Self-Crosslinkable Thermosetting Acrylics for Use in Water-Dilutable Coatings
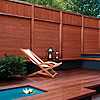
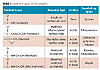
These properties can be controlled by the use of acrylics in the coating formulation. They can provide a range of properties to the applied films due to the wide range of available monomers.2 The incorporation of two different acrylic-type monomers, having diverse physical and/or chemical properties, in the same polymer molecule in varying proportions leads to the formation of new acrylic copolymers with great scientific and commercial importance.3 To obtain optimal properties, high-performance coatings require crosslinking, as the crosslinking improves film toughness, provides solvent resistance and reduces dirt pick-up.4 That’s why crosslinkable or thermosetting acrylics (TSAs) are increasingly used in a variety of surface coating applications, since they are designed to react chemically after application.5
Generally, TSAs are stoving-type systems, in which the final film is obtained after heating at a particular temperature. When TSA resins are stoved, the reactive groups from the functional acrylic monomer units take part in crosslinking reactions, which convert the low-molecular-weight polymer to a high-molecular-weight film-forming material. On the basis of their crosslinking mechanism, TSAs can be sub-classified into two main groups.
(1) One–pack TSA systems: In these systems, the use of external crosslinkers is not needed because the two types of pendant side groups present in a molecule crosslink with themselves intra-molecularly in the presence of trace amounts of catalyst, above a threshold temperature (e.g., TSA resin containing glycidyl and methylol groups).
(2) Two-pack TSA systems: For these systems the use of an external crosslinker is needed because the polymers by themselves are not capable of undergoing chemical reactions through the pendant chemical groups. Therefore, the addition of an external agent – a crosslinker – is required. The crosslinker must be at least difunctional.6 Table 1 shows five TSA systems that are commercially exploited today.7
Industrially, TSAs are formulated in several ways and can be grouped conveniently into two classes: solventborne TSAs (where organic solvent is used for binder dissolution) and waterborne TSAs (where water is used for binder dissolution). Solventborne TSAs have a particular prominence in the area of industrial coatings, where performance is essential.4 Waterborne TSA systems for surface coating applications are growing rapidly and expanding vigorously, which may be attributed to the fact that regulations on all the four E’s, i.e., emission, environment, ecology and energy, could be restored.8
In the present work, a series of TSA resins were prepared by using 2-hydoxyethyl methacrylate (HEMA) and acrylic acid (AA) as monomers in varying molar ratios and butanol as solvent in the presence of benzoyl peroxide (initiator) at 60 °C. The prepared TSA resins were made water-soluble by neutralizing with ammonia solution. The coating films were then baked at 90 ºC for 30 minutes and the various optical, mechanical and chemical resistance properties of the cured films evaluated. In this work, the thermal curing and thermal degradation behavior of water-thinnable TSA resins were also studied by differential scanning calorimetry (DSC) and thermal gravimetric analysis (TGA) respectively.
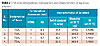
Experimental
Materials• Acrylic acid: reagent purity-grade (E-Merck) was distilled under reduced pressure.
• 2-Hydroxyethyl methacrylate: reagent purity grade (E-Merck) was distilled under reduced pressure.
• Benzoyl peroxide: reagent purity-grade (E-Merck) was used as received.
• Butanol: reagent purity-grade (E-Merck) was distilled under reduced pressure.
• Ammonia solution (15% concentration in water): reagent purity-grade (S.D.fine) was used as received.
Synthesis
Copolymer synthesis was carried out by using various monomer mole ratios of (a) 1: 0.3 (b) 1:0.5 (c) 1:0.7 and (d) 1:1 as 2-hydroxyethyl methacrylate to acrylic acid respectively. The monomer-to-solvent ratio was kept as 40:60 (w/w). The benzoyl peroxide initiator concentration was 6.6% (w/w) to total monomer weight. The reaction setup, consisting of a three-necked round-bottom flask (capacity 500 ml) equipped with mechanical stirrer, thermometer pocket and water-cooled condenser, was used as the reactor for resin preparation. The flask was placed in a thermostatically controlled water bath held at 60 °C. After 1.5 hours, when the polymerization was completed, the solution was cooled to terminate the polymerization reaction, and neutralized with aqueous ammonia (15%, w/w) to a pH of 8.5 – 9.0 for making it water-soluble. The prepared water-soluble resinous material was separated by a separating funnel. Following this procedure and using different molar ratios of HEMA to AA, several other water-soluble TSA resins could also be prepared. The prepared resin designations, along with the details of their formulation, are given in Table 2.
