Harnessing the Cleaning Power of Dry Ice
Dry ice blasting can safely remove coatings and contaminants from surfaces without the secondary waste treatment and disposal often required with pretreatment chemicals and other cleaning methods. As a result, it offers benefits such as cost reductions, extension of equipment's useful life, minimized substrate damage and improved environmental safety compared to other cleaning methods.

In the early 1930s, the manufacture of solid phase carbon dioxide (CO2) became possible. During this time, the creation of “dry ice” was nothing more than a laboratory experiment. As the procedure for making dry ice became readily available, applications for this innovative substance grew. The first use was in refrigeration, and dry ice is still widely used in the food industry for packaging and protecting perishable foods today.
1945 saw stories of the U.S. Navy experimenting with dry ice as a blast media for various degreasing applications. In November 1972, Edwin Rice received a patent for his “method for the removal of unwanted portions of an article by spraying with high-velocity dry ice particles.” Similarly, in August 1977, Calvin Fong received a patent on “sandblasting with pellets of material capable of sublimation.”
Dry ice pelletizers and dry ice blasting machines entered the industrial markets in the late 1980s. At that time, the blasting machines were physically large, expensive and required high air pressure for operation (pressures greater than 200 psi or 13.8 bar). As the CO2/dry ice blasting technology advanced, the dry ice blasting machines’ size and cost dropped. Today, the latest nozzle technology has made blasting effective at shop air pressures (80 psi or 5.5 bar).

With a low temperature of -109°F (-78°C), dry ice has an inherent thermal energy ready to be tapped. At atmospheric pressure, dry ice sublimates directly to vapor without going through a liquid phase. This unique property means that the blast media simply disappears, leaving only the original contaminant to be disposed of. In addition, using dry ice allows blast cleaning to be carried out in water-sensitive areas.
The grade of carbon dioxide used in dry ice blasting is the same as that used in the food and beverage industry and has been specifically approved by the U.S. Food and Drug Administration (FDA), the Environmental Protection Agency (EPA) and the U.S. Department of Agriculture (USDA). Carbon dioxide is a non-poisonous, liquefied gas that is both inexpensive and easily stored at work sites. Of equal importance is its non-conductive and non-flammable nature.
CO2 is a natural byproduct of several industrial manufacturing processes such as fermentation and petrol-chemical refining. The CO2 given off by the above production processes is captured and stored without losses until needed. When the CO2 is returned to the atmosphere during the blasting process, no new CO2 is produced. Instead, only the original CO2 byproduct is released.
Table 1 lists the physical properties and conversion factors for CO2 in its various forms.
Another technique is to manufacture hard pellets of dry ice in a pelletizer and then immediately blast with the pellets or store the pellets in an insulated container until they are needed. These dry ice pellets are generally 0.08 to 0.12 in. (0.2 to 0.3 cm) in diameter and 0.1 to 0.4 in. (0.25 to 1 cm) in length.
Pelletized dry ice is manufactured by flashing pressurized liquid CO2 into snow, and then compressing the snow into solid form. The snow is either directly nuggetized into pellets (mechanical compression) or is extruded into solid pellet form through a die under hydraulic pressure. The latter process allows for a more efficient conversion from the liquid phase to the solid phase. Generally, it is desirable to have dry ice pellets that are well compacted to minimize the entrapment of gaseous CO2 and/or air, which can affect product quality.
As seen in Table 1, the yield achieved when flashing liquid carbon dioxide into snow increases as the temperature of the liquid CO2 decreases, so it is important to pre-chill the incoming liquid CO2 via heat exchangers with the outgoing CO2 vapor.
Several manufacturers make dry ice pelletizers, which may prove beneficial to have on-site for companies with a high pellet demand. Facilities required for such an arrangement generally include a refrigerated liquid CO2 tank, a pelletizer and liquid CO2 lines to reach the equipment.
Some manufacturers make combined dry ice pelletizer/blast machines, which manufacture the dry ice and then blast it all in one operation. Facilities required for this type of arrangement include an air compressor (typically either 120 psi at 250 scfm/8.3 bar at 7.1 m3/min, or 350 psi at 250 scfm/24.1 bar at 7.1 m3/min), a liquid CO2 tank, a pelletizer/blast machine, a compressed air hose and liquid CO2 lines to reach the equipment, a blast hose from the machine to the blasting operation, and the appropriate nozzle(s) for the application. This equipment is best suited to high-volume, continuous dry ice blasting applications where the cost savings of manufacturing pellets on-site justifies the capital expenditure for the system.
