Nanoparticle Additives for Enhanced Scratch Resistance in UV-Cured Coatings
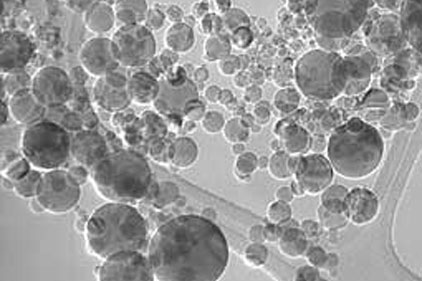
To answer this question, it is instructive to study the evolution of attempts to use nanoparticles in coatings applications in general. In some instances, products were taken to market by nanotechnology companies which, while perhaps expert in the synthesis and manipulation of nanomaterials, had little technical, marketing or sales expertise in the coatings industry. In other cases, large, well-known chemical additives companies with a strong existing presence in coatings but little knowledge of nanomaterials attempted to invent or purchase nanotechnology and rapidly deploy it through existing channels to market. Neither of these approaches has been especially successful commercially; many small nanotechnology companies have simply disappeared, and at least one large specialty chemical company has exited the nanoadditives business altogether.
The authors have identified what they believe to be the primary barriers to the successful technical and commercial use of nanotechnology in the coatings industry, and have assembled a model that has been shown to be successful in guiding the development of commercial coatings products using nanotechnology solutions to solve long-standing, unmet challenges. Although the model is general, the present examples will be selected from the area of scratch protection in transparent UV-cured coating systems, showing the performance improvements possible with nanometric aluminum oxide-based additives and a framework upon which to consider performance, cost and transparency simultaneously.
Discussion
![]() |
Figure 1 Click to enlarge |
A Prescriptive Three-Step Model for Success
Owing to the very high surface areas, the stabilization of nanoparticulate metal oxide dispersions (such as aluminum oxide) can be challenging and often requires both specialized chemistry and process technology. If the nanoparticles are not (or cannot be) dispersed down to their primary particle size, transparency in coatings will not be achieved except in the event that the refractive index of the coating exactly matches that of the particle. Because of this, most users of nanometal oxides, even those experienced with pigment dispersions, will fail to achieve the desired result when attempting to work with nanomaterials in powder form. Incomplete or improper dispersion will result in high viscosities, rapid gravimetric settling and large particle agglomerates.
In addition to having a stable dispersion of primary particles to begin with, it is also necessary that the dispersion be compatible with the coating formulation it is intended to be incorporated into. Because of the wide variety of coating ingredients (resins, additives, pigments, etc.) and the number of variables typically present (resin chemistry, solvency, pH, solids loadings, etc.), the effective use of nanoparticle additives is not a “one size fits all” situation, and companies that attempt to market nanoparticle dispersions with but a limited repertoire of formulation tools are likely to be unsuccessful. In the examples to be considered here, failure to achieve compatibility with the target UV-cured formulation will result in opacity (haze) and poor scratch protection. It is primarily for this reason that nanomaterial additive companies that simply “throw samples over the wall” without the means to ensure compatibility with customer formulations will, in most instances, disappoint their customers.
Finally, given that stable dispersions of primary particles are available and that compatibility with the target coatings formulation can be achieved, it is necessary then (and only then) to empirically determine the impact of the nanoparticle type, size, loading level and surface treatment on the coating properties in order to identify the optimum solution that balances performance, cost and transparency in a system. This optimized solution will likely vary from formulation to formulation, and thus knowledge of both the nanomaterial properties as well as the end-use application is critical if success is to be realized.
Aluminum Oxide Nanoparticles
It is well known that the incorporation of micron-size alumina (grit) in applications such as pre-finished laminate flooring substantially improves the scratch and abrasion resistance. The extreme hardness of the alumina particles translates into effective wear resistance when incorporated into such coatings; however, the use is restricted to substrates where the high haze resulting from the particle loading can be tolerated, or in systems where the refractive index of the resin binder is sufficiently high so as to mask the presence of the alumina (e.g., melamine formaldehyde). In order to allow the use of alumina particles in clear topcoats while retaining a high level of transparency and gloss, it is necessary that the particle size be reduced to the nanometer size range.
