Atomic Force Microscopy Study
Effect of Low-VOC Coalescents on Morphology and Phase Distribution of Latex Films

Today, growing concerns about the negative impact of volatile organic compounds (VOCs) on the environment have motivated the development of stricter regulations that control the VOC content of coatings. Due to this, coalescents with higher molecular weights and boiling points have been developed in order to comply with these regulations and help paint formulators to develop low-VOC paints.
Despite this trend of developing high-boiling-point coalescents there is little information available in the open literature about the drawbacks of using these coalescents. Some drawbacks can arise from the fact that high-boiling-point coalescents may not evaporate completely from the films, and thus negatively affect the properties of the final paints, such as the hardness1 and durability.
Atomic force microscopy (AFM) is a consolidate technique for evaluating the morphological aspects of latex films2,3 as well as the effects of drying conditions and coalescent content on latex film formation.4
In this work, latex films containing three low-VOC coalescents, based on Method 313 of SCAQMD (South Coast Air Quality Management District), were evaluated through AFM, and their impact on film morphology and phase distribution within the films was compared to the impact of a standard high-VOC coalescent.
Experimental
Materials and Methods
Latexes
Commercial vinyl-acrylic and pure acrylic latexes were used in the AFM evaluations.
Coalescents
Three of the coalescents evaluated are commercial and one coalescent is an experimental product. The coalescent descriptions are presented in Table 1.
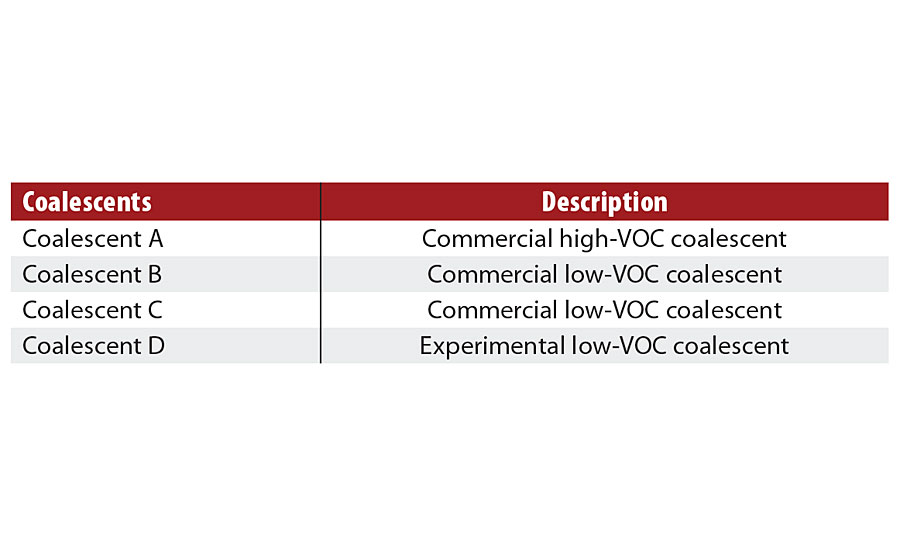
TABLE 1 » Coalescents evaluated in this work.
VOC Content
VOC content within the coalescents was determined according to ASTM 6886 using methyl palmitate as marker as specified by Method 313 of SCAQMD.
Boiling Temperature
The boiling temperature analyses were perfomed according to ABNT NBR 9292.
Density
The density of the coalescents was determined in a Metler DE-40 density meter.
Minimum Film Formation Temperature (MFFT)
The MFFT evaluations of the latexes containing different coalescents were performed according to ASTM D2354. The equipment used was a Rhopoint-MFFT 60.
Hansen Solubility Parameters (HSP)
Coalescent HSP and octanol-water partition coefficients (Log Kow) were estimated using HSP software.
Preparation of Films for AFM Evaluation
Vinyl-acrylic and pure acrylic latexes, containing 8 parts per hundred of polymer (php) of different coalescents were cast on glass substrate, cover slip. The latex films were dried at 25 °C and 50% RH for 3 days before the AFM evaluations.