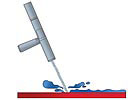
As with other blast media, the kinetic energy associated with dry ice blasting is a function of the mass density and impact velocity of the particles. Since CO2/dry ice particles have a relatively low hardness, the process relies on high particle velocities to achieve the needed impact energy. The high particle velocities are the result of supersonic propellant or airstream velocities.
Unlike other blast media, the CO2/dry ice particles have a very low temperature of -109° F (-78.3°C). This inherently low temperature gives the dry ice blasting process unique thermodynamically induced surface mechanisms that affect the coating or contaminate in greater or lesser degrees, depending on the coating type. Because of the temperature differential between the dry ice particles and the surface being treated, a phenomenon known as “fracking” or thermal shock can occur. As a material’s temperature decreases, the material becomes embrittled, enabling the particle impact to break up the coating (see Figures 1 and 2).
The thermal gradient or differential between two dissimilar materials with different thermal expansion coefficients also can serve to break the bond between the two materials. This thermal shock is most evident when blasting a non-metallic coating or contaminant bonded to a metallic substrate.
Quite often, companies examining the dry ice blasting process are concerned with the effect the thermal shock will have on the parent metal. Studies have shown that the temperature decrease occurs on the surface only, so there is no chance of thermal stress occurring in the substrate metal.
To illustrate this principle, an experiment was performed where thermocouples were embedded into a steel substrate at varying depths (flush with the surface to 2 mm deep). A CO2/dry ice blast jet was constantly swept across the test specimen for 30 seconds (a relatively long dwell time for this process), and the thermocouples recorded the changing temperatures at the various depths. The surface-mounted thermocouple showed a temperature drop each time the blast jet passed directly upon it (50°C in about 5 seconds). In contrast, the thermocouples embedded at various depths in the substrate recorded a slow gradual drop in temperature corresponding to the overall test plate temperature drop. The thermocouple 2 mm deep only dropped 10°C after 30 seconds. This experiment illustrated that the thermal shock occurs only at the surface where the coating or contaminant is bonded to the substrate and has no detrimental effect on the substrate.1
Even at high impact velocities and direct “head-on” impact angles, the kinetic effect of solid CO2/dry ice particles is minimal when compared to other media (grit, sand, PMB, etc.). This is due to the relative lack of hardness of the dry ice particles and the almost instantaneous phase change to a gas on impact, which effectively provides an almost nonexistent coefficient of restitution in the impact equation. Because dry ice blasting is considered non-abrasive and relies on the thermal effects discussed above, the process may be applied to a wide range of materials without damage. Soft metals such as brass and aluminum cladding can be dry ice blasted for the removal of coatings or contaminates without creating surface stresses (pinging), pitting or roughness.
In the two-hose system, dry ice particles are delivered and metered by various mechanical means to the inlet end of a hose and are drawn through the hose to the nozzle by means of vacuum produced by an ejector-type nozzle. Inside the nozzle, a stream of compressed air (supplied by the second hose) is sent through a primary nozzle and expands as a high velocity jet confined inside a mixing tube. When flow areas are properly sized, this type of nozzle produces a vacuum on the cavity around the primary jet and can therefore draw particles up through the ice hose and into the mixing tube, where they are accelerated as the jet mixes with the entrained air/dry ice particle mixture. The exhaust Mach number from this type of nozzle is, in general, slightly supersonic. The advantages of this type of system are relative simplicity and lower material cost, along with an overall compact feeder system. One primary disadvantage is that the associated nozzle technology is generally not adaptable to a wide range of conditions (i.e., tight turns in a cavity, thin-wide blast swaths, etc.). Also, the aggression level and strip rate of the two-hose system is less than comparable to single-hose blast machines.
