The Effect of Surfactant Selection on Emulsion Polymer Properties
Surfactants have a strong effect in reducing the surface tension between the water and the air, as well as the interfacial tension between the water and otherwise immiscible liquids such as vinyl monomers. The practical fact that commercial emulsion polymerizations can be run smoothly and quite rapidly with many water-insoluble monomers is due to the presence of surfactants. Normally, surfactants are used in concentrations well above their critical micelle concentration (CMC). At such practical concentrations, surfactants form micelles, which are entities capable of “solubilizing” non-polar substances like organic monomers.
A critical issue in commercial latex manufacture is their stability during and after production. As mentioned, surfactants have an effect on overall latex stability. Thus, the appropriate surfactant selection is an important consideration when designing a latex formulation. Anionic and nonionic are the most effective and widely used surfactants in emulsion polymerization. While anionic surfactants prevent coagulation due to electrostatic repulsions, nonionic surfactants prevent coagulation due to steric stabilization. Anionic surfactants prevent coagulation by electrostatic repulsions originated from the anionic charges adsorbed on the polymer particles and their associated double layers. And nonionic surfactants, especially polyethoxylates, prevent coagulation by spatial or steric stabilization. Some specialty surfactants, such as fatty alcohol ether sulfates, Disponil® FES, combine both, electrostatic and steric stabilization in their molecules.
It is also well known that the amount and chemical structure of surfactant/s used in emulsion polymerization have a strong influence on conversion, particle size and distribution, viscosity, overall latex stability, and cleanness. Surfactants also have an effect on final properties such as film formation, adhesion, wet ability, water resistance and foamability. While the typical surfactant concentration is above the critical micelle concentration (CMC), there are some rules of thumb that work well as a guidance. Surfactants are used in the range of a few tenths of percent to 5%. Typically, however, the concentration of anionic surfactants would be between 0.5% and 3% based on the monomer if used as the primary surfactant; and between 0.5% and 2% if used in combination with a nonionic surfactant. Nonionic surfactants are rarely used alone because of their tendency to give larger particle sizes, mainly due to their lack of charge. If there is a need to do so, their concentrations are normally higher, typically 5%. However, even a small percentage of an anionic surfactant used in combination with a nonionic surfactant would be sufficient to reduce the particle size. In order to achieve a desirable balance of properties, most commercial emulsion polymers are made using appropriate combinations of anionic surfactants for particle size control and electrostatic stabilization, and nonionic surfactants to enhance mechanical, electrolyte, freeze thaw and thermal stabilities.9,10
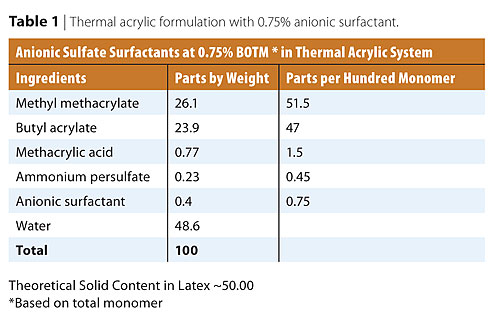
Experimental
PolymerizationSemi-continuous polymerization was used to produce the acrylic latexes for this study. A polymer seed was formed adding 2% monomer pre-emulsion at the beginning of the reaction, the remainder being added according to a predetermined schedule during the course of the polymerization. The benefit of using a seeded emulsion polymerization is to produce a narrower particle size distribution. The slow addition of monomers also results in a more uniform copolymer composition. During the seed stage, particles are nucleated and allowed to grow until the monomer is completely converted. The addition of the remaining monomer and surfactant provided growth of the particles formed during the seed stage. No new particles are expected to form during the addition of the remaining monomer and surfactant.