AFM
AFM images of the surfaces were obtained using a commercial setup (NX-10, Park Systems, Korea). The microscope was operated in intermittent contact mode, slightly below the frequency of resonance, in N2 and at room temperature, using NCLR (Nano sensors) Si probes with spring constant of 48 N.m-1 and resonance frequency within 190 kHz. During scanning, both topographic and phase-contrast images were recorded. Analysis and processing of the AFM images were performed with the Gwyddion software.
Results and Discussions
The vinyl-acrylic and acrylic latexes evaluated in this work are conventional latexes widely used in the American market. The main properties of these latexes are presented in Table 2. Both latexes have typical solid content, particle sizes ranging from 200 to 300 nm, and MFFT lower than their Tg, probably due to the presence of hydrophilic groups within the latex particles that help their hydroplasticization.3-5
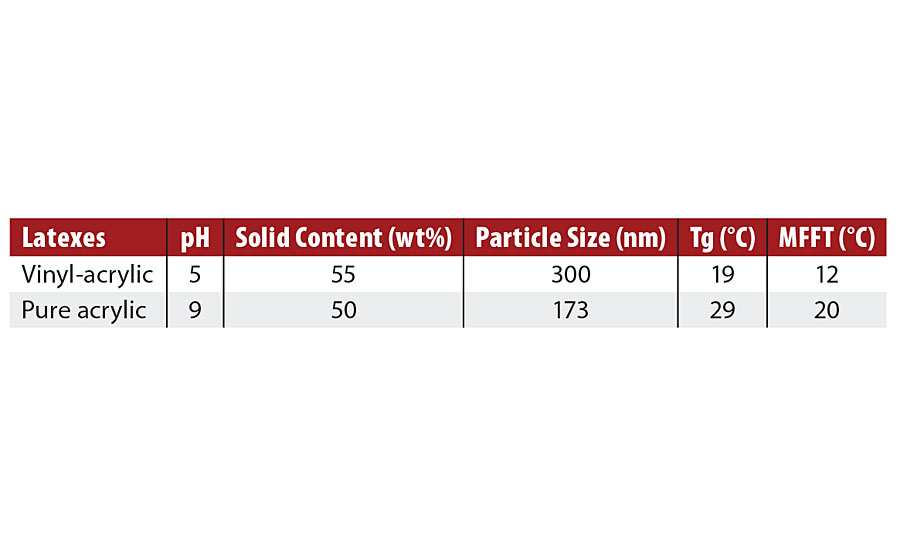
TABLE 2 » Properties of commercial latexes.
Coalescent A is a commercial high-VOC coalescent with high plasticization power and low solubility in water used as reference in this study. The properties of the low-VOC coalescents, Coalescents B-D, and reference high-VOC coalescent, Coalescent A, are presented in Tables 3 and 4.
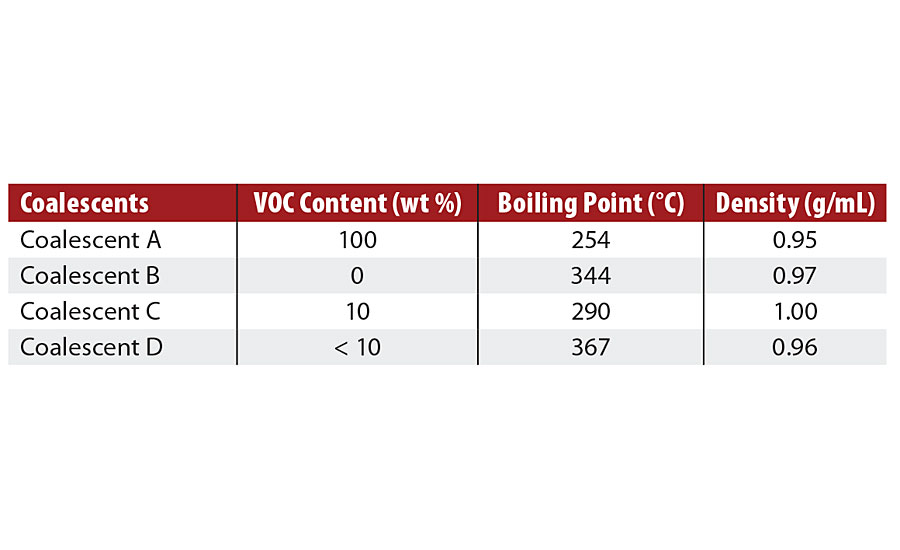
TABLE 3 » Physicochemical characteristics of coalescents.