In a single-hose system, particles are fed into the compressed air line by one of several types of airlock mechanisms. Reciprocating and rotary airlocks are both used in the industry. The stream of pellets and compressed air is then fed directly into a single hose, followed by a nozzle where both air and pellets accelerate to high velocities. The exhaust Mach number from this type of nozzle is generally in the 1.7 to 3.0 range, depending on design and blast pressure. Advantages of this type of system are wide nozzle adaptability and the highest available blast aggression levels. Disadvantages include relatively higher material cost due to the complex airlock mechanism.
Dry ice blasting machines can be further differentiated as either dry ice block shavers or dry ice pellet blasters.
Block Shavers. The block shaver machines take standard 60 lb (27.3 kg) dry ice blocks and use rotating blades to shave a thin layer of ice off the block. This thin sheet of dry ice shatters under its own weight into sugar grain sized dry ice particles. These particles then fall into a funnel for collection. A two-hose delivery system is used to transfer the particles at the bottom of the funnel to the surface to be cleaned. The low mass of these particles combined with the inefficient two-hose system limits the block shavers to light-duty cleaning. Because the shaved ice machines deliver a dry ice particle blast with high flux density (a high number of particles striking a square area of surface per second), they are effective on thin, moderately hard coatings, such as an air-dried oil based paint. The disadvantage of the ice shaver is that the dry ice particle’s size and flux density, as well as its velocity, are fixed.
Pellet Blasters. Pellet blasting machines have a hopper that is filled with pre-manufactured CO2/dry ice pellets. The hopper uses mechanical agitation to move the pellets to the bottom of the hopper and into the feeder system. As stated earlier, the pellets are extruded through a die plate under great pressure. This design creates an extremely dense pellet for maximum impact energy. The pellets are available in several sizes, ranging from 0.04 to 0.12 in. (0.1 to 0.3 cm) in diameter. With a single-hose delivery system, the final pellet size and blast flux density exiting the nozzle is governed by the type of blast hose (hose diameter and interior wall roughness) and nozzle used. Because of its design, the single-hose dry ice pellet blasting units are capable of “dialing-in” the correct blast type needed for a wide range of individual coating or contaminant removal requirements.
For example, soft coatings such as rubber, silicone, foams and waxes, and release agents need large dry ice pellets with a low flux density for maximum strip rate and efficiency. These coatings require maximum thermal energy (i.e., dry ice pellets with large mass) and large spacing between the pellets (i.e., low flux density) for optimum cleaning performance. In contrast, hard coatings such as paints, varnishes, carbon buildup, etc., require a smaller particle size with a high flux density and a high particle velocity.
Another way to categorize dry ice blasting machines is by their feed mechanism, which is typically either all-pneumatic or electro-pneumatic.
All-pneumatic machines have a pneumatically operated dry ice particle feed mechanism and controls. This may include the use of air motors. The advantage of such a machine is the availability of compressed air at the blast locations, especially outdoors. One disadvantage is that the operation of the machine may be susceptible to disruption due to moisture or contamination in the compressed air supply. In addition, these machines are more prone to freeze-ups and are better suited for light-duty spot cleaning applications. Also, if the machine is powered by an air motor, it will have a continuous exhaust of oily air. This same air motor can easily be flooded with water if the air system is not adequately dried.
Electro-pneumatic machines are truly environmentally friendly because they emit no oily exhaust and they are more tolerant of moisture and contaminants in the air supply. The electro-pneumatic machines rarely freeze up, which makes them ideal for automated line applications where around-the-clock dry ice blasting is required. Also, these machines provide pulse free blasting for uniform cleaning and efficient use of the dry ice. However, a slight inconvenience factor is associated with supplying both electrical power and compressed air to the machine at each blast location.
One of the most challenging technologies associated with either an all-pneumatic or electro-pneumatic blast machine is the achievement of a smooth, continuous pellet feed. One surprising property of dry ice is that it is not smooth or slippery like water ice, nor is it smooth-flowing like sand or glass beads. Instead, it is somewhat resistant to flow. Because of this, dry ice blast machines tend to have various agitators, augers and other devices in the hopper to improve pellet flow. Generally, the poorer the quality of the dry ice - for example, if it contains water ice buildup or a large percentage of CO2 “fines” or snow - the more difficult its flow will be through a system. An additional property of dry ice is that it is extremely cold and will draw moisture out of the surrounding air in the form of frost. Therefore, the machine must be tolerant of repeated freeze-thaw cycles and the associated moisture accumulation that will take place over time.
