Surface-Treated Metallic Pigments
Metallic effects in coatings have continued to grow in popularity, driven initially by the trend over the past few decades for metallic-effect car paints that has culminated in silver being a dominant colour in the automotive industry for the past six years. Recent signs are that other shades of metallic effects in the automotive industry are now coming to the forefront including metallic greys, blues and blacks. This popularity of metallic effects has spread to many other coatings areas, and the manufacturers of metallic-effect pigments have responded by continuing to push forward with the development of new and improved metallic-effect pigments.
The most immediately visible area of use for metallic pigments in coatings is in the area of automotive coatings, where metallic effects have accounted for as high as 60-70% of new cars over the past decades. Metallic silver comprised as much as 37% of new car registrations in certain areas over the past six years. However, metallic effects are becoming more apparent in many other areas as the technology of metallic-effect pigments has continued to evolve to meet other demanding applications. Metallic effects can be seen today in:
• other transportation coatings - motorcycles, buses, lorries;
• decorative coatings - emulsion paints, wallpapers;
• electrical and electronic coatings - mobile phones, laptops, LCD TVs;
• construction coatings - building claddings, window profiles;
• packaging - shampoo bottles, bags;
• printing inks - cigarette packaging, labels, confectionary;
• plastics - sports goods, toys, oil bottles; and
• cosmetics - nail polish, lip gloss, eye shadows.

Aluminium Pigment Production
To understand why metallic pigment technology has been required to continually advance to meet the requirements of new coatings applications, it is first necessary to understand some of the basics of metallic pigment technology and, in particular, what makes aluminium pigments unique in their appearance and properties.The basic process is to wet ball-mill high-purity atomized aluminium powder into highly polished flakes of aluminium in mineral spirits. A milling lubricant is utilized in the milling process to prevent aggregation of the aluminium particles due to the high degree of energy transfer from the milling process. Aluminium flakes are then passed through a screen apparatus to control particle size and particle size distribution prior to being concentrated in a filter press. The resulting filter cake material is then homogenized in a batch mixer where final quality control inspection is carried out and the non-volatile content is adjusted to that of the desired product specification (Figure 1).
The advances made in this basic process allow conventional cornflake aluminium pigments to be manufactured as well as so-called silver dollar grades (Figures 2 and 3). Cornflake types tend to have a wider particle size distribution and thinner, more irregularly shaped flakes. The silver dollar grades typically have a more uniform round shape, narrower particle size distribution and higher degree of flake surface reflectivity. Further developments of the metallic pigment manufacturing process have resulted in new-technology aluminium pigments such as vacuum metallized pigments and ultra grades. These new technologies, when efficiently formulated, provide an appearance approaching the so-called liquid metal and chrome-like effects that are desired by today's designers and stylists.
The resulting aluminium pigment from this type of milling process is a highly polished flake of high-purity aluminium. This product is typically supplied in the form of an aluminium paste containing 60-80% pigment in a mixture of hydrocarbon solvents and provides a product suitable and compatible with many solventborne coating formulations and applications.
The emergence of alternative non-traditional coating systems has become necessary in Europe to comply with current and pending legislation intended to cut down on VOC emissions. For example, the Solvent Emission Directive has a compliance date of October 2007 and is further supplemented by the Paint Directive, which has a compliance date of 2010 and affects a wider range of ready-to-use coatings. In an effort to comply with these and other directives, a variety of compliant coating technologies have emerged, including (but not limited to) high-solids solventborne, waterborne, powder and radiation-curable coatings.
Each of these brings further challenges to the manufacturers of metallic and other effect pigments. In no particular order, these include:
• The visual appearance of the metallic coating must be acceptable.
• These compliant coatings need to be cost effective compared to the traditional coatings they may be replacing.
• The compliant technologies need to continually evolve to satisfy designer's desire for continued new effects.
• The physical performance characteristics of the coating cannot be compromised in any way.
• Any compliant technologies emerging will also need to satisfy the demands of future legislation such as REACH.

High-Solids Solventborne Coatings
Metallic pigment manufacturers have responded to the move to higher-solids-content solventborne coatings by providing metallic pigments in a product form that is inherently extremely low in VOC content. These are typically supplied in the form of a granule with high metallic pigment content of 80% (Figure 4). A synthetic carrier resin is used to bind the metallic pigment together in a safe, convenient and easy-to-disperse product form. The synthetic resins used are designed to allow exceptional compatibility with most solventborne coatings systems, while typical VOC levels of less than 1% help contribute to maintaining low overall VOC targets in the final coatings. These types of products are finding use in automotive OEM and refinish coatings, as well as in general industrial coatings applications.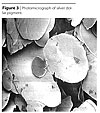
Aluminium in Waterborne Coatings
The emergence of waterborne coatings systems has posed a particular challenge to manufacturers of aluminium pigments due to the high surface area of those pigments and the tendency for aluminium pigment to react with the water in the formulation. This reaction of aluminium with water has the unfortunate side effect of producing hydrogen gas, which as well as being a flammable gas, also leads to pressurization should an unstable paint formulation be stored in sealed, non-vented paint containers. The resulting bulging drums can pose quite a safety hazard. In addition, if the storage stability of the paint is poor, there is the additional problem of stability of the metallic effect. This can result in a significant colour difference in the paint when it reaches the customer, resulting in quality complaints.The solution to this problem is to apply a surface treatment or modification to the metallic pigment to provide protection of the pigment and reduce tendency for reaction with water or the other components of the waterborne coating system. Unfortunately there has been no one surface protection that has been found to be compatible with the wide range of waterborne coatings systems in use or being developed. As a result, a number of surface treatments are employed including: modified phosphonic acid, phospho silicate, phosphono molybdates and silica encapsulation.
