Pigment Selection for Colored UV-Curable Powder Coatings
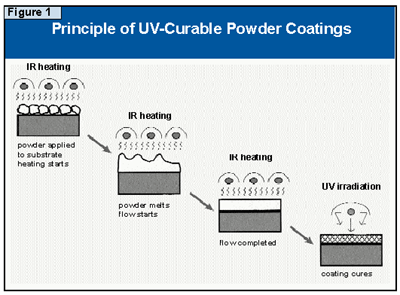
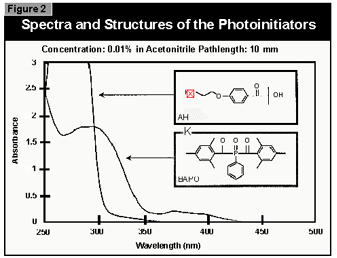
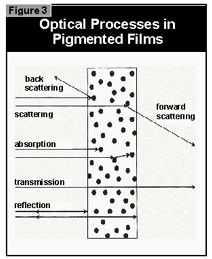
UV-curable powder coatings need to be formulated in a variety of surface finishes and colors, and some initial work on this problem has already been reported
(1). When selecting pigments for conventional, thermally cured powder coatings, the thermal stability of the pigment must be considered. This is not a problem with UV-curable powders. As with conventional powder coatings, however, there is a need to achieve optimal pigment dispersion in one extruder pass and to have minimum batch-to-batch variation in color. For these reasons, as well as for cleaner handling, the use of solid pigment dispersions, such as the Microlen range of products, is most advantageous.
Selection of the appropriate photoinitiators is also a matter of great importance. If the photoinitiator lowers the glass transition temperature of the resin (i.e. has a plasticizing effect), the storage stability and ability to recycle the UV-curable powder will be impaired. Other key properties of a suitable photoinitiator for UV-curable powder coatings are (in addition to the spectral absorption): low volatility, low yellowness after cure and absence of volatile scission products that cause odor
(2). For curing pigmented systems, the combination of an alpha-hydroxyketone (AHK) and a bis-acylphosphine oxide (BAPO) is the most suitable. The alpha-hydroxyketone gives the coating good surface cure because this class of compounds is relatively insensitive to oxygen inhibition. The bis-acylphosphine oxide enables a deep cure in pigmented systems because it absorbs in the visible at wavelengths where TiO2 opacifiers are transparent. Also, the ability of the BAPO class of photoinitiators to photobleach during cure promotes cure through to the base of the coating.
In
a previous study, bis(2,4,6-trimethylbenzoyl)-phenyl-phosphine oxide (Irga-cure
819) and 1-[4-(2-hydroxyethoxy)-phenyl]-2-hydroxy-2-methyl-1-propan-1-one (Irgacure
2959) were found to fit the requirements for photoinitiators for UV-curable
powder and were therefore used in this work (Figure 2).
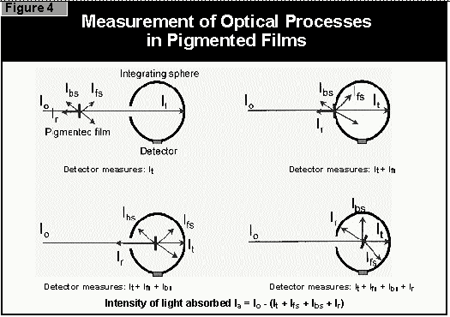
- the light is reflected off the surface of the coating
- the light is scattered by the pigment
- the light is absorbed by the pigment
- the light passes through the coating without being absorbed.
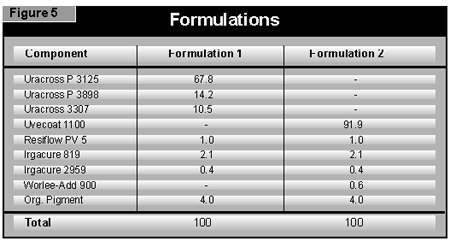

The extrudates were milled to give a powder of mean size at about 45 µm using a Retsch ultracentrifugal mill and sieved through a 125-µm sieve, and 0.25% of silica (Aerosil R972) was added to the crushed extrudate before milling to prevent the finished powders from caking. The powders were sprayed using a Wagner Tribo-star gun. A coating film of exactly 70 µm was achieved by spraying the powder first onto a metal panel with a metal backing plate behind it to prevent powder from adhering to the rear of the panel. In this way, the weight of powder needed to produce a 70-µm film on the metal panel could be determined. The MDF panels could then be sprayed in the same manner until the required weight of powder had been applied.
