Analysis of Coatings Using Pyrolysis-GC/MS

Because they are complex formulations of both organic and inorganic materials, coatings offer a special challenge to the analytical chemist. The high concentration of opaque materials that give paints their ability to cover a surface may also make the application of some typical analytical techniques impossible. Further, the organic constituents are generally very high molecular weight, and so not volatile, making both GC and mass spec inapplicable. When dry, the paint is also insoluble, ruling out many other solvent-based analytical procedures.
Analytical pyrolysis is a technique that creates volatile compounds from organic macromolecules, making samples like polymers, adhesives and dried paint suitable again for analysis using gas chromatography, mass spectrometry and FT-IR. Application is simple; a small piece of the solid material is heated rapidly to a high temperature (generally 600 – 800 °C), causing it to degrade into smaller organic compounds, which then are delivered to the analytical device for processing as with any other sample. Interpretation of the resulting fragments requires an understanding of how various polymers behave at high temperatures, but once the basic theory of pyrolytic degradation is understood, the information is invaluable in understanding the molecular composition of these complex systems.
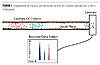
Introduction
Processing polymerized materials by pyrolysis for the purpose of analytical scrutiny has been commonplace for decades.1 Based essentially on free radical-induced degradation, the chemistry follows basic rules of chemistry, and the resulting products are both instructive of the original polymer and reproducible. It is important to use parameters that produce a wide array of products, if possible, which retain much of the identity of the original polymer,2 rather than creating very small, but undiagnostic fragments. Many polymers used in the coating industry produce monomers and other small oligomers upon pyrolysis, so the identity of the original polymer is evident. For example, polystyrene produces considerable styrene, poly(methyl methacrylate) makes methyl methacrylate, and so on. When using gas chromatography as the analytical tool,3 these volatile compounds should be produced quickly and transferred rapidly to the column inlet. Once the pyrolysis volatiles are on the GC column, they behave in the same way as compounds introduced by any other technique (Figure 1). The column contains a liquid phase that interacts with the volatiles as they proceed through the length of the column, separating the mixture of compounds into peaks for individual components. These compounds then pass into the detector, producing a signal presented as a chromatogram. In practice, the pyrolysis step is conducted at relatively high temperatures so that the polymer degrades in seconds. The transfer interface from the pyrolyzer to the gas chromatograph must have a small volume so that the peaks do not spread out, producing poor chromatography.Molecularly, polymers are generally comprised of a long chain backbone (or crosslinked network), onto which are attached groups that make one polymer different from another. Degradation by pyrolysis is initiated by breaking the weakest bond in the polymer molecule. If that bond is part of the chain or backbone, the length of the chain is reduced until the pieces are volatile and can be analyzed by GC. These fragments frequently include the monomer of the polymer, and some polymers unzip to produce little but monomer. Poly(methyl methacrylate), as well as the other methacrylates, and Teflon are examples of polymers that unzip in this way.
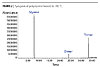
Some polymers degrade by chain bond breaking without producing much of the monomers, but do make characteristic and reproducible products. Condensation polymers like polyamides and polyesters produce a wide array of compounds, which permits distinguishing one polymer from another, even if the monomers themselves are minor products.
Finally, in some polymers, the bonds attaching groups to the side of the chain are the weakest, and these are the first to break. In this case, the side-groups are removed before the length of the polymer is degraded, so monomers are not seen. Still, the products formed are characteristic and indicative of the polymer. Removal of the side-groups frequently leaves behind a polyunsaturated chain, which then breaks apart to form aromatics including benzene, toluene, xylenes and so on.
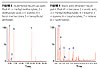
Examples
All of the examples shown here were produced by pyrolyzing a small piece (approximately 100 µg) of dried paint using a CDS Analytical Pyroprobe Model 5200. Sample temperature was 750 °C for 15 seconds, and the pyrolyzer was interfaced to a Perkin Elmer Clarus GC/MS equipped with a 30 m, 5% phenyl methylsilicone capillary column. The GC oven was programmed from 40 °C to 300 °C at 8 °C/minute.Acrylic
Many paints are formulated with acrylic and methacrylic monomers, including automotive paints,4 spray paint and artist media. These formulations often include styrene as well, and are generally random polymer structures. They may be crosslinked and include additives such as plasticizers.
Since the acrylics and polystyrene either unzip or form oligomers, the composition of the paint may be ascertained even from the dried, cured, crosslinked final product. In addition, the presence of inorganic fillers does not interfere with the process. Figure 3 shows the pyrolysis results for a sample of dried automotive touch-up spray paint. The formulation includes methyl methacrylate, methacrylic acid, butyl methacrylate and styrene. There is a pronounced peak for the plasticizer benzylbutyl phthalate as well. This plasticizer is not chemically bound to the paint polymer and could be thermally removed at a lower temperature to separate it from the pyrolysis products. A different paint formulation using the same monomers but in different amounts would produce a pyrogram with the same peaks,5 but different relative sizes for those peaks, making distinction among even very similar formulations possible.
