A Simple Polymer Identification Scheme Based on Pyrolysis-GC/MS
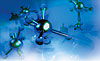
Although Pyrolysis-GC has been used to identify polymers for decades, there has not been a simple scheme available for interpretation of the data. Identification frequently relies on expertise, pattern recognition and the use of special libraries for mass spectra. The problem is compounded by the fact that some compounds generated by pyrolyzing a polymer are unlikely to be found in other analyses, and so are not included in general mass spec libraries.
This paper suggests a simple approach to compiling analytical data in a way that permits identification of typical polymers based on the most prominent and most diagnostic pyrolysate compounds. For many polymers, the production of monomer is significant. The largest peak in a pyrogram of polystyrene, for example, is just styrene. Others, however, produce little or no monomer. Poly(vinylidine chloride), for example, produces trichlorobenzene, which is informative if not intuitive.
Selection of the most important, diagnostic or even prominent peak involves recognition that the relative amounts of compounds made may depend on analytical parameters such as temperature, so the most diagnostic compound may (or may not) be the most abundant. The scheme presented here includes naming several of the most abundant peaks, plus the most chemically significant (and ideally, unique) for identification, as well as other peaks that should be present to support the identification. The data are placed into a spreadsheet, which may then be organized by polymer name, significant product, and most abundant or supporting products.

Introduction
Analytical pyrolysis permits the use of GC and GC/MS to study polymers by breaking the large molecules into smaller pieces using thermal energy. Synthetic polymers frequently degrade to produce monomers. Sometimes only the monomers are made, and sometimes higher oligomers as well, and the monomer may be only a minor product. Other polymers produce small molecules, but no monomers at all.

Copolymers behave in much the same way, frequently producing a peak for each of the monomers in the copolymer, and also frequently making new compounds not seen in pyrograms of the individual homopolymers alone.
Experimental
Synthetic polymers generally produce the same pyrolysis products over a wide range of temperatures, but the relative amounts of these products may shift depending on the pyrolysis temperature. Polystyrene, for example, makes styrene monomer, dimer and trimer at almost any pyrolysis temperature, but relatively more trimer at cooler temperatures. Selection of a temperature for the production of a database must recognize that at significantly different temperatures the analytical results will not be identical, but should still provide typical and useful information about the sample.For this work, the samples were pyrolyzed at 750 °C for 15 seconds in a quartz tube.
Equipment/Conditions
GC/MS: Perkin Elmer Clarus 500
Column: 5% phenyl methyl silicone/ 30 m x 0.25 mm
Carrier: He, 50:1 split
Oven: 40 °C for 2 minutes; 10 °C/ minute to 300 °C
Pyrolysis Unit: CDS Analytical Pyroprobe 5200
Conditions: 750 °C for 15 seconds
Interface: 325 °C
Valve oven: 325 °C
Transfer line: 325 °C

Discussion
The decision was made to try to differentiate polymers based on their significant pyrolysis products. A significant peak in the pyrogram of a polymer could be the largest or most noticeable, a peak that only that polymer produces (large or not) or a smaller peak, which is not specific but always associated with a particular polymer.Most Prominent Peak
Although many polymers produce complex pyrograms with many peaks, all about the same size, there is frequently one peak that is consistently larger than the others. In simple pyrograms, this may be the monomer, but it is frequently not. The largest peak may be a common compound, which by itself does not indicate the identity of the polymer (such as benzene or phenol), but still provides information for interpreting the analytical results. Since experimental conditions, including pyrolysis temperature, detector, scanning range of the mass spectrometer, column performance and compound polarity also affect the relative sizes of analyte peaks, the “largest peak” designation should be taken with a grain of salt, and using other conditions could mean that that peak is no longer the single largest one. It should, however, remain one of the largest and certainly still be present. In some polymers, including the methacrylates, polystyrene, Nylon 6, and many polyolefins (Figure 4), the largest peak stays the same over a wide range of analytical conditions. The bisphenol-A peak in the epoxy shown in Figure 5 is significant, but not diagnostic since polycarbonates also produce bisphenol-A.

The diagnostic peak is the peak, which, regardless of abundance, is a direct indicator of the specific polymer analyzed. Frequently this is the monomer of the polymer, since the monomer of one polymer is rarely produced by pyrolyzing some other polymer. The diagnostic peak could also be the dimer or the trimer, especially if the monomer is a small molecule, present in small amounts, or could be mistaken for some other molecule. For some polymers, the diagnostic peak is also the most prominent, especially for polymers that unzip. Poly(methyl methacrylate), for example, unzips to methyl methacrylate monomer, so the pyrogram shows one large peak, which is also diagnostic. As shown in Figure 1, poly(butyl methacrylate) behaves in the same way.
Some polymers produce a collection of characteristic peaks, but no one peak that is actually diagnostic. Polycarbonates, for example, produce phenolics and bisphenol-A, which together help identify the polymer, but alone are not specific identifiers.

The presence of more than one diagnostic peak indicates copolymers.


Some polymers produce a prominent peak and also some smaller peaks that are reliable and informative, and can help confirm identification. As with the most prominent peak, depending on parameters, these peaks may not always be the second and third largest, but they should always be present. For example, cellulosics and many saccharides produce several furans such as dimethylfuran, furancarboxaldehyde and hydroxymethylfurancarboxaldehyde, which may be fairly small peaks, but if absent make the identification doubtful. Figure 8 shows two of these furans in a pyrogram of Rayon.

The online version of this article features a table that summarizes pyrolysate information for 100 polymeric materials.
Looking for a reprint of this article?
From high-res PDFs to custom plaques, order your copy today!