Presentation. Date unknown.
Wosniak, Mark. “Overview of Extenders: Basic Properties, Types and Processing.” FSCT Extender and Filler Training Course. November 1998. Orlando, FL.