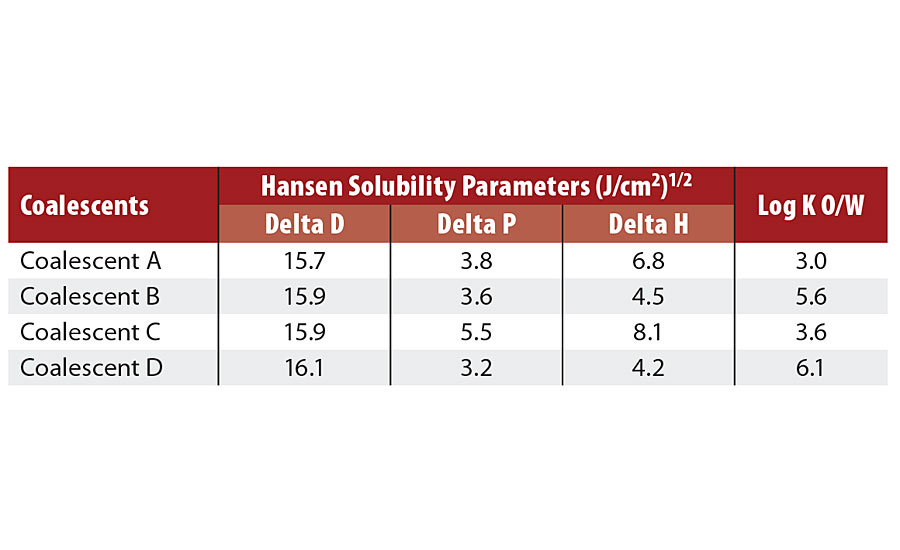
TABLE 4 » Hansen solubility parameters6 of coalescents.
Based on Table 3, the low-VOC coalescents have VOC content lower than 10 wt% based on Method 313 of SCAQMD and boiling point higher than 290 °C.
All coalescents investigated have high dispersion energy (Delta D), which means considerably high hydrophobicity, and most of them (except Coalescent C) have polarity ranging between 3.2-4.5. Coalescent C has the highest polarity and ability to form hidrogen bonds among the coalescents evaluated.
The estimated octanol-water partition coefficients of the low-VOC Coalescents B-D suggest that they have low solubility in water. Previous works5 have shown that even coalescents with high plasticization power but high solubility in water5 or that evaporate too fast from films can be less effective in reducing the MFFT than those coalescents with low solubility in water that evaporate slowly from the films due to the higher concentration of the latter in the polymeric phase.
Figures 1a and 1b present the estimated coalescent concentration required to form film at 0 °C of vinyl-acrylic latex, MFFT of 12 °C, and acrylic latex, MFFT of 20 °C. The estimated concentrations of low-VOC coalescents required to form film at 0 °C were close to the one of Coalescent A. These MFFT results indicate that all low-VOC coalescents have high affinity6 to the polymeric phases of the latexes investigated in this work.
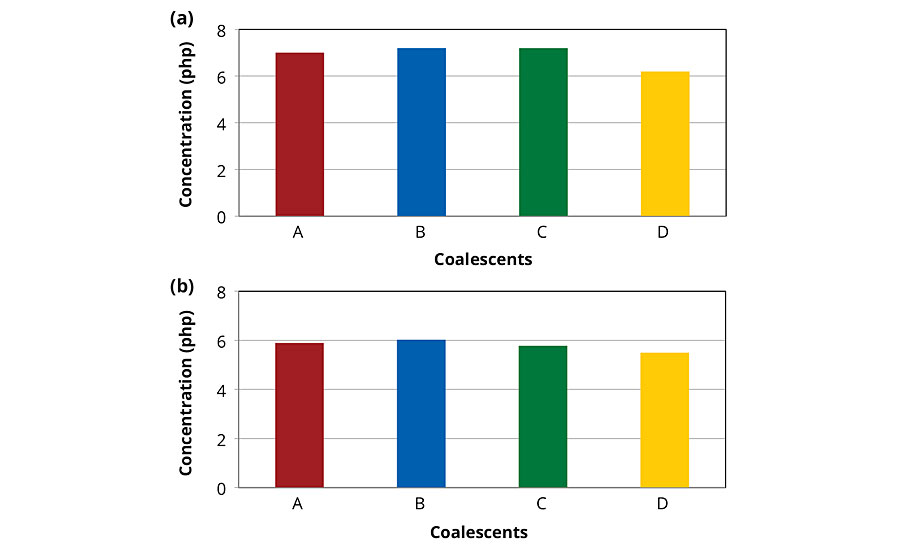
FIGURE 1 » MFFT of vinyl-acrylic (a) and acrylic latexes (b) containing 8 php of coalescents.