The coated panels were then melted under an IR lamp until a surface temperature of 140°C was reached and then cured immediately. The panels were cured in an Aetek exposure unit by placing them on a moving belt at different speeds and passing them under 2- by 80-Watt/cm Fusion type H medium-pressure Hg-vapor UV-lamps. The temperature of the panels leaving the conveyor was about 130°C. With the BAPO photoinitiator, it is possible to use normal, undoped mercury lamps.
The physical properties of the steel and MDF panels were tested when they had cooled (30 minutes after curing). The results are as follows:
König pendulum hardness. An average of three determinations according to DIN 53157 was taken.
Acetone
double rubs. A 10- by 10- by 5-mm felt pad was used for 100 double rubs, and the
result assessed visu ally:
1 = no damage, 2 = slight matting or scratches, 3 = moderate scratching, 4 =
heavy damage and 5 = coating fully destroyed. This test main ly
indicates the level of surface cure.
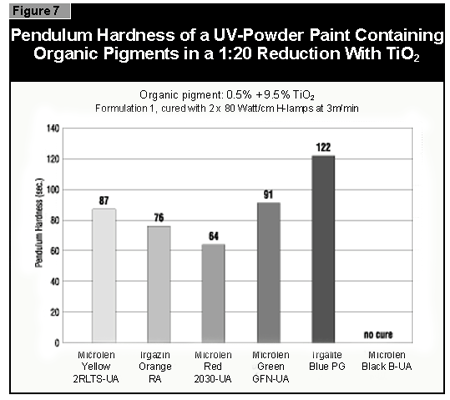
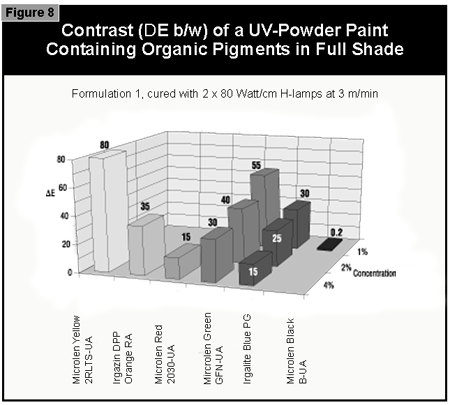
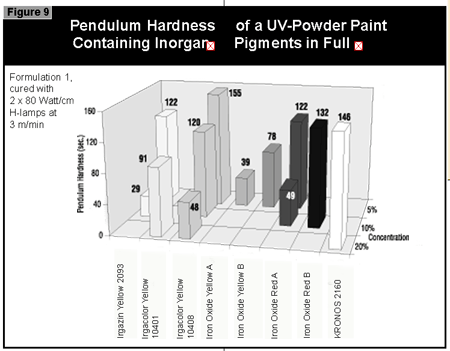
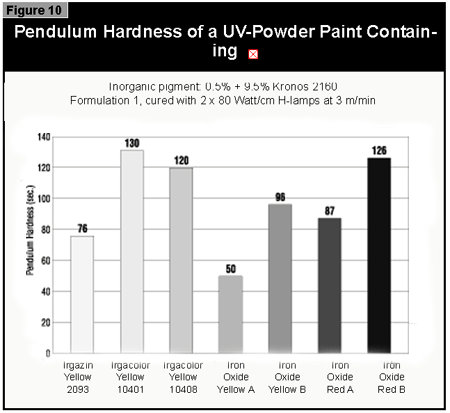
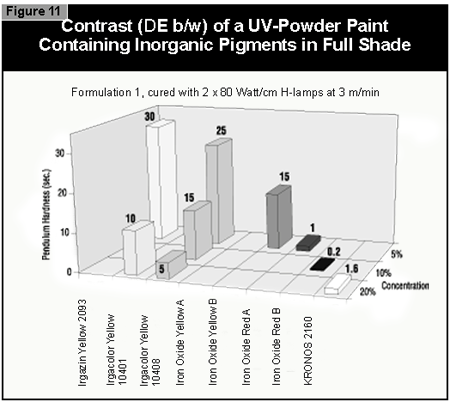
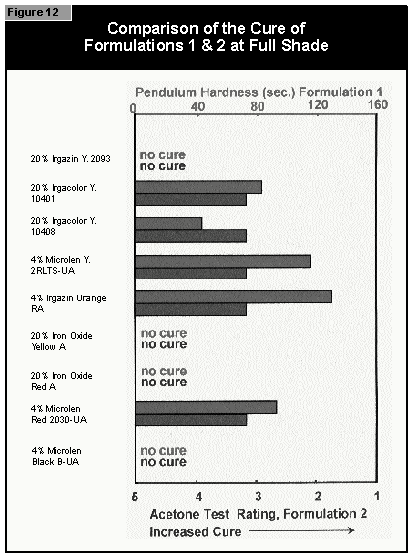
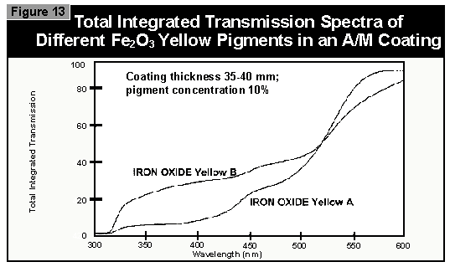
Gallium-doped UV lamps have a spectral output that is greater in the visible region of the spectrum than undoped mercury-vapor UV lamps. It was therefore expected that a better through-cure would be obtained for the yellow pigments, which absorb at the same wavelength as the BAPO photoinitiator (3). When pigmented coatings were cured using either two gallium-doped lamps on their own or the combination of a gallium-doped lamp and an undoped lamp, only minimal improvement of the pendulum hardness was found. This was well below the limits of error (±15 sec.) of the pendulum hardness measurements. It is possible that differences in lamp output and the geometry and construction of the exposure equipment are of importance. Other workers report better cure results with the gallium-doped lamps in white (3) and gray (4) pigmented systems, but this was not found with the equipment used in this study.
