Is a New Pre-Dispersion Milling Process the Key
to Improved Efficiency in Color Suspensions?

For years, color coatings manufacturers have been using high-shear mixing techniques to try to wet pigments and reduce particle sizes in raw materials before wet milling. The theory is clear. The smaller the bead you can use in the final milling process, the less mass-specific energy is required to create a quality color dispersion. And that means less energy cost for production, and less time to create a quality product.
However, the optimum bead size in wet milling is dictated by the largest particle size in the raw materials. Even small fractions of oversized particles left in the raw material after a pre-milling process will result in inefficient wet milling.
The high-speed dispersing technique based on shearing through the solvent favored by the industry today leaves a long tail of coarse particles that compromise the milling process. A new pre-dispersion process has been developed that creates a homogenous slurry suitable for micro-bead milling, reliably bringing the promised cost savings and efficiency to an industrialized color suspension process for the first time.
What Fundamentals Are at Work Here?
The two fundamental aspects of bead milling are the intensity of a unit milling event and the number of bead-bead interactions.
The intensity of bead impact needs to be high enough to break a particle or agglomerate in the slurry. The more of these interactions, the faster the process and the higher quality the end product. While maybe not intuitive, the bead size presents the strongest lever to impact the number of beads and therefore bead-bead interactions in the process chamber.
Since the number of beads scales inversely with the diameter of the beads to the power of three, reducing the bead size by one order of magnitude results in three orders of magnitudes more beads in the same volume.
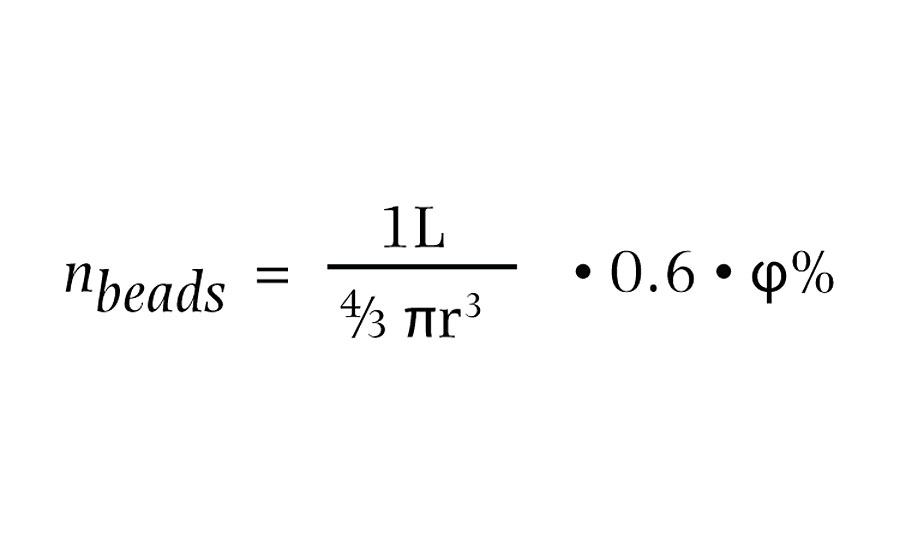
Even cutting the size of beads in half results in an eightfold increase in the number of beads, much more than would be possible changing other variables, such as the adjustment of bead loading (Figure 1).
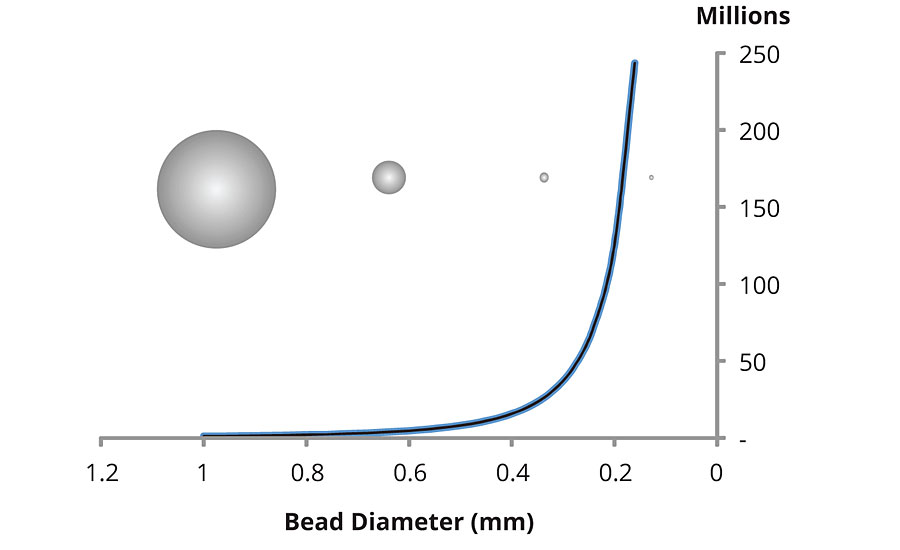
Since many beads introduce the milling energy more efficiently, using smaller beads results in a lower energy demand for a given milling task, which in turn can be accomplished in shorter time, with less energy or in a smaller mill.
What Determines the Optimum Size of a Bead for Wet Milling?
Why not use the smallest bead on every occasion? The limiting factor is that beads need to be large enough to break the largest particle in the slurry. In a starting slurry, pigment particle agglomerates of various sizes are present (Figure 2) and form a size distribution. Even though there’s typically only a small amount of large agglomerates, the milling beads need to be able to break these as well. The smallest beads suitable for milling a color dispersion are therefore usually oversized for the vast majority of pigment particles.