The MFFT evaluation is often used for predicting the efficiency of a coalescent to assist the formation of continuous and transparent latex films at a given temperature. Usually, this occurs in Stage 2, considering the accepted mechanism for latex film formation.3,4
- Stage 1: Water evaporates, leading to the concentration, packing and irreversible contact of the latex particles.
- Stage 2: At drying temperatures close to or higher than the MFFT, particle deformation allows a closer contact between them, thus forming void-free transparent films.
- Stage 3: At drying temperatures higher than the polymer Tg there is interdiffusion of polymer chains among the particles, leading to particle coalescence. Film leveling is another event that occurs simultaneously to coalescence, due to polymer chain interdiffusion driven by polymer-air surface tension in order to decrease the film surface energy.
In the industrial world, as Stages 2 and 3 can occur simultaneously, it is a common simplification to assume that the transparent and homogenous latex films obtained at temperature above their MFFT are coalesced and leveled. Due to this, there is a need for complementary evaluations that allow understanding film features on a microscopic level as well as coalescent efficiency in assisting particle coalescence and film leveling. Based on this need, AFM microscopies of vinyl-acrylic and acrylic latex films containing 8 php of Coalescents A-E, formed on glass, dried for 3 days at 25 °C and 50 % of RH, were performed.
Representative AFM images of vinyl-acrylic latex films containing 8 php of coalescents A-D are shown in Figures 2-5.
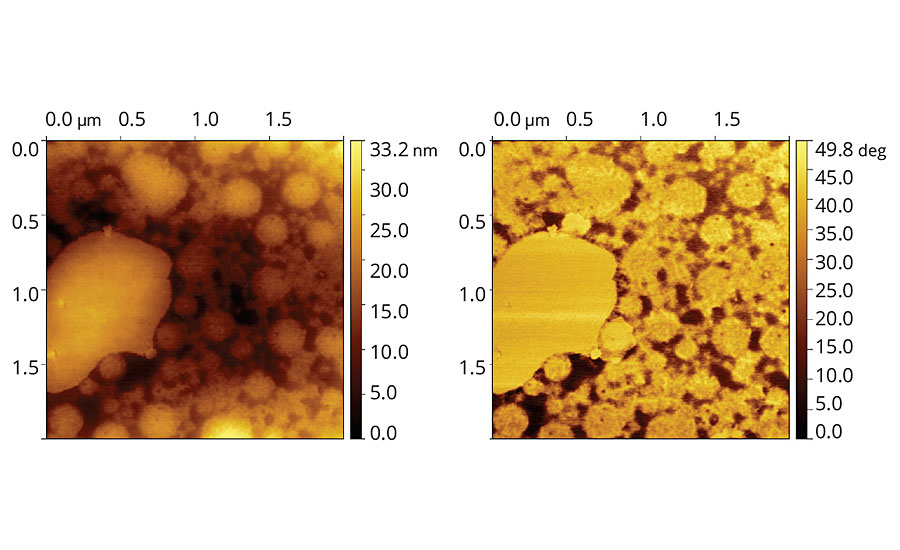
FIGURE 2 » Topographic (left) and phase (right) images of vinyl-acrylic latex film containing 8 php of Coalescent A.
The topographic image of the vinyl-acrylic latex containing Coalescent A, Figure 2, shows particles with distinct particle sizes, particle size ranging from 200 to 1,100 nm, although the film is relatively smooth considering that the maximum height difference along the film is 33 nm, only 10% of the average particle size obtained through light scattering. This feature suggests that the particles are considerably packed and deformed. The phase image suggests accumulation of species with distinct viscoelasticity, in relation to the particles, in the valleys among the particles.