The question arises as to why some pigments allow better UV-curing than others, even though the colors of the pigments are nearly identical. For optimal cure, a maximum amount of light in the region of the spectrum at which the photoinitiators absorb must reach the base of the coating. This is a complex problem because the extent of cure depends on:
- spectral output of the lamp
- absorption spectra of the photoinitiators
- quantum yield for radical formation of the photoinitiators--this can be expected to be wavelength dependent
- absorption of light of the wavelength range of interest by all species other than the photoinitiator
- extent of light scattering by the pigment. This increases the path length of the light through the coating and hence the probability of absorption.
- reflectivity of the coating substrate.
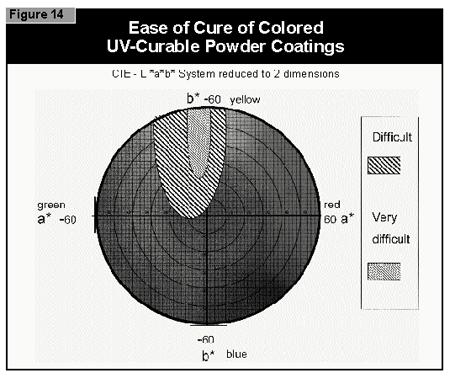

Summary and Recommendations
In general, the most difficult color to cure is yellow. At present, it is not possible to cure this color if a high color strength is required. In reductions with TiO2, it is possible to cure yellows at low color strength. The neighboring colors in the color space diagram (orange, brown, some greens and gray) are also difficult to cure (Figure 14).Reds and blues, however, are relatively easy to cure. Black pigments tend to have good hiding power and can be used in UV-curable powder coatings, but only at concentrations less than 1%. At 0.5% pigment concentration, a pendulum hardness of 123 was achieved. Figure 15 gives recom mendations for pigments for use in powder coatings both in reductions with TiO2 and at full shade.
In curing colored pigmented powder coatings, it is essential that enough light can be absorbed by the photoinitiator at the bottom of the coating. A higher ratio of BAPO:AHK of 4:1 is therefore recommended, rather than the 1:1 ratio commonly used for white pigmented systems, but at the same total concentration of 2.5%.
This paper was originally presented at Powder Coating Europe 2000, sponsored by Vincentz Verlag.
Looking for a reprint of this article?
From high-res PDFs to custom plaques, order your copy today!