Characterization
Infrared spectra of the synthesized co-polymers (before neutralization) were recorded by using NaCl dies on a Perkin-Elmer 1750 FT-IR spectrophoto-meter. 1H NMR spectra were generated by an FT NMR system (Lambda JEOL JNM-LA400) and chemical shifts reported as (ppm) relative to tetramethyl silane. For DSC analysis, a universal V4.2E TA Instruments Q20 differential scanning calorimeter was used. The temperature range was kept as 20-200 °C, at the rate of 20 °C/min. The analysis was carried out on a 10 mg sample in an argon atmosphere at the rate of 40 ml/min. The thermal stability of TSA resin was measured by using a universal V4.2E TA Instruments Q50 thermogravimetric analyzer under dynamic scans (from 0-600 °C at the rate of 20 °C/min) of 13.4470 mg sample held under the dry flow of nitrogen at the rate of 30 ml/min. The refractive index of aqueous resin solutions was determined by an Abbe refractometer, at 25 °C. Viscosity of the aqueous resin solutions was determined by Brookfield cone and plate 2000 + viscometer, at 25 °C (w.r.t. spindle - 3). Solid content of aqueous solutions was checked as per ASTM D 1259.The results of characterization, along with the TSA resin designations, are presented in Table 2.
Coating Preparation
The prepared water-thinned TSAs were used for the application of clear films on glass plates (5 cm × 10 cm) and tinned mild steel panels (5 cm × 15 cm). Dry film thickness (DFT) of the coated film was maintained at around 30 µm (with the help of a bar applicator). DFT of the coated films was measured by a Positector 600 (Sheen, U.K.). The films were allowed to bake at the required temperature in an air-circulatory oven for effecting film cure. Then various optical, mechanical and chemical resistance properties of the cured films were evaluated as per standard procedures.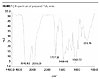
Results/Discussion
Chemical StructureIR-Spectra
FTIR spectra of each TSA resin, obtained before neutralization, were almost the same. Figure 1 shows the IR spectrum of resin TSA4, which confirms the molecular structure of TSA resins in all aspects. The sharp band in the range of 2935 cm-1 may be due to C–H stretching vibration of methyl and methylene groups. Absorption at 1460 cm-1 is assigned to asymmetric –CH3 or –CH2 bending vibrations. A sharp band at 1717 cm-1 is attributed to C=O stretching frequency. A sharp broad band in the range of 3342 cm-1 is due to O–H stretching. A split peak at 1069 cm-1 might be due to C–O stretching of alcoholic and ester groups. The absence of a peak at 3000-3040 cm-1 and at 1600-1700 cm-1 indicates that no vinyl hydrogen is present in the final molecular structure of the polymer.
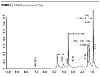
Proton NMR spectra were all as expected, with resonance at 3.5 ppm [t, 4H, O-(CH2-CH2)-OH], the singlet peak at 4.8 ppm (s,H, OH), 1.4 ppm (Figure 2) and 0.6 - 0.8 ppm (Figure 2). The 1H NMR spectrum (Figure 2) of sample TSA4 was a close match to its theoretically elucidated structure.
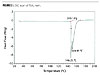
Thermal Behavior
Differential Scanning Calorimetry (DSC)The curing characteristics were determined by DSC. A thermoset acrylic resin DSC thermogran is shown in Figure 3. The figure clearly indicates a sharp peak temperature range from 146 to 150 °C, which could be the curing temperature of the prepared TSA resin. Total heat required for this was 315 J/g.

The thermal decomposition behaviour of organic polymers is commonly determined by TGA. The TG curve (Figure 4) reveals the weight loss of substances in relation to the temperature of thermal degradation. Figure 4 shows excellent thermal stability of the synthesized resin TSA4. TGA of the copolymer shows major weight loss at two stages. The first stage ranges from 20 to 170 °C, which shows about a 65.51% weight loss. This may correspond to the evaporation of water molecules from the compound having a 32.3% solids content. The second stage of weight loss starts at 170 °C and continues up to 380 °C during which there was a 10.51% weight loss due to the evaporation of free monomer and bound water present at that stage. The decomposition temperature of this compound is 465.95 °C.
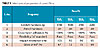
Evaluation of Film Properties
Cured films were tested for their optical, mechanical and chemical resistance properties, as per standard test methods viz. gloss at 60° (ASTM D 523-99); scratch hardness (ASTM D 5178); pencil hardness (ASTM D 3363–00); flexibility (ASTM D 4541); impact test (ASTM D 2744); chemical resistance (ASTM D 1308-87); and solvent resistance (ASTM D 5402).Mechanical Properties
(a) Scratch Hardness
Scratch hardness was determined using an automatic Scratch Hardness Tester (Sheen, UK) having a hardened steel hemispherical point of 1 mm diameter as scratching needle. Results are shown in Table 3.