A typical trend can be observed in the topographic and phase images of the vinyl-acrylic latex films containing the low-VOC Coalescent B, presented in Figure 3. The topographic image shows smoother film containing only few large particles in comparison to the film containing Coalescent A. These features suggest that film containing the low-VOC Coalescent B is more coalesced and leveled than the one containing the reference high-VOC Coalescent A. The phase image is also more homogenous in comparison to the film containing Coalescent A.
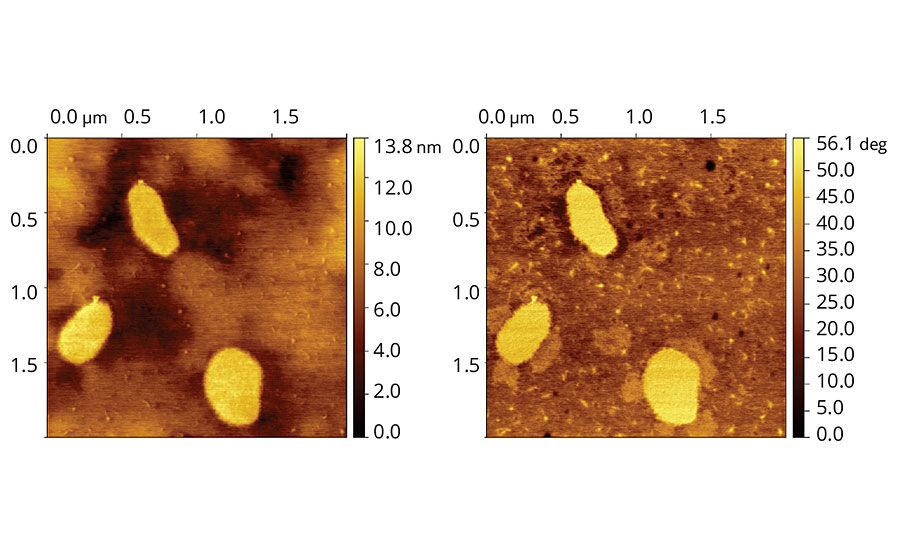
FIGURE 3 » Topographic (left) and phase (right) images of vinyl-acrylic latex film containing 8 php of Coalescent B.
AFM image of film containing Coalescent C, Figure 4, shows no particles and smooth film, suggesting intensive coalescence of particles and film leveling. Phase image shows segregation of species with distinct viscoelasticities along the film.
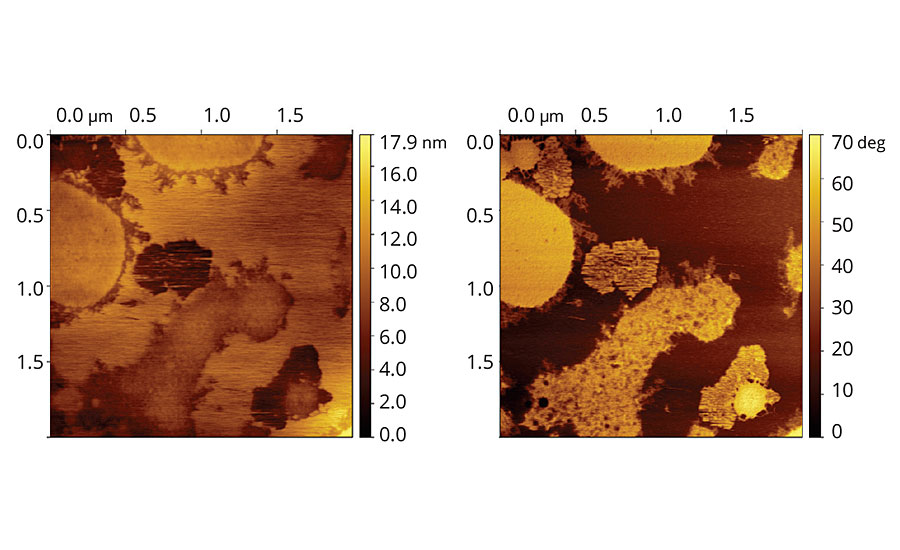
FIGURE 4 » Topographic and phase images of vinyl-acrylic latex film containing Coalescent C.