The light-scattering behavior of sub-micron-sized particles dispersed into a fluid medium such as a coating matrix is described by Mie theory, and the relationship is summarized by Equation 1 where Is refers to the intensity of scattered light, N = the number of particles, d = the particle size, and ∆η = the difference in refractive index between the fluid medium and the particle.
Is = (N)(d6)(∆η)2 (1)
Since the refractive index of aluminum oxide (1.72) is substantially higher than that of UV-cured coating resins, either the number of particles or the particle size must be minimized in order to minimize the amount of light scattering (which is perceived as haze to the eye). Of the two parameters, the particle size has the stronger influence on the extent of light scattering and resulting haze; the authors have found that in UV-cured coatings, alumina with an average particle size of < 50 nm is required in order to retain a high level of transparency.
One process by which nanoscale aluminum oxide particles can be prepared is through physical vapor synthesis (PVS) using a plasma arc. A transmission electron micrograph (TEM) of aluminum oxide prepared by this process is shown in Figure 1. The crystal phase of alumina made by this process is γ/δ, and the primary particles emerge with a spherical morphology. A unique aspect of the plasma process is that each crystalline alumina sphere forms as a discrete entity without any durable particle-particle superstructure that, if present, could prevent full dispersion to primary particles.
![]() |
Figure 2 Click to enlarge |
The physical vapor process yields a log-normal distribution of the discrete alumina primary particles, as depicted by the particle size distributions shown in Figure 2, which were obtained using a CPS disc centrifuge particle sizer. The figure shows the relative size distributions of alumina particles prepared by two different plasma processes. Variation in the plasma conditions allows control over the average particle size as well as the particle size distribution.
Particle Dispersions and Formulation Compatibility
While the first step toward the production of an alumina product suitable for use in transparent UV-cured coatings is the synthesis of discrete nano-size particles exhibiting no significant secondary structure, the second step is the dispersion of those particles from the powder state, where they are weakly associated by electrostatic forces, into a format suitable for the coating application. Creating stable dispersions of nanomaterials can be a significant challenge owing to the strong tendency for primary particles of this size to associate and flocculate in fluids because of the very high surface area. It has been discovered that stable dispersions of nanoparticles prepared via the plasma vapor process can be achieved by surface treating the primary particles with a stability package designed specifically for the particle/liquid interface. In order to prepare concentrated alumina dispersions for use in 100% solids UV-curable coatings formulations, a surface treatment was designed that allows stable dispersions to be generated directly in low-viscosity acrylate monomers such as tripropyleneglycol diacrylate (TPGDA) and 1,6-hexanediol diacrylate (HDDA). With such stability packages, concentrated but fluid dispersions containing up to 30 wt% alumina can be prepared.
![]() |
Figure 3 Click to enlarge |
The surface treatment package used to disperse and stabilize the alumina particles against flocculation and settling in the concentrated dispersion must also be capable of providing compatibility when this concentrate is let down into the coating formulation. As an example, the vials shown in Figure 3 represent two extremes of compatibility.
Starting with the same UV-cured coating formulation, vial A on the left contains 1 wt% of a nano-alumina product, which was prepared with a surface treatment that allows for a fully compatible and stable system, whereas vial B on the right contains the same concentration of nano-alumina but was prepared with an ineffective surface treatment system that exhibits incompatibility and has begun to flocculate out of the coating formulation. The key to generating compatible systems of nanoparticles and coatings is an intimate understanding of not only the surface chemistry of the nanomaterial itself, but also of the coating application in which the nanomaterial is to be incorporated.
Results
![]() |
Figure 4 Click to enlarge |
Clarity and Scratch Resistance
The performance of alumina nanoparticles in improving scratch resistance while having but a minimal impact on clarity was evaluated in a commercial UV-cured coating formulation. Aluminum oxides with mean particle sizes of 20 and 40 nm prepared via a physical vapor synthesis process were surface treated and dispersed at 30 wt% in TPGDA monomer. These concentrated alumina dispersions were then blended into the commercial coating formulation at loading levels ranging from 0.5 to 2.5 wt% alumina solids on total resin. The resulting formulations were applied to glass substrates at 10 µm film thickness, cured by UV exposure, and the haze measured to determine the effect of the alumina particles on the clarity of the coatings. The results are depicted in the graph of haze versus alumina loading shown in Figure 4.