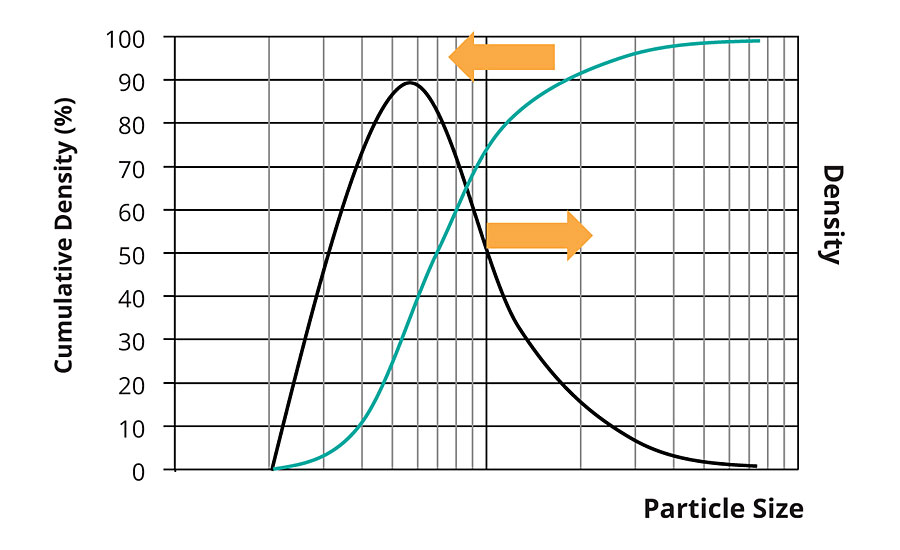
Why Does Bead Size Matter?
There are three essential drawbacks with being forced to use bigger bead sizes in wet milling. Firstly, as discussed above, the bigger the bead size, the more mass-specific energy is required in the process. When coatings manufacturers are forced to use larger bead sizes to cope with a tiny fraction of coarser particles (as most in the industry are), they are wasting energy. That’s a high price to pay for inadequate pre-processing.
Secondly, this process of using larger beads to reduce the coarser materials takes longer. In fact, grinding the final coarser materials to make a homogenous product takes disproportionately longer than grinding the majority of the slurry. The manufacturer must choose the optimum balance between increased cycle times, compromised product quality or an added filtering process, all of which will add time and cost.
Finally, the quality of the end product may be compromised because the smallest beads result in the finest grinding. Industrial quality related to particle size distribution include color – strength, gloss and transparency; rheology/viscosity; and stability/shelf life. Using smaller beads gives producers better control over all of these variables.
Why Does Traditional Pre-Milling Leave Over-Sized Particles?
Traditionally, coatings manufacturers use high-speed dispersers and other equipment based on applying shear through the liquid medium of water, solvent or some kind of formulation. This methodology is capable of breaking down loose agglomerates in a starting slurry, but the mechanism doesn’t provide enough force to break down more strongly aggregated or even unit particles that are part of the coarse tail of the distribution.
Typically, it is the last 10 to 15% of the particle size distribution that is the hardest to breakdown, and shearing simply isn’t able to apply enough force in most cases. Given that even a small percentage of larger particles left in the slurry will compromise the wet milling process, this is a significant issue. For example, where high-quality coatings are required in an automotive application, even 0.1% of larger particles is enough to ruin the finish.
How Can Advanced Pre-Dispersing Make A Difference?
Bühler’s new MacroMedia advanced pre-dispersing methodology uses bead impacts, which are much more efficient in breaking down particles, including hard aggregates and even crystals (Figure 3).
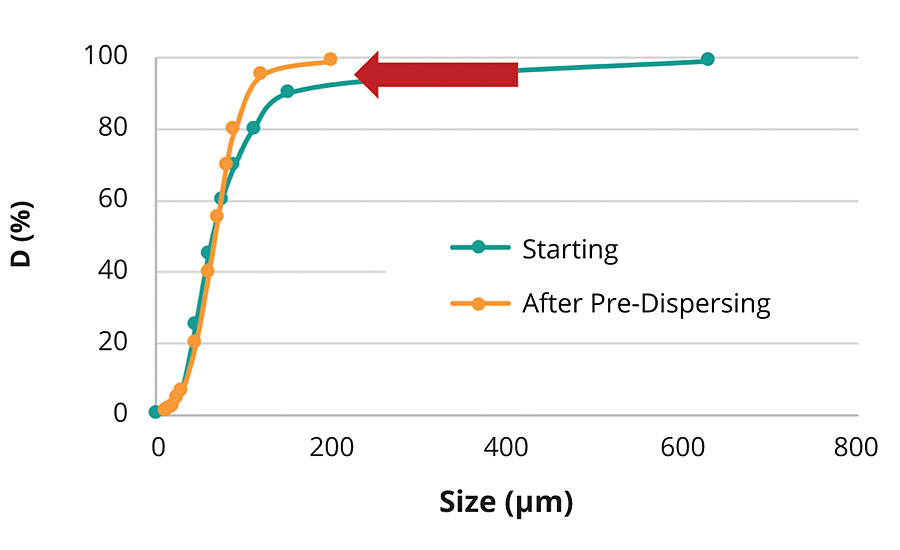
The other important variable is flow rate, which dictates