AFM image of vinyl-acrylic latex with coalescent D, Figure 5, shows considerably smooth and leveled film, although it is possible to identify some small particles along the film. Phase image also shows segregation of species with distinct viscoelasticities along the film.
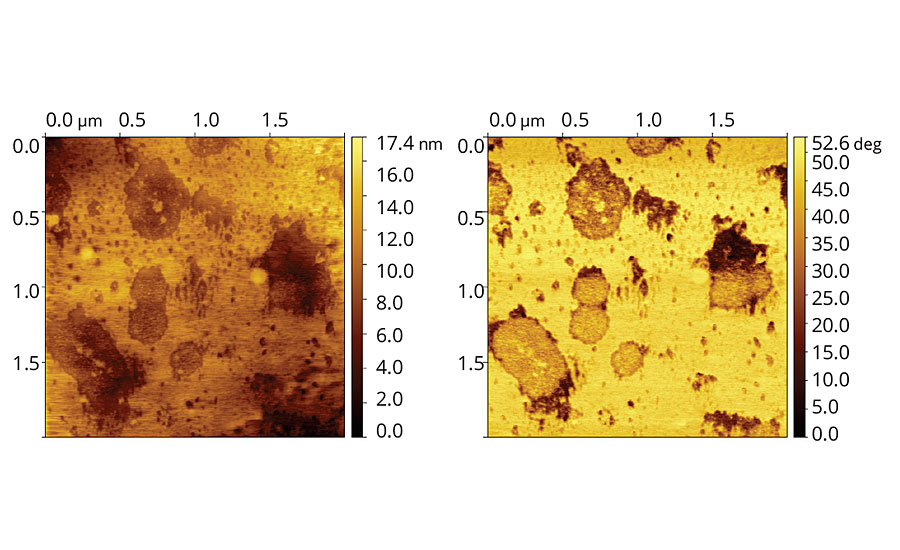
FIGURE 5 » Topographic (left) and phase (right) images of vinyl-acrylic latex film containing 8 php of Coalescent D.
Some representative AFM images of acrylic latex films containing 8 php of coalescents A-D are shown in Figures 6-9.
Figure 6 presents the topographic and phase images of films cast from acrylic latex with Coalescent A. The topographic images show that it is still possible to see particle domains, although the particles are very packed, and film roughness is low. The phase image also shows that it is possible to see particle limits, although domains with distinct phases are evenly distributed along the film.
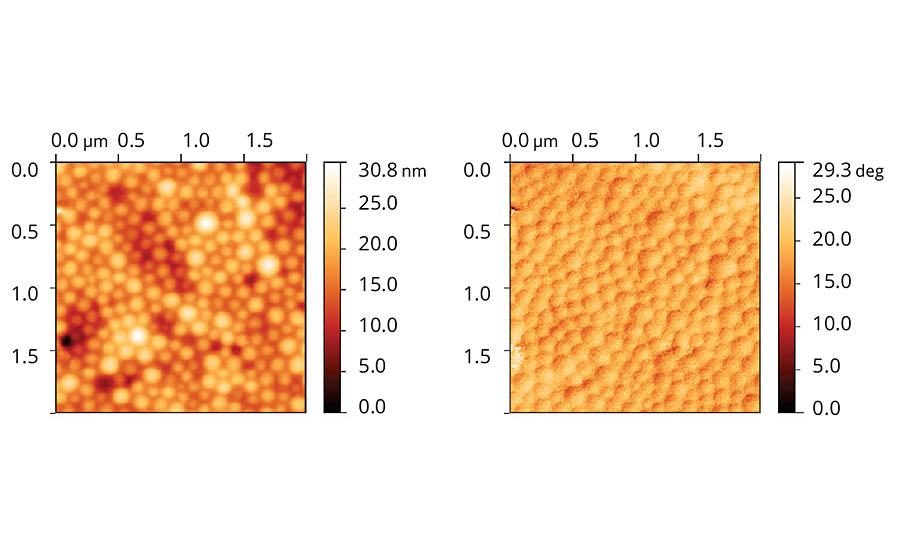
FIGURE 6 » Topographic (left) and phase (right) images of acrylic latex film containing Coalescent A.