Generally, the difference between a high-quality dry ice blasting machine and a mediocre one lies in the unit’s ability to do a cleaning job quickly, cost-effectively, and with the reliability of smooth and continuous dry ice pellet flow under real-world conditions.

Since the size of the dry ice particles affects cleaning performance, a dry ice blasting system should have the flexibility to “dial-in” the correct particle size. This can be done in a couple of different ways. First, the size of the dry ice pellet being produced by the pelletizer may be varied. Once the pellet is in the dry ice blasting machine’s hopper, the size of the pellet reaching the surface to be cleaned can be changed in several ways. The diameter and type of blast hose used will either keep the pellet intact or break it up into smaller particles. Also, the nozzle may be intentionally mis-expanded to produce partially destructive shockwaves within the nozzle. Both techniques are used independently or together to optimize the dry ice particle size, blast stream velocity, and flux density for any cleaning job.
When sand or any similar media with a very small diameter is used in blasting, the size of the nozzle throat is very large compared to the blast media. In dry ice blasting, however, the nozzle throat may only be slightly larger than the dry ice particle being accelerated.
Table 2 indicates the approximate size of a round nozzle throat for four different levels of blast pressure at a constant airflow of 200 scfm/5.7 m3/min, a typical flow rate available for blasting operations. At higher pressures, the dry ice particle size needs to be smaller to correspond with the smaller throat size. The high-pressure blast stream is described as high-velocity small particles with a high flux density. Again, this particle blast profile is best suited for removing hard coatings such as paint. Table 2 also shows a larger nozzle throat diameter corresponding to low-pressure operations. As stated above, large pellets impacting the surface with low flux density is ideal for cleaning soft coatings.
Dry ice blasting nozzles tend to be long as a result of the requirement to accelerate particles to as high a velocity as possible. Therefore, a very long nozzle with a small throat tends to have a high scrubbing-surface area per unit of airflow. This effect explains the higher efficiency of low-pressure dry ice nozzles compared to high-pressure nozzles. A minimum-cost dry ice blasting system for industrial use has a design point at 80 psi/5.5 bar, a typical pressure for a plant air system.

In the early 1930s, the manufacture of solid phase carbon dioxide (CO2) became possible. During this time, the creation of “dry ice” was nothing more than a laboratory experiment. As the procedure for making dry ice became readily available, applications for this innovative substance grew. The first use was in refrigeration, and dry ice is still widely used in the food industry for packaging and protecting perishable foods today.
1945 saw stories of the U.S. Navy experimenting with dry ice as a blast media for various degreasing applications. In November 1972, Edwin Rice received a patent for his “method for the removal of unwanted portions of an article by spraying with high-velocity dry ice particles.” Similarly, in August 1977, Calvin Fong received a patent on “sandblasting with pellets of material capable of sublimation.”
Dry ice pelletizers and dry ice blasting machines entered the industrial markets in the late 1980s. At that time, the blasting machines were physically large, expensive and required high air pressure for operation (pressures greater than 200 psi or 13.8 bar). As the CO2/dry ice blasting technology advanced, the dry ice blasting machines’ size and cost dropped. Today, the latest nozzle technology has made blasting effective at shop air pressures (80 psi or 5.5 bar).

What Is Dry Ice?
Dry ice is the solid form of CO2, which is a colorless, tasteless, odorless gas found naturally in the atmosphere. Though it is present in relatively small quantities (about 0.03% by volume), it is one of the most important gases in existence.With a low temperature of -109°F (-78°C), dry ice has an inherent thermal energy ready to be tapped. At atmospheric pressure, dry ice sublimates directly to vapor without going through a liquid phase. This unique property means that the blast media simply disappears, leaving only the original contaminant to be disposed of. In addition, using dry ice allows blast cleaning to be carried out in water-sensitive areas.
The grade of carbon dioxide used in dry ice blasting is the same as that used in the food and beverage industry and has been specifically approved by the U.S. Food and Drug Administration (FDA), the Environmental Protection Agency (EPA) and the U.S. Department of Agriculture (USDA). Carbon dioxide is a non-poisonous, liquefied gas that is both inexpensive and easily stored at work sites. Of equal importance is its non-conductive and non-flammable nature.
