Effect of Polyether Monoamine Structure on Pigment Dispersant Properties

In an ink formulation, the pigment must be dispersed and stabilized to achieve good color strength, gloss, transparency and transfer efficiency. Stable, concentrated dispersions with small particles and narrow particle size distribution potentially can lead to higher gloss and color strength per unit mass of pigment. The push towards waterborne formulations and increasing pigment loading underlines the need for good dispersion and stabilization properties.
In this study, the uses of polyether amines (PEAs) as dispersants for carbon black, as well as their physico-chemical properties, were studied systematically. A series of polyether amines was prepared by varying the molecular weight and the ethylene oxide/propylene oxide ratio. By understanding the effect of each portion of the molecule on the physical properties and performance, new dispersants can be developed. A variety of different techniques have been used in this study to characterize these properties. Equilibrium surface tension and solution depletion methods were used to determine the adsorptions at the air-water interface and carbon black-water interface, respectively. Dynamic interfacial tension measurements have been made using mineral oil as a dispersed phase. Contact angles of water on carbon black dispersions were measured to provide information about wettability. The performance of these materials in a carbon black ink formulation, including rheology, stability and color transfer efficiency, was also studied.

Experimental
Polyether amines (PEAs) contain primary amino groups attached to the terminus of a polyether backbone. The polyether backbone is based either on propylene oxide (PO), ethylene oxide (EO) or mixed EO/PO. Polyether amines were prepared by reaction of a methanol initiator with ethylene and/or propylene oxide, followed by conversion of the resulting terminal hydroxyl group to an amine by reacting with ammonia over a heterogeneous catalyst. In this work, a series of polyether amines with different degrees of ethoxylation, propoxylation and various molecular weights were made and studied systematically. The chemical structure of the polyether amines used in the present study is
CH3-[OCH2-CH2]x-[OCH2CH(CH3)]y-NH2
where x varies from ~5 to 60 and y varies from ~2 to 45.
The PEAs used in the present study are given in Table 1, along with their properties. The molecular weights listed are theoretical values. The first number designates the molecular weight and the last two numbers designate the weight percent of EO. For example, PEA 270 represents a polyether amine with 2,000 molecular weight and 70% EO.
The HLB values are calculated based on the structure of each molecule.1 In this method, a number from 0 to 40 is assigned to the surfactant based on the balance between the hydrophobic and hydrophilic portions of the molecule. For the nonionic molecules in the present study, the relationship used to calculate the HLB values is given by
HLB = 20 x MH/(MH + ML)
where MH is the formula weight of the hydrophile and ML is the formula weight of the lipophile. HLB values give a measure of the surfactant's solubility in aqueous solution. Water solubility in general increases with increasing HLB value.
Surface tension measurements were determined with a Kruss K12 tensiometer using the Wilhelmy plate method. Basically, a clean platinum plate with exactly known geometry is brought in contact with liquid, and the force acting on the plate is measured via a microbalance. The surface tension of the liquid is calculated from the measured force. Dynamic interfacial tension (IFT) measurements were made using a Kruss DVT-10 drop volume. The instrument measures dynamic IFT as a function of surface age.
The surface energy of carbon black was estimated from the contact angles of water and methylene iodide on carbon black. The contact angles of carbon black in water and methylene iodide were measured with a Kruss K12 tensiometer using the Washburn's method. Basically, about 1 gram of carbon black was placed in a powder holder obtained from Kruss (FL12) and the amount of fluid adsorbed by carbon black was measured as a function of time. Hexane was used as a solvent to calculate the capillary constant, and once the capillary constant is known the contact angles of water and methylene iodide on carbon black can be determined.
Contact angle measurements of water on a clean glass slide coated with a carbon black dispersion were made using a Kruss K-12 tensiometer. The coated glass slide was air-dried for 36 hours prior to contact angle measurements.
The carbon black was obtained from Cabot Chemical Corporation. It has a particle size of 24 nm and a surface area of 112 m2/g, which was given by the supplier. Carbon black suspensions (C=0.45% w/w) were prepared at room temperature in the presence of polyether amine solutions of different concentrations. The dispersion was obtained by treatment in an ultrasonic bath for 30 minutes and followed by rotating the samples end over end for 18 hours to reach equilibrium. Each suspension was centrifuged at 7,000 rpm for 35 minutes to sediment all the solids, leaving a clear supernatant at the top. The concentration of the remaining polyether amine in the solution was determined using a calibrated surface tension curve, and the amount adsorbed was estimated from the difference between the amount of polyether amine added and the amount remaining in the supernatant.