Micrographs of films cast from acrylic latex containing Coalescent B are shown in Figure 7. In the topographic image the particles are flatter and film leveling is higher in comparison to films containing Coalescent A. The phase image also shows the particle limits and the distribution of species with different phases, or degree of plasticization, between the particles.
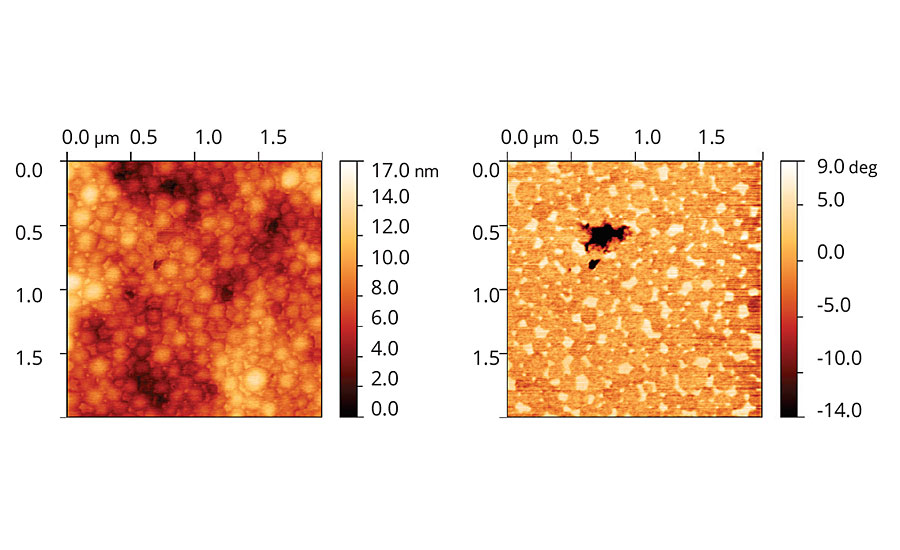
FIGURE 7 » Topographic and phase images of acrylic latex film containing Coalescent B.
In the case of film containing Coalescent C, Figure 8, the AFM image shows no presence of particle domains and flatter film in comparison to the ones presented in Figures 6 and 7. However, in the phase image we can see two predominant phases: one phase that seems to be particle cores reminiscent from coalescence immersed in another continuous phase.
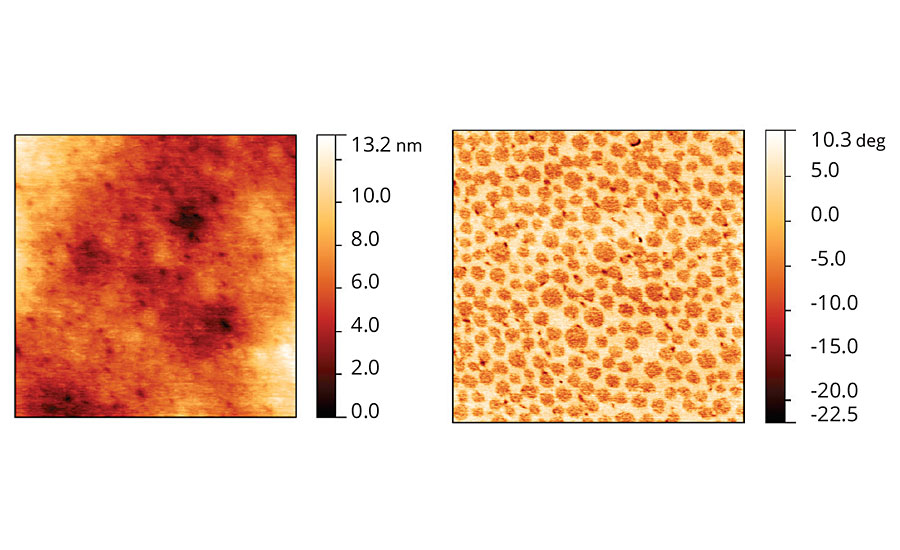
FIGURE 8 » Topographic and phase images of acrylic latex film containing Coalescent C.