CO2 is a natural byproduct of several industrial manufacturing processes such as fermentation and petrol-chemical refining. The CO2 given off by the above production processes is captured and stored without losses until needed. When the CO2 is returned to the atmosphere during the blasting process, no new CO2 is produced. Instead, only the original CO2 byproduct is released.
Table 1 lists the physical properties and conversion factors for CO2 in its various forms.
Media Manufacture
In dry ice blasting, several methods are used to manufacture the blasting media. One technique is to shave dry ice granules from a solid CO2 (dry ice) block at the blasting machine. This method generally produces sugar-crystal-sized dry ice granules, which must be used quickly due to rapid sublimation (a result of the granules’ high surface area-to-volume ratio).Another technique is to manufacture hard pellets of dry ice in a pelletizer and then immediately blast with the pellets or store the pellets in an insulated container until they are needed. These dry ice pellets are generally 0.08 to 0.12 in. (0.2 to 0.3 cm) in diameter and 0.1 to 0.4 in. (0.25 to 1 cm) in length.
Pelletized dry ice is manufactured by flashing pressurized liquid CO2 into snow, and then compressing the snow into solid form. The snow is either directly nuggetized into pellets (mechanical compression) or is extruded into solid pellet form through a die under hydraulic pressure. The latter process allows for a more efficient conversion from the liquid phase to the solid phase. Generally, it is desirable to have dry ice pellets that are well compacted to minimize the entrapment of gaseous CO2 and/or air, which can affect product quality.
As seen in Table 1, the yield achieved when flashing liquid carbon dioxide into snow increases as the temperature of the liquid CO2 decreases, so it is important to pre-chill the incoming liquid CO2 via heat exchangers with the outgoing CO2 vapor.
Several manufacturers make dry ice pelletizers, which may prove beneficial to have on-site for companies with a high pellet demand. Facilities required for such an arrangement generally include a refrigerated liquid CO2 tank, a pelletizer and liquid CO2 lines to reach the equipment.
Some manufacturers make combined dry ice pelletizer/blast machines, which manufacture the dry ice and then blast it all in one operation. Facilities required for this type of arrangement include an air compressor (typically either 120 psi at 250 scfm/8.3 bar at 7.1 m3/min, or 350 psi at 250 scfm/24.1 bar at 7.1 m3/min), a liquid CO2 tank, a pelletizer/blast machine, a compressed air hose and liquid CO2 lines to reach the equipment, a blast hose from the machine to the blasting operation, and the appropriate nozzle(s) for the application. This equipment is best suited to high-volume, continuous dry ice blasting applications where the cost savings of manufacturing pellets on-site justifies the capital expenditure for the system.
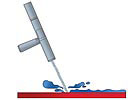
Figure 1. Thermal shock induces micro-cracking in the surface coating.
How Does Dry Ice Blasting Work?
Dry ice blasting is similar to sand blasting, plastic bead blasting or soda blasting in that a media is accelerated in a pressurized air stream (or other inert gas) to impact the surface being cleaned or prepared. With dry ice blasting, the media is solid CO2 particles. One unique aspect of using dry ice particles as a blast media is that the particles sublimate (vaporize) upon impact with the surface. The combined impact-energy dissipation and extremely rapid heat transfer between the dry ice pellet and the surface causes the instantaneous sublimation of the solid CO2/dry ice into gas. The gas then expands to nearly 800 times the volume of the dry ice pellet in a few milliseconds in what is effectively a “micro-explosion” at the point of impact. Because of the solid CO2 vaporizing, the dry ice blasting process does not generate any secondary waste. All that remains to be collected is the contaminant being removed.As with other blast media, the kinetic energy associated with dry ice blasting is a function of the mass density and impact velocity of the particles. Since CO2/dry ice particles have a relatively low hardness, the process relies on high particle velocities to achieve the needed impact energy. The high particle velocities are the result of supersonic propellant or airstream velocities.