All of the dispersions contained 34.8% carbon black with dispersant-to-pigment ratio of 4.1% and pigment-to-binder (a styrene acrylate copolymer) ratio of 4.4%. Initially, a premix was made by weighing water, binder, defoamer and dispersant into a blender container and mixing for 20 seconds. The pigment was then added in three equal parts, followed by mixing after each addition. After incorporating all the pigment, the premix was blended for 10 minutes to deflocculate the pigment. The formulation was then introduced to an Eiger Mini-100 horizontal media mill. The mill contained about 75 g of 0.8 mm YTZ media, and was operated at a speed of 5,000 rpm. Each sample was milled for 10 minutes.
Waterborne flexographic printing inks were also made from the dispersions by combining 17.5 g of dispersion with 32.5 g of masstone vehicle, which contains acrylic emulsion (68.42%), styrene acrylic resin (26.32%) and water (5.26%). This yields inks with 12.2% pigment loading. Using a Pamarco hand proofer fitted with a 550 line ceramic laser-engraved anilox with 2.8 bcm capacity, the inks were printed side by side on Leneta 3NT-3, 7 mil polyester film and foil (Leneta Alu-Card) and compared for color density, gloss and transparency. Brookfield viscosities of the inks were also measured.
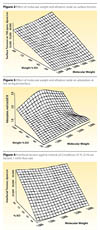
Results and Discussion
Adsorption At Air-Liquid InterfaceSurface tension curves for various PEAs are shown in Figure 1. Since PEA 213 is water insoluble, it was excluded. These PEAs do not show a distinct critical micelle concentration (cmc) even though they are surface active. Unlike conventional surfactants like sodium dodecyl sulfate (SDS), some of these materials reveal three breaks. For example, PEA 183 shows the first break (C1) at 100 ppm, second break (C2) at 800 ppm and third break (C3) at 10,000 ppm . It is likely that this curve is the result of the broad molecular distribution of the compound. A similar trend was also observed for the EO/PO/EO block copolymer.2 Most surface-active material begins to aggregate in the solution at C1, while the less surface-active material is not forming micelles and is increasing with the bulk concentration. It is only at C3 where all the material form micelles. Literature review indicates that the concentration C3 is very sensitive and shifts with increasing temperature to smaller concentrations, while C1 is not so temperature dependent.
PEA 320 and PEA 120 exhibited lower surface tension than the others and this can be due to much lower HLB values. Figure 2 depicts the dependence of PEA surface tension measured at 100 ppm on molecular weight and weight % of ethylene oxide. It can be seen that the surface tension decreases with decreasing ethylene oxide. Molecular weight has a slight effect on surface tension, being more pronounced at high ethylene oxide content.
The concentration of polyether amine adsorbed at the air-liquid interface, Γ, can be determined by applying the Gibbs adsorption equation to the surface tension results:
Γ= -(dγ/d ln C)(1/RT)
where γ and C are the surface tension and concentration of polyether amine, respectively. R is a gas constant and T is the absolute temperature. From the equation, Γ can be obtained from the slope of a plot of γ versus ln C. As can be seen in Figure 3, the adsorption increased with increasing ethylene oxide and exhibited a maximum at 2,000 molecular weight.
Adsorption At Liquid-Liquid Interface
Dependence of interfacial tension on molecular weight and ethylene oxide against mineral oil is shown in Figure 4. Similar to surface tension measurements (Figure 2), interfacial tension decreases with decreasing ethylene oxide and molecular weight has little effect on interfacial tension.
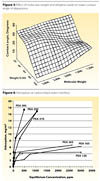
Contact angle provides information about wettability. A low contact angle indicates that a dispersant is effective at wetting the carbon black particles, making them more hydrophilic and easier to disperse. Figure 5 shows the effect of molecular weight and ethylene oxide on contact angle. It appears that the contact angle decreases with increasing ethylene oxide, and molecular weight has a less pronounced effect. From the contact angles of water and methylene iodide on carbon black, we also determined the surface energy of carbon black. Details for calculating a surface energy of a solid are given elsewhere.3 The total surface energy of carbon black is about 31.8 dyne/cm, which is a sum of dispersion force (28.0 dyne/cm) and acid/base (polar) force (3.8 dyne/cm).