Each of these is commonly supplied in a low- or zero-VOC paste form or, more recently, in a granule version with a water-compatible resin allowing easy handling, processing and dispersion.
Irrespective of the surface treatment applied, it is extremely important that the stability of waterborne coatings is confirmed prior to and after scale up to full production for both safety and quality reasons. A typical stability test involves making a 200 g sample of the paint including any required additives used in the formulation and any necessary pH adjustments. This will typically contain approximately 8 g of treated aluminium pigment, although the level could be higher or lower depending on the type of pigment used and the desired effect and properties. This paint sample is then stored at an elevated temperature such as 40 or 50°C for a period of typically 168 hours. This type of accelerated storage test will generate gassing at a rate dependant on the stability of the coating, and this is collected in a calibrated container. A typical specification for such a gassing test would be for a maximum amount of 10mL of gas to be collected at atmospheric pressure, however this can vary depending on the performance requirements of the coating and intended storage conditions, customer location, etc.
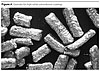
Metallic Effects in Powder Coatings
Traditional metallic pigments in paste form are not of use in powder coatings applications due to incompatibility of the hydrocarbon solvents contained in the paste products and the inability to disperse a paste into the free-flowing powder coating resin. In practice, there are three methods in use to add metallic pigments to a powder coating to produce a metallic-effect powder coating.
1. Co-extrusion process - The metallic pigment is provided in a dry, free-flowing granule form containing a compatible resin. The granule product is added at the pre-mix stage of powder coating production along with the other raw materials and additives. This pre-mix is then passed through the extrusion, kibbling and milling stages of production to yield a metallic-effect powder coating with excellent over spray recyclability and suitability for tribo as well as corona application. However, pigment damage during extrusion is difficult to completely eliminate, therefore this approach is generally used for sparkle or hammer-finish metallic powder coatings.
2. Dry blending process - The metallic pigment is generally supplied in the form of a free-flowing powder where the metallic particles are completely encapsulated by a uniform and coherent silica matrix. The dry blending process involves gentle tumble blending of the finished powder coating resin with the surface-treated metallic pigment in a double cone blender. This type of process provides a metallic-effect powder coating with excellent chemical and humidity resistance often suitable for one-coat application. The type of metallic pigment available allows the formulation of metallic effects approaching some more traditional wet coating effects.
3. Bonding process - The metallic pigment is physically attached (or bonded) to the finished powder coating particles through a combination of mechanical action and heat. This approach provides a metallic-effect powder coating with superior recovery of over spray, however, the mechanical action does result in some pigment damage that can reduce the intensity of the metallic effect as well as reducing chemical stability performance.
A number of surface-treated metallic pigments are available for powder coatings, particularly for addition via either the dry blending or bonding processes. Surface treatment by silica matrix provides excellent compatibility with most powder coating resin chemistries, while a polymer encapsulation is also available providing a metallic pigment with good compatibility, particularly with acrylic powder coatings. Each of these surface treatments improves the spray efficiency of the metallic-effect powder coating whether by electrostatic or tribo application methods. The surface treatments have also been shown to improve bonding efficiency when added via the bonding process. The other major advantage of the surface treatment is the excellent chemical and humidity stability of the final powder coating due to improved pigment stability, particularly when added by dry blending.
Metallic Effects in Radiation-Cure Coatings
Metallic pigments without surface treatment are in use in two-pack radiation-cure coatings where the pigment is isolated to prevent reaction with the monomer used in the coating. More recently, surface-treated metallic pigments have been developed that allow excellent one-pack stability. The surface treatment is generally a modification of silica encapsulation of the metallic pigment, and the resulting product is usually supplied in the form of a low- or zero-VOC pigment or paste exhibiting excellent compatibility with radiation-cure coatings. The high light reflectivity of metallic pigments results in almost no adverse effects on the curing or speed of curing of the formulated coating.Conclusion
Metallic pigments have continued to develop at a pace that allows their use in an ever-wider range of coating applications and meeting designer's desire for added value, product differentiation and consumer appeal. The emergence of compliant coatings to meet ever more demanding environmental considerations while maintaining excellent performance requirements has challenged metallic pigment manufacturers to provide pigments tailored for use in those compliant coatings. Metallic pigment manufacturers have risen to that challenge through advanced pigment technologies and surface treatments that are meeting those demands. Metallic pigments and metallic effects will continue to evolve to meet present and future market requirements.
For further information, visit www.silberline.com.
This paper was presented at the Colour & Appearance of Paint International Conference, May 2006, Copenhagen. The conference was organized by the Paint Research Association Coatings Technology Centre in association with The Departement of Colour Chemistry, University of Leeds.
Looking for a reprint of this article?
From high-res PDFs to custom plaques, order your copy today!