Unlike other blast media, the CO2/dry ice particles have a very low temperature of -109° F (-78.3°C). This inherently low temperature gives the dry ice blasting process unique thermodynamically induced surface mechanisms that affect the coating or contaminate in greater or lesser degrees, depending on the coating type. Because of the temperature differential between the dry ice particles and the surface being treated, a phenomenon known as “fracking” or thermal shock can occur. As a material’s temperature decreases, the material becomes embrittled, enabling the particle impact to break up the coating (see Figures 1 and 2).

Figure 2. CO2 gas expansion and pellet kinetic effects break away and remove the coating particles.
Quite often, companies examining the dry ice blasting process are concerned with the effect the thermal shock will have on the parent metal. Studies have shown that the temperature decrease occurs on the surface only, so there is no chance of thermal stress occurring in the substrate metal.
To illustrate this principle, an experiment was performed where thermocouples were embedded into a steel substrate at varying depths (flush with the surface to 2 mm deep). A CO2/dry ice blast jet was constantly swept across the test specimen for 30 seconds (a relatively long dwell time for this process), and the thermocouples recorded the changing temperatures at the various depths. The surface-mounted thermocouple showed a temperature drop each time the blast jet passed directly upon it (50°C in about 5 seconds). In contrast, the thermocouples embedded at various depths in the substrate recorded a slow gradual drop in temperature corresponding to the overall test plate temperature drop. The thermocouple 2 mm deep only dropped 10°C after 30 seconds. This experiment illustrated that the thermal shock occurs only at the surface where the coating or contaminant is bonded to the substrate and has no detrimental effect on the substrate.1
Even at high impact velocities and direct “head-on” impact angles, the kinetic effect of solid CO2/dry ice particles is minimal when compared to other media (grit, sand, PMB, etc.). This is due to the relative lack of hardness of the dry ice particles and the almost instantaneous phase change to a gas on impact, which effectively provides an almost nonexistent coefficient of restitution in the impact equation. Because dry ice blasting is considered non-abrasive and relies on the thermal effects discussed above, the process may be applied to a wide range of materials without damage. Soft metals such as brass and aluminum cladding can be dry ice blasted for the removal of coatings or contaminates without creating surface stresses (pinging), pitting or roughness.
Blast Machine Types
Dry ice blasting machines are characterized by their method of transporting pellets to the nozzle. The two general classes are the two-hose and the single-hose systems. In either type of system, the proper selection of blast hose is important because of the low temperatures involved and the need to preserve particle integrity as the dry ice particles travel through the hose.In the two-hose system, dry ice particles are delivered and metered by various mechanical means to the inlet end of a hose and are drawn through the hose to the nozzle by means of vacuum produced by an ejector-type nozzle. Inside the nozzle, a stream of compressed air (supplied by the second hose) is sent through a primary nozzle and expands as a high velocity jet confined inside a mixing tube. When flow areas are properly sized, this type of nozzle produces a vacuum on the cavity around the primary jet and can therefore draw particles up through the ice hose and into the mixing tube, where they are accelerated as the jet mixes with the entrained air/dry ice particle mixture. The exhaust Mach number from this type of nozzle is, in general, slightly supersonic. The advantages of this type of system are relative simplicity and lower material cost, along with an overall compact feeder system. One primary disadvantage is that the associated nozzle technology is generally not adaptable to a wide range of conditions (i.e., tight turns in a cavity, thin-wide blast swaths, etc.). Also, the aggression level and strip rate of the two-hose system is less than comparable to single-hose blast machines.
In a single-hose system, particles are fed into the compressed air line by one of several types of airlock mechanisms. Reciprocating and rotary airlocks are both used in the industry. The stream of pellets and compressed air is then fed directly into a single hose, followed by a nozzle where both air and pellets accelerate to high velocities. The exhaust Mach number from this type of nozzle is generally in the 1.7 to 3.0 range, depending on design and blast pressure. Advantages of this type of system are wide nozzle adaptability and the highest available blast aggression levels. Disadvantages include relatively higher material cost due to the complex airlock mechanism.
Dry ice blasting machines can be further differentiated as either dry ice block shavers or dry ice pellet blasters.