It is clear from the data that films having higher acrylic acid content had high scratch hardness because acrylic acid is known to impart hardness to the films. Consequently, scratch hardness of the films increased from TSA1 to TSA4.
(b) Pencil Hardness
A cured film strip was drawn under a pencil until a pencil hardness grade was reached to scratch the surface. The coating film was then assigned a hardness value such as H, 2H, 3H etc., signifying the hardness grade using a Pencil Hardness Tester (Sheen, UK). Results of pencil hardness tests are also shown in Table 3. Coating films of TSA3 and TSA4 showed high pencil hardness due to higher acrylic acid content.
(c) Crosshatch Adhesion
Adhesion was measured by using a Crosscut Adhesion Tester (Sheen, UK). The tester consists of a die made up of 9 parallel blades, 1/16 inches apart and 1 mm long. The die was pressed onto the panel in two directions at right angles to each other. A strip of self-adhesive tape was then stuck over the pattern, left in contact for 10 seconds, and then stripped rapidly by pulling the tape back at an angle of ~120º. The property was rated as good if 95% of squares were not removed. The results were recorded as percentage of squares not removed. All the coating films had good crosshatch adhesion (Table 3).
(d) Flexibility
Flexibility was carried out on a ¼-inch Mandrel Bend Tester (Sheen, UK). All coating film compositions passed the ¼-inch Mandrel bend test. Therefore, it can be said that the film had reasonably good flexibility; results are shown in Table 3.
(e) Impact
Impact resistance was determined using a Tubular Impact Tester (Sheen, UK). Values were reported in cm at which the film passed the impact test using a weight of 2 lb (approx. 0.91kg). Impact test results are presented in Table 3.
It is clear from the data that films having higher HEMA content, had high impact resistance, because HEMA is known to impart flexibility to the coating films. That could be the possible reason why impact resistance decreased progressively from TSA1 to TSA4.
(f) Gloss
A Triglossometer (Sheen, UK) was used to measure films at a 60º angle; all coating films had good gloss (Table 3).
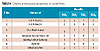
Cured coating films were tested for their chemical resistance properties and data is presented in Table 4.
(a) Acid
Coating films were immersed in 1.0 N sulfuric acid up to a period of 7 days. The films of TSA1 and TSA2 were highly affected (loss in gloss along with slight softening) by the acid solution due to their alkaline nature. Films of TSA3, due to their slight alkaline nature, showed only slight loss in gloss. This behavior can be attributed to the fact that after crosslinking some unreacted hydroxyl groups were present in the coating films. The TSA4 film was unaffected by acid solution due to the presence of a sufficient degree of crosslinking (Table 4).
(b) Alkali
Coating films were immersed in 1.0 N sodium hydroxide for 1 week. All coating films were unaffected by alkali solution due to their alkaline nature (Table 4).
(c) Water
Coating films of TSA1, TSA2 and TSA3 were slightly affected due to moderate polarity because of the presence of free hydroxyl groups when they were immersed in distilled water up to a period of 2 weeks. TSA4 films were unaffected because of the higher degree of crosslinking (Table 4).
(d) Solvent
All films exhibited good solvent resistance when immersed in non-polar solvents like xylene and mineral turpentine oil. But, when exposed to low-polar MEK, a slight loss in gloss was observed in TSA1, TSA2 and TSA3. This film behavior might be due to the fact that even after crosslinking, the presence of some unreacted OH-functional groups leads to moderate polarity in coated films. On immersion into ethanol, almost all films of TSAs showed slight loss in gloss due to the attack of moderatly polar ethanol on moderate polar films. In the coating films of TSA1 loss in gloss along with slight softening was observed due to the presence of a lesser degree of crosslinking, which leads to high polarity in the films (Table 4).
Conclusion
Four TSA resin samples were prepared successfully in the presence of varying amounts of 2-hydroxyethyl methacrylate (HEMA) and acrylic acid (AA). On comparing the performance properties, TSA4 outperforms TSA1, TSA2 and TSA3, except that it has low impact resistance. Among all the coating compositions, films of TSA4 showed good optical, mechanical and chemical resistance properties. This behavior of TSA4 might be due to the presence of a higher degree of crosslinking.Therefore, it can be concluded that water-thinable TSA coatings based on the developed copolymer/resin prepared by using AA and HEMA in the molar ratio of (1:1) can be used as protective coatings for automobiles and other industrial applications, as eco-friendly coatings with no/negligible evolution of VOCs and hazardous air pollutants.
For further information contact pramod.hbti@yahoo.co.in.
Looking for a reprint of this article?
From high-res PDFs to custom plaques, order your copy today!