Adsorption At Solid-Liquid Interface
Figure 6 shows the adsorption of various PEAs on carbon black. It appeared that the amount increased with increasing ethylene oxide and molecular weight.

Ink Formulations
The amount of work done on milling a premix can be ascertained from the ampere draw experienced on the mill during processing. Additionally, a rise in sample temperature is an indication that high shear forces are being generated during dispersion, often leading to better dispersions with higher color development. The dispersant that promoted this behavior the most was PEA 295. Fluidity during dispersion is also an important indicator of how well a dispersant functions in a given formulation. PEA 385 and PEA 295 were found to exhibit the most fluidity. Alternatively, a high degree of thixotropy is often an indicator of incompatibility of the dispersant with the formulation. Such behavior leads to low shear forces generated in the mill, poor rheology and poor color strength development. The most pseudoplastic formulas were those containing PEA 213 and PEA 120. We attempted to measure the tint strengths and the results indicated that none of the dispersants yielded a significant color strength advantage over any of the others. The purpose of evaluating tint strength is to ascertain the amount of color generated during milling.Figure 7 shows the initial viscosity of inks, and the results indicated that all of the dispersants gave similar dispersion viscosities and all the dispersions were fairly pseudoplastic. The aged data (Figure 8) indicated that PEA 213 had yielded a higher degree of pseudoplastic character to the ink over time. The other dispersants demonstrated similar viscosity behavior. The latter part of the curve for PEA 295 appears to be an aberration.
Print densities of the inks were also measured and averaged. Figure 9 summarizes the results. It can be seen that color density increases with increasing ethylene oxide, and molecular weight has little effect. What the data indicate is that color strength differences are not attributable to higher/lower viscosity, nor are they attributable to increased color development in the dispersion step. It is interesting to note that the adsorption data and contact angle measurements correlated well with color density data, in which the performance (adsorption, wettability and color density) increased with increasing ethylene oxide and with molecular weight to a lesser extent. Most likely, the differences seen in color density are indicative of better ink transfer or better color retention and thus better flocculation stability afforded by the dispersant. Interfacial tension and surface tension measurements are not a good predictor of color strength performance nor stability.
Dispersions containing PEA 213, PEA 120 and PEA 320 separated after a couple of weeks while the others are still stable at room temperature. The separation was due to poor adsorption of these dispersants at carbon black/water interface and high contact angles (poor wettability). In addition, low HLB values may also be a factor.
Effect of HLB
The HLB value is calculated from an empirical equation as shown in the Experimental Section. The HLB concept has proved useful to provide guidance as a first selection of surfactant for a given application. For example, an emulsifier for a water-in-oil emulsion should be hydrophobic with an HLB of 3-6; an emulsifier for an oil-in-water emulsion should be in the HLB range of 8-18; and a dispersant for carbon black should be in the HLB range of 10-12.4 However, the HLB concept does not indicate the concepts of surfactant or dispersant packing and the adsorption layer thickness at the interface. In this study, when the HLB is below 5, the dispersion was unstable. Two surfactants or dispersants having the same HLB can behave differently at the interface. For example, PEA 183 and PEA 385 have a HLB of about 17; however, the latter showed a much stronger adsorption at the interface, better wetting and higher color density than the former.Conclusions
The following conclusions may be drawn.1. Adsorption, contact angle and color density measurements indicate that a polyether amine having a high ethylene oxide content and high molecular weight provides the best performance.
2. Surface tension and interfacial tension values decrease with decreasing ethylene oxide. Molecular weight has little impact on surface tension and interfacial tension.
3. Low HLB dispersants (below 5) exhibit poor dispersion stability.
4. All of the polyether amines tested made fairly pseudoplastic inks, and the ink viscosities are comparable.
5. Tint strengths are very much the same for dispersions using these polyether amines.
Looking for a reprint of this article?
From high-res PDFs to custom plaques, order your copy today!