Block Shavers. The block shaver machines take standard 60 lb (27.3 kg) dry ice blocks and use rotating blades to shave a thin layer of ice off the block. This thin sheet of dry ice shatters under its own weight into sugar grain sized dry ice particles. These particles then fall into a funnel for collection. A two-hose delivery system is used to transfer the particles at the bottom of the funnel to the surface to be cleaned. The low mass of these particles combined with the inefficient two-hose system limits the block shavers to light-duty cleaning. Because the shaved ice machines deliver a dry ice particle blast with high flux density (a high number of particles striking a square area of surface per second), they are effective on thin, moderately hard coatings, such as an air-dried oil based paint. The disadvantage of the ice shaver is that the dry ice particle’s size and flux density, as well as its velocity, are fixed.
Pellet Blasters. Pellet blasting machines have a hopper that is filled with pre-manufactured CO2/dry ice pellets. The hopper uses mechanical agitation to move the pellets to the bottom of the hopper and into the feeder system. As stated earlier, the pellets are extruded through a die plate under great pressure. This design creates an extremely dense pellet for maximum impact energy. The pellets are available in several sizes, ranging from 0.04 to 0.12 in. (0.1 to 0.3 cm) in diameter. With a single-hose delivery system, the final pellet size and blast flux density exiting the nozzle is governed by the type of blast hose (hose diameter and interior wall roughness) and nozzle used. Because of its design, the single-hose dry ice pellet blasting units are capable of “dialing-in” the correct blast type needed for a wide range of individual coating or contaminant removal requirements.
For example, soft coatings such as rubber, silicone, foams and waxes, and release agents need large dry ice pellets with a low flux density for maximum strip rate and efficiency. These coatings require maximum thermal energy (i.e., dry ice pellets with large mass) and large spacing between the pellets (i.e., low flux density) for optimum cleaning performance. In contrast, hard coatings such as paints, varnishes, carbon buildup, etc., require a smaller particle size with a high flux density and a high particle velocity.
Another way to categorize dry ice blasting machines is by their feed mechanism, which is typically either all-pneumatic or electro-pneumatic.
All-pneumatic machines have a pneumatically operated dry ice particle feed mechanism and controls. This may include the use of air motors. The advantage of such a machine is the availability of compressed air at the blast locations, especially outdoors. One disadvantage is that the operation of the machine may be susceptible to disruption due to moisture or contamination in the compressed air supply. In addition, these machines are more prone to freeze-ups and are better suited for light-duty spot cleaning applications. Also, if the machine is powered by an air motor, it will have a continuous exhaust of oily air. This same air motor can easily be flooded with water if the air system is not adequately dried.
Electro-pneumatic machines are truly environmentally friendly because they emit no oily exhaust and they are more tolerant of moisture and contaminants in the air supply. The electro-pneumatic machines rarely freeze up, which makes them ideal for automated line applications where around-the-clock dry ice blasting is required. Also, these machines provide pulse free blasting for uniform cleaning and efficient use of the dry ice. However, a slight inconvenience factor is associated with supplying both electrical power and compressed air to the machine at each blast location.
One of the most challenging technologies associated with either an all-pneumatic or electro-pneumatic blast machine is the achievement of a smooth, continuous pellet feed. One surprising property of dry ice is that it is not smooth or slippery like water ice, nor is it smooth-flowing like sand or glass beads. Instead, it is somewhat resistant to flow. Because of this, dry ice blast machines tend to have various agitators, augers and other devices in the hopper to improve pellet flow. Generally, the poorer the quality of the dry ice - for example, if it contains water ice buildup or a large percentage of CO2 “fines” or snow - the more difficult its flow will be through a system. An additional property of dry ice is that it is extremely cold and will draw moisture out of the surrounding air in the form of frost. Therefore, the machine must be tolerant of repeated freeze-thaw cycles and the associated moisture accumulation that will take place over time.
Generally, the difference between a high-quality dry ice blasting machine and a mediocre one lies in the unit’s ability to do a cleaning job quickly, cost-effectively, and with the reliability of smooth and continuous dry ice pellet flow under real-world conditions.