The formulation used for this study is shown in Table 1. The initial charge was heated to 80 ºC while purging with nitrogen under continuous mechanical stirring (200-300 RPM). A nitrogen blanket was maintained throughout the run. At 80 ºC, a 25% initiator solution and 2% monomer emulsion were added. The reactor temperature was held at 80 ºC for 15 minutes to allow for seed formation. The remaining monomer emulsion and initiator solution were fed into the polymerization reactor over 3 hours. After this period, the temperature was increased to 85 ºC and held for 30 minutes. The reactor was then cooled to room temperature, and the pH adjusted to 9.
The surfactant concentration was held constant at 0.75% by weight based on 100% monomer. The monomer composition, consisting of 51.5% by weight methyl methacrylate, 47.5% butyl acrylate, and 1.5% methacrylic acid, forms a hard, brittle polymer, giving the final film its water-resistant qualities. Butyl acrylate is added to soften the film, making it flexible and scrub resistant. Methacrylic acid enhances stability and adhesion, as well as providing available acid functionality. A nitrogen blanket was used to expel the oxygen from the reactor, as O2 retards the polymerization reaction. Three different anionic Disponil surfactant chemistries were chosen for this study. Alkyl sulfates (AS), FES series of Fatty Alcohol Ether Sulfates (FAES), and AES series of Alkyl Phenol Ether Sulfates (APES).
Light Scattering Particle Size Analysis
The latex particle sizes were determined using a Nicomp Submicron Particle Size Analyzer Model 370 (Hiac/Royco Instruments). The mean diameter particle sizes and the percent standard deviations, which are a measure of the particle size distributions, generated during polymerization are given in nanometers.Atomic Force Microscopy Particle Size Analysis
A Nanoscope IIIa Scanning Probe Microscope (Digital Instruments) was used to image the samples. The AFM was used in both, contact and tapping mode, the latter to minimize the tip-film interaction. Analysis was performed using Digital Instrument software provided with the AFM; the images to be presented were processed with only moderate contrast enhancement. AFM was conducted on films subjected to various conditions, including different drying times and after annealing to temperatures above the observed phase transitions. AFM images of several films were cast on both steel (roughness approximately 5 nm) and mica substrates (sub-nanometer roughness). The AFM study was conducted at Drexel University.12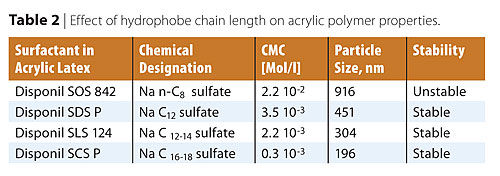
Results
Effect of Hydrocarbon Chain Length - Hydrophobic CharacteristicsTable
2 presents the properties of
acrylic latexes made with alkyl sulfate (AS) surfactants. The homologue series
of hydrophobes were chosen to show contrasting effects on micelle formation,
between a low-molecular-weight hydrophobe such as n-octyl (C8),
and a very large-molecular-weight hydrophobe such as a cetyl-oleyl
(C16-18). In the AS hydrophobe homologue
series represented by CnH (2n+1)
OSO3 Na, while the tendency to micelle
formation is virtually nonexistent at n = 8, such tendency is strong in the
range from n = 12 to n =18. Above n =18, the hydrophobic group has a more
predominant effect on the molecule, which becomes insoluble and difficult to
handle, exhibiting less surfactant-like properties. Table 2 shows a decrease in
CMC values with increasing hydrocarbon chain length. The trend is a clear
indication of the increase in micelle formation efficiency and monomer
solubilization.
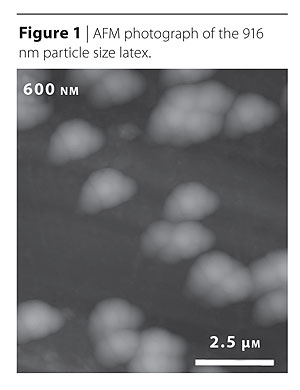
The micelles of surfactants with shorter hydrocarbon chains, i.e. relatively less hydrophobic character, have lower solubilization power to monomers than those with larger ones. They also exhibit less tendency to form micelles. Indeed, the solubilizing power of surfactants increases as the hydrocarbon chain length increases. This solubilizing capability is reflected in a significant increase in micelle formation efficiency in the range from n = 12 to n =18. The micelle formation efficiency is the result of smaller sized latexes with typically superior stabilization for n values in the range of n = 12 to n =18. It is also important to point out the higher efficiency shown by hydrocarbon chains with broad distribution.