At loading levels up to 2.5 wt% with the 40 nm alumina particles, the haze remained at less than 1% in the coating. As expected, the 20 nm alumina particles had even less impact on the film clarity with significantly lower haze when compared against the 40 nm system. Although haze values of less than 1% can be measured in these nano-alumina containing films when applied to a transparent substrate, in actual practice haze readings of less than 1% are not perceptible to the eye in coatings applied to opaque substrates. It is this very high clarity level, achievable only with well-dispersed alumina particles in the nano-size range, which allows for their use in transparent UV-cured coating applications.
![]() |
Table 1 Click to enlarge |
To evaluate the effect of nano alumina particles on the physical properties of UV-cured coatings, a commercial coating formulation was loaded with 40 nm alumina particles at 0.5, 1.0, and 2.0 wt% on resin (using a concentrated dispersion of the particles in HDDA monomer). The system was then applied at 4 mil thickness to a glass substrate, and the pencil hardness was measured after curing. In this test, a weighted stylus containing a graphite “pencil lead” is pulled across the surface of the coating and the surface inspected for a scratch.
The progression from softer to harder “lead’ in the stylus before a scratch is observed may be taken as an indication of improved relative hardness of the film. The results for the system under discussion are shown in Table 1. The incorporation of the alumina particles increased the coating hardness from a level of HB in the unfilled (0 wt% alumina) coating up to 3H (4 “lead” grades harder) with 2.0 wt% alumina loading. While the increase in film hardness does not always correlate positively to increased wear resistance, it is a good indication that the alumina nanoparticles are improving the scratch-resistant properties of the coating, even at relatively low concentration, while not degrading the appearance of the coating by reducing gloss or contributing haze.
![]() |
Figure 5 Click to enlarge |
The scratch-resistant properties of a UV-cured coating containing nano alumina were also measured using a steel wool scratch test. In this test, a steel wool pad was fitted with a one pound weight and attached to the arm of a reciprocating linear motion abrader instrument. Coatings were applied to a transparent substrate and subjected to repeated scratch cycles with the weighted steel wool pad. After a set number of cycles, the haze of the film was measured to determine the degree of scratching as evidenced by the increase in haze of the coating.
A scratch test was conducted on a commercial UV-cured coating into which 40 nm alumina was incorporated at levels ranging from 0.5 to 2.5 wt% alumina on resin using a TPGDA concentrate as the vehicle to deliver the alumina nanoparticles. A #0000-grade steel wool pad was used, and the coatings were subjected to 1000 double cycles with the linear motion abrader. In this case, two different surface treatment packages were applied to the alumina particles during the dispersion process.
Both treatment systems provided full compatibility between the particles and the coating formulation in terms of clarity; however, the treatments resulted in different performance with respect to scratch resistance. The results of the scratch tests are shown in Figure 5. The coating containing the alumina particles featuring surface treatment A showed a significant improvement in scratch resistance with an alumina loading of just 0.5 wt%, then a relatively flat response as the alumina loading was increased. With this surface treatment, the scratch resistance of the coating was more than doubled using as little as 1.0 wt% of the nano-alumina additive. The performance of the alumina system using surface treatment B was even more dramatic. The results indicate that the degree of scratching can be reduced by a factor of 5 using alumina loadings in the 1.5 to 2.5 wt% range. This example illustrates the necessity to conduct the final optimization step, after dispersancy and compatibility have been achieved, in order to realize the maximum performance and/or the most economical use of the nanoparticle additive in a particular system.
Conclusions
Successful incorporation of nanometric aluminum oxide particles into UV-cured coating formulations for improved scratch resistance requires adherence to a three-step process: (1) Preparation of a stable, concentrated, liquid dispersion of primary alumina particles directly in an acrylate monomer; (2) Ensuring compatibility of the nanoparticle dispersion concentrate with the coating formulation; and (3) Optimizing the performance of the nanoparticles with respect to clarity (appearance), scratch resistance (performance) and loading (cost).
If the three-step model is followed, aluminum oxide nanoparticles can enable transparent UV-cured coating formulations with substantially improved scratch resistance while expressing minimal negative impact on the coating clarity or gloss. Through selection of the proper alumina particle surface treatment, the scratch resistance of these additives can be optimized for maximum performance.
This paper was presented at the RadTech 2010 Technology Expo and Conference, Baltimore, MD, www.radtech.org.
For more information, visit www.nanophase.com.
Looking for a reprint of this article?
From high-res PDFs to custom plaques, order your copy today!