Nozzle Technology
The nozzle is where the dry ice particles are accelerated to the highest velocity possible to create an effective dry ice blast stream. Tests comparing two-hose ejector nozzles to single-hose convergent-divergent supersonic nozzles operating under the same cond itions (i.e., air volume, pressure, temperature, CO2 particle mass, etc.) showed a significantly higher efficiency capability for the single-hose type nozzles. This difference in capability is directly related to the two-hose ejector nozzle’s overall supplied energy being used not only to accelerate the CO2/dry ice particles, but also to create the vacuum pulling the secondary pellet flow through the secondary hose. More energy is drained to mix this low-velocity particle flow with the high-velocity jet flow in order to accelerate the dry ice particles through the two-hose nozzle. In simple terms, the net resultant energy available for pellet acceleration is inherently lower for two-hose systems because much of the available energy is lost simply in combining the CO2/dry ice particle flow with the air-jet flow.Since the size of the dry ice particles affects cleaning performance, a dry ice blasting system should have the flexibility to “dial-in” the correct particle size. This can be done in a couple of different ways. First, the size of the dry ice pellet being produced by the pelletizer may be varied. Once the pellet is in the dry ice blasting machine’s hopper, the size of the pellet reaching the surface to be cleaned can be changed in several ways. The diameter and type of blast hose used will either keep the pellet intact or break it up into smaller particles. Also, the nozzle may be intentionally mis-expanded to produce partially destructive shockwaves within the nozzle. Both techniques are used independently or together to optimize the dry ice particle size, blast stream velocity, and flux density for any cleaning job.
When sand or any similar media with a very small diameter is used in blasting, the size of the nozzle throat is very large compared to the blast media. In dry ice blasting, however, the nozzle throat may only be slightly larger than the dry ice particle being accelerated.
Table 2 indicates the approximate size of a round nozzle throat for four different levels of blast pressure at a constant airflow of 200 scfm/5.7 m3/min, a typical flow rate available for blasting operations. At higher pressures, the dry ice particle size needs to be smaller to correspond with the smaller throat size. The high-pressure blast stream is described as high-velocity small particles with a high flux density. Again, this particle blast profile is best suited for removing hard coatings such as paint. Table 2 also shows a larger nozzle throat diameter corresponding to low-pressure operations. As stated above, large pellets impacting the surface with low flux density is ideal for cleaning soft coatings.
Dry ice blasting nozzles tend to be long as a result of the requirement to accelerate particles to as high a velocity as possible. Therefore, a very long nozzle with a small throat tends to have a high scrubbing-surface area per unit of airflow. This effect explains the higher efficiency of low-pressure dry ice nozzles compared to high-pressure nozzles. A minimum-cost dry ice blasting system for industrial use has a design point at 80 psi/5.5 bar, a typical pressure for a plant air system.
Benefits of Dry Ice Blasting Technology
Compared to other cleaning methods, dry ice blasting offers a number of benefits in the finishing industry. These include:- Cost Reductions. The natural sublimation of dry ice particles eliminates the cost of collecting the cleaning media for disposal. Containment and collection costs associated with water/grit blasting procedures are also eliminated. Additionally, because CO2/dry ice blasting systems provide on-line maintenance capabilities for production equipment (on-line cleaning), time-consuming and expensive detooling procedures are kept to a minimum. Dedicated cleaning cycles are no longer required; instead preventive maintenance schedules can be adopted, which allow for equipment cleaning during production periods. As a result, throughput is increased without the addition of labor or production equipment.
- Extension of Equipment’s Useful Life.Unlike sand, walnut shells, plastic beads and other abrasive grit media, dry ice particles are non-abrasive. Cleaning with dry ice will not wear tooling, texture surfaces, open tolerances, or damage bearings or machinery.
- Minimized Substrate Damage. Unlike steam or water blasting, CO2/dry ice blasting is a dry process will not damage electrical wiring, controls or switches. Also, any possible rust formation after cleaning is far less likely with dry ice blasting than with steam or water blasting.
- Environmental Safety. Carbon dioxide is a nontoxic element that meets EPA, FDA and USDA industry guidelines. By replacing toxic chemical processes with CO2/dry ice blasting systems, employee exposure and corporate liability stemming from the use of dangerous chemical cleaning agents can be materially reduced or eliminated completely. Since CO2 gas is heavier than air (CO2 gas displaces oxygen), care must be taken if blasting in enclosed areas or down in a pit.
Links
Looking for a reprint of this article?
From high-res PDFs to custom plaques, order your copy today!