Polymer Weathering - Between Empiricism and Science

Although the weathering of organic materials has long been acknowledged, it was only in the 1950s, with the widespread use of polymeric materials in our everyday life, that the detrimental effect of light became a serious concern to engineers in the plastic industry. It appeared that two main approaches could be considered to address the problem: consider weathering as a global process affecting the functional properties of a material; or approach weathering as a range of chemical reactions at the molecular level accounting for the loss of the service life properties.
One Field, Two Approaches
The first approach is sometimes referred to as conventional or empirical, as it is driven by two empirical principles.1,2
Therefore, with this approach, the polymeric material is considered as a macroscopic system presenting functional properties, which are affected by weathering.3 Back in the '50s, it made sense to set up laboratory tests and interpret the results on the basis of that approach - it was more cost effective than a deeper investigation at the molecular level, quicker, and seemed to be about as reliable. Moreover, acceleration in laboratory conditions could be gained over outdoor weathering by continuously running the instrument.
More than 50 years later, considerable enhancement in the control of weathering parameters in laboratory instruments, together with more accurate evaluation techniques, has increased the reliability of accelerated tests in predicting lifetimes. Despite those improvements, the predictive usefulness of the empirical approach is limited by examples of catastrophic failures, e.g., the delaminating of paint systems in the automotive field, that weren't predicted from accelerated laboratory tests, pushing most paint producers to rely exclusively on dramatically long outdoor exposure tests to evaluate new coating formulations.4 Such deficiencies justify the need to complement this approach with a deeper, more scientific understanding of the weathering phenomenon.
In the mid 1970s, when analytical techniques adapted for the study of the solid state at the molecular level became cheaper, faster and easier to use, a more precise understanding of the chemical processes involved in the weathering of polymers became the main focus of few research laboratories. It has been recognized that most polymeric materials, which undergo environmental stresses under normal conditions of use, should in fact be seen as photochemical reactors. Moreover, the complex chemical phenomena accounting for physical deterioration, also called chemical photo-aging, should be identified at the molecular level.5 In this so-called "mechanistic" approach, the degradation mechanism of a polymeric material is examined not only in its final formulation but also after every processing operation.
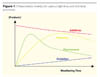
Chemical Photo-Aging at the Molecular Level
The long-term study of the outdoor weathering of several classes of polymers for over 30 years has led to the recognition of a group of photochemical processes involved in the chemical aging of any organic polymeric material. These routes are as follows:
The relative importance of each specific process should be established by analytic techniques, especially by means of in-situ spectrophotometric techniques carried out in the solid state (e.g., FT-IR and UV-visible spectrophotometry).
Each chemical route has its own macroscopic consequence. Photochemical oxidation of the matrix (and in some cases, hydrolysis of oxidation products, e.g., in polyamide or polyurethane) controls the mechanical detriment (through chain scissions), and partly controls the gloss loss via surface micro-cracking.6 Photolysis, yellowing, bleaching, formation and photooxidation of fluorescent compounds contribute to optical change.7 Transformation of additives results in the alteration of their function, especially in fading if additives are colored species or in discoloration when additives are precursors of yellowing substances.8
Several processes may therefore be implicated in the aspect change. Moreover, those processes each have their own kinetics and acceleration factor, as represented in Figure 1. Consequently, when more than one photochemical process is involved in the overall optical change, the choice of this criterion to predict lifetimes from accelerated tests may lead to erroneous conclusions.

Control of Relevance Based on Chemical Analysis
One statement of the mechanistic approach stipulates that the chemical aging observed during any accelerated photo-aging or weathering test should involve the same "mechanism" as in natural weathering to make an accelerated test relevant. Yet, what is to be understood by mechanism?The mechanistic approach is based on a useful description of a photooxidation reaction pathway rather than the analysis of reactive species such as excited states, radicals or vibrationally excited products, which consists of the following steps:9
- - It should be formed in the main degradation pathway as a consequence of a chain scission process.
- It should be a final and stable product that accumulates in the matrix; therefore, it cannot be a product leading to yellowing, and should not be a low-molecular-weight product.
- It should accumulate in a linear fashion with exposure time until the complete loss of the physical properties; since such loss occurs after a very low extent of chemical evolution, the critical photoproduct is formed during the very early stages of photooxidation.
- It should be selected according to the best understanding of the degradation pathway.
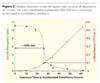
Toward Durability Prediction
When the photooxidation mechanism has been fully recognized in real and accelerated conditions, the controlling chemical detriment is evaluated from the accumulation of the critical photoproduct. In accelerated conditions, the material lifetime is based on the establishment of the relation between the detriment of a service life property and the accumulation of the critical photoproduct. Figure 2 shows the evolution of both the tensile elongation (mechanical test) and the concentration in critical photoproduct for a non-stabilized polypropylene film (evaluated from the variation of the absorbance at 1711cm-1 via FT-IR spectrophotometry in transmission mode) plotted against exposure time in accelerated photo-aging conditions. For instance, we can read that for a variation of absorbance of 0.1, a loss of 50% in tensile elongation occurs.Prediction of the lifetime in natural conditions is then derived via the so-called acceleration factor that is defined as the ratio between the accumulation rate of the critical photoproduct in accelerated weathering and in real weathering.10 For obvious reasons, the accelerated test should be performed in a time span much shorter than the actual material lifetime in real conditions (e.g., during one summer for mildly stabilized formulations).
Prediction of material lifetime based on the variation of functional properties appears more complex. When a product lifetime is defined on the basis of a mechanical property, which depends mostly on the extent of oxidation, prediction is difficult, though not impossible.
When the prediction is based on the loss of gloss, then oxidation is the dominating mechanism and lifetime prediction is possible.11 However, if the change of visual properties is a consequence of several simultaneous processes, such as oxidation, photolysis, formation and bleaching of yellowing products, formation and photo-oxidation of fluorescent compounds, decomposition of pigments or dyes, then lifetime prediction is only possible if one of these phenomena appears to be dominant.
Conclusion
The photochemical degradation of macromolecules in general is fairly complex. Variations of physical properties and of chemical composition can easily be observed during real-life conditions. However, since long periods of time are involved, the variations in environmental parameters (temperature, humidity, light intensity) are not accurately known, although they may be monitored throughout the experiment.
At the laboratory level, each accelerated weathering instrument has specific characteristics depending on the light source used and the type of temperature and humidity control. The relevance of accelerated laboratory experiments to service life conditions cannot be easily and reliably assessed based on the variations of functional properties like mechanical or optical changes since a particular variation can have several different origins. Reliability is gained when relevance is assessed by a more detailed survey of the various processes occurring at the molecular level i.e., oxidation, photolysis, hydrolysis of oxidation products, formation and bleaching of absorbing yellowing substances, formation and photooxidation of fluorescent compounds. Still largely unused in the field of polymeric materials weathering, this approach should gain more and more credit in the coming years.
Looking for a reprint of this article?
From high-res PDFs to custom plaques, order your copy today!