House Paint
Alkyd house paints that are glyceryl phthalate polyester-based pyrolyze to produce mostly phthalic anhydride and some small, oxygenated compounds from the glycerol. By contrast, the water-based latex emulsions produce much more complex programs. Typically, these paints contain considerable amounts of polyvinyl acetate. The bonds attaching the acetate groups to the polymer chain are relatively weak, and break first when the sample is pyrolyzed. This releases acetic acid and leaves behind an unsaturated polymer chain. As the sample is heated further, this chain breaks apart to form a wide range of aromatic compounds, including benzene, indene and naphthalene. At the same time, the acrylic part of the paint is also being pyrolyzed, so the pyrogram will also contain compounds from that polymer as well. The products from the various polymeric constituents are produced independently and do not interfere with each other.
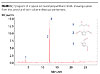
Polyurethanes
Polyurethane polymers are made by reacting a di- isocyanate with a di- or poly-functional polyol. When they are pyrolyzed, the diisocyanate is regenerated and becomes one of the dominant peaks in the pyrogram. Other peaks provide information about the nature of the polyol used in making the polymer. The polyols may be polyester based or polyether based, and information is generated that helps distinguish the two. Polyester polyols are frequently made using adipic acid and, upon pyrolysis, this portion of the molecule produces cyclopentanone. Polyether polyols will not make cyclopentanone, but will produce small compounds that retain the ether structure.
A piece of dried polyurethane finish was pyrolyzed to produce Figure 5. This was a typical solvent-based finish, made with toluene diisocyanate. Toluene diisocyanate is usually a mixture of two isomers, the 2,4- and 2,6- configurations. Not only is the diisocyanate regenerated – a marker for polyurethanes – but in the case of isomers, the isomeric configuration is preserved in the pyrogram. This is true of complex formulations in which the polyurethane content is relatively small in addition to materials that are essentially pure polyurethane. As a consequence, the relative amount of polyurethane in a paint or finish may be assayed using pyrolysis.
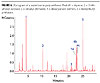
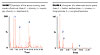
Epoxies are considered rugged and indestructible, but they are organic polymers and may be analyzed using Py-GC/MS like any other polymer.6 Most epoxies are made using Bisphenol A, which is one of the main products when the sample is pyrolyzed. Figure 7 shows results for a sample of the coating used as a liner inside a food can. A small piece was scraped off the inner surface of the can and pyrolyzed, revealing phenol and Bisphenol A among other things.
Although the presence of Bisphenol A is important in identifying the epoxy, it is actually not diagnostic for epoxies since polycarbonates also use Bisphenol A. In these cases, the presence and relative abundance of the other pyrolysis products are important to make the distinction.
Additives
Not all of the peaks in a pyrogram are necessarily pyrolysis products from the polymer. If the sample has been heated just once, volatile and semi-volatile compounds may also be present, which are desorbed from the polymer before it pyrolyzes. Residual solvents, contaminants and additives7 are likely to be present among the peaks from the polymer itself, and may be important in the analysis of the paint as a finished product. These compounds may be present in small quantities, making only small peaks, or they may be substantial as in the case of plasticizers. When the additives are present at low levels, it is helpful to adopt a multi-step approach8 to the analysis. In this case, the sample is heated to one or more temperatures below the pyrolysis temperature of the polymer, releasing the volatiles intact but not degrading the polymer. This removes the trace-level compounds, like antioxidants and light stabilizers, and puts them into their own chromatogram where they can be studied without all the peaks from the polymer pyrolysis.
Plasticizers are usually present at sufficient concentration to be identified easily from a single sample run. The paints in Figures 3 and 6 both contain a phthalate plasticizer, which produces a substantial peak and is easily identified, and would not be confused with the pyrolysis products made from the paint polymer. There is an interesting additive in Figure 8 as well. This is a satin acrylic paint that contains methyl methacrylate, styrene and butyl acrylate, which are the three largest peaks in the pyrogram. There are smaller peaks, also related to the polymer structure, and then the peak marked #4, which is octylisothiazolone (2-n-octyl-4-isothiazolin -3-one). This is a mildewcide that has a mass spectrum different from the monomers used in the paint, and is identified by the library of spectra used by the mass spectrometer. Had the paint been heated to 200 °C for a desorption run before the pyrolysis, this peak would show up in the first run, since it is desorbed intact and is volatile enough to go through the gas chromatograph.
Conclusions
Coatings may be analyzed, even as finished products that have been dried and cured, using gas chromatography and mass spectrometry when coupled to a pyrolyzer. The sample is converted to volatiles – either desorbed intact molecules or fragments of the polymer – by the application of heat. Since no solvents are used, any solvent molecules detected are known to come from the sample. The type of polymer involved, the monomers used, the relative concentrations, and even the polymer microstructure may be studied by identifying the compounds produced when the polymer is pyrolyzed. No special adaptations of the GC/MS are required other than an interface linking the pyrolyzer to the injection port of the GC.For more information see http://cdsanalytical.com.
Looking for a reprint of this article?
From high-res PDFs to custom plaques, order your copy today!