Film containing coalescent D, presented in Figure 9, shows a topographic pattern similar to Coalescent B. However, the phase image of this film is much more homogeneous than the phase images of Coalescents B and C, and similar to the phase pattern presented by Coalescent A.
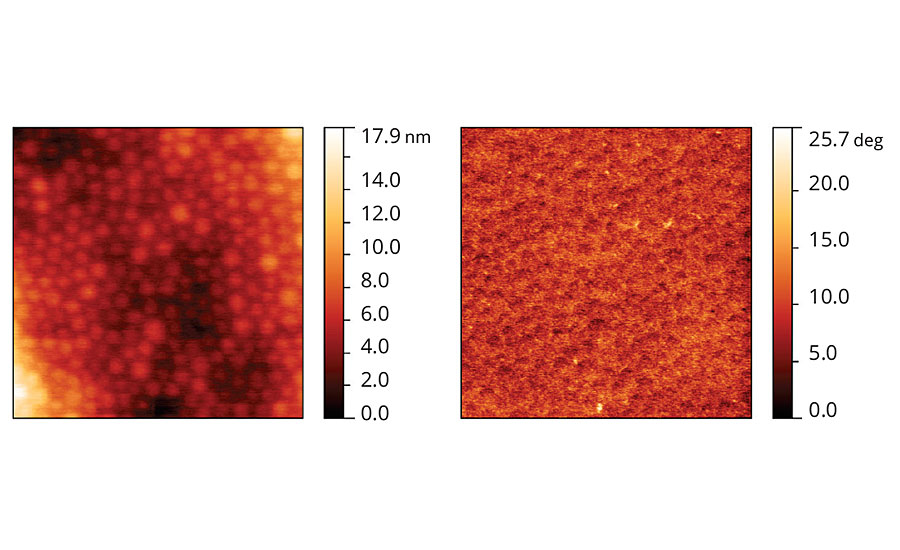
FIGURE 9 » Topographic and phase images of acrylic latex film containing Coalescent D.
Conclusions
The low-VOC coalescents investigated in this work presented high affinity for vinyl-acrylic and acrylic latexes and ability to decrease their MFFT. Topographic images suggest that the low-VOC coalescents generated more coalesced and leveled latex films than the standard high-VOC coalescent. Among the low-VOC coalescents, Coalescent C generated vinyl-acrylic and acrylic latex films with highest degree of coalescence. Phase images of acrylic latex containing Coalescent A and Coalescent D are more homogenous than the ones containing Coalescent B and Coalescent C, suggesting lower segregation of species with distinct viscoelasticities along the films.
Future Work
Further evaluations will be performed in order to correlate the features obtained from AFM micrographs with paint properties and apply this knowledge for developing paint formulations with optimum technical and environmental performance.
References
1 Gilbert, J.A. Recent Trends in the Architectural Coatings Industry, Proceeding of 43rd Annual International Waterborne Symposium 2016.c
2 Santos, J.P; Corpart, P.; Wong, K.; Galembeck, F. Heterogeneity in Styrene-Butadiene Latex Films, Langmuir 2004 20 (24), 10576- 10582.
3 Keddie, J.L.; Film Formation of Latex, Materials Science and Engineering R: Reports 1997, R21, 101.
4 Steward, P.A; Hearna, J.; Wilkinson, M.C.; An Overview of Polymer Latex Film Formation and Properties; Advances in Colloid and Interface Science 2000, 86, 195-267.
5 Lohmeijer, B.; Balk, R.; Baumstark, R. Journal Coating Technology Research 2012, 9(4), 399-409.
6 Hansen, C. M.; Hansen Solubility Parameters: A User’s Handbook: Taylor & Francis Group, 2007.
For more information, e-mail juliane.santos@oxiteno.com.
By Juliane Pereira Santos, Robson André Pagani, Carlos Roberto Tomassini, Rafael Caetano Jardim Pinto da Silva, and Silmar Balsamo Barrios, Oxiteno Indústria S.A. Brazil; and Kip Sharp, Oxiteno USA
Looking for a reprint of this article?
From high-res PDFs to custom plaques, order your copy today!