Effect of Ethoxylation Level in FAES and APES - Hydrophilic Characteristics Fatty Alcohol Ether Sulfate Series
One of the most interesting properties of fatty alcohol ether sulfates (FAES), is the combination of a non-polar hydrophobic group, a strong anionic hydrophilic group, and a less hydrophilic polyoxyethylene chain susceptible to hydration. Table 3 shows the effect of the ethoxylation chain length (EO level) on the acrylic polymer physical properties. The FAES study was done with surfactants bearing the same hydrophobe, a C12-14 fatty alcohol. The only difference was the molecular weight of the polyethylene oxide chain ranging from 2 to 50 moles of ethylene oxide.The AS Disponil SLS 2010, an AS without ethoxylation, was used as a reference. It is interesting to note that the particle size exhibited a minimum at the 2-4 moles of ethylene oxide level. For nonionic adducts of ethylene oxide, an increase in ethoxylation results in an increase in CMC, a decrease in micelle formation efficiency, and an increase in the surface tension (ST) at their respective CMCs. The opposite has been observed in the FAES series. The CMC of FAES decreases from 0-4 EO moles, reaching a minimum at 4 EO moles. Above 4 EO moles, little or no change is observed. The observed decrease in CMC was coupled with a decrease in surface tension at the corresponding CMC’s.13,14 Table 3 and more clearly Figure 2 show a minimum in particle size in the range of 2-4 moles of EO. This sharp decrease in the mean particle size seems to correlate well with the decrease in CMC.
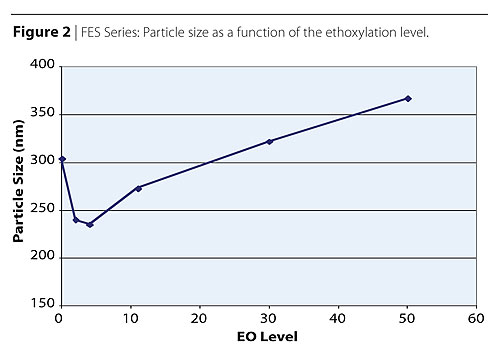
These results indicate the importance of selecting the appropriate ethoxylation
level to obtain desirable polymer particle sizes and distributions as well as
desirable physical properties to target specific application performance.
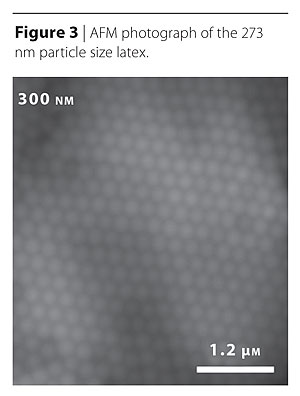
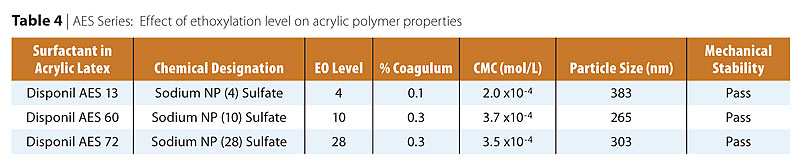
Alkyl Phenol Ether Sulfate Series
Table 4 and Figure 4 show the effect of the ethoxylation chain length (EO) on the acrylic polymer physical properties and particle size respectively. APES alkyl phenol bearing surfactants are being replaced by more environmentally acceptable fatty alcohol ether sulfates such as FES. The APES study was done with surfactants bearing nonyl phenol hydrophobe. The only difference was the molecular weight of the polyethylene oxide chain ranging from 4-28 moles of ethylene oxide. Similarly to the FAES series, a minimum in particle size was found, in this case at 10 EO moles. Similarly to the FAES series, the minimum in particle size corresponds to a maximum in micelle formation efficiency. In this case, the optimum balance of hydrophobic and hydrophilic properties within the surfactant molecules seems to be at 10 EO moles. The reasoning for the rapid increase in particle size above 10 EO moles is the same as previously discussed with the FAES. It is interesting to notice that the particle sizes obtained with AES are larger than those obtained with FAES bearing similar ethoxylation level. The results suggest a stronger effect of the EO chain length on the particle size than on the CMC values.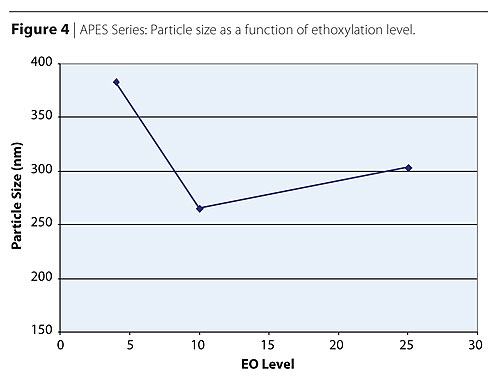
Conclusion
This paper presents the effect of surfactant molecular structure on model acrylic polymer properties. The effect of the hydrophobe structure, as well as the effect of the polyoxyethylene chain length, were explored with three different anionic surfactant chemistries: Alkyl Sulfates (AS), FES series of Fatty Alcohol Ether Sulfates (FAES) and AES series of Alkyl Phenol Ether Sulfates (APES). The AS surfactants used in this study gave the largest and the smallest particle sizes. In the AS study, it was found that surfactants with shorter hydrocarbon chains exhibit less tendency to form micelles. In contrast, the higher solubilizing capability of larger chains in the range of C12 to C18 yielded a significant increase in micelle formation efficiency and higher polymer stabilization. In the FAES and APES study, it was found that as the ethoxylation level increased the mean particle diameter decreased, up to an EO level where the hydrophilic character of the surfactant became more predominant causing an increase.This paper was presented at The Waterborne Symposium, Advances in Intelligent Coatings Design, February 2007, New Oleans, LA. The Symposium is sponsored by The University of Southern Mississippi School of Polymers and High Performance Materials.
References
1. Harkins, W.D. J.Am. Chem Soc. 1947, 69,1428.
2. Smith W.V.; Edwards, R.H. J.
Chem. Phys. 1948, 16,592.
3. Smith. W.V. J. Am. Chem. Soc. 1949, 70,3695, 1948, ibid. 71,4077.
4. Blackley D.C. Emulsion Polymerization. Applied Sci. Publishers
Ltd., 1975
5. Gilbert G.G. Emulsion Polymerization. Academic Press,
1995
6. Piirma I. et.al. J. Polym. Chem. 1982, 20, 489.
7. Piirma et.al.Polym. Bulletin, 11, 497, 1984.
8. Medvedev, S.S. et.al.
J. Macromol.Sci. Chem. 1973, 7,715.
9. Okamura, S. et. al. J.
Polyms. Sci. 1962,
58,221.
10. Fernandez, A.M, et. al. International Waterborne Symposium, 1999.
11. Fernandez, A.M., Marie Esther Saint Victor et.al. FSCT, 2004.
12. Twardowski, T., Gentile, E., Brennan, D., Guzman, M., DiNardo, J.,
Fernandez A.M. International Waterborne Symposium, 2001.
13. Schwuger, M.J. ACS Symposium series253, 1984.
14.
Rosen, M. Surfactants and Interfacial
Phenomena,
John Wiley, 1989.
Looking for a reprint of this article?
From high-res PDFs to custom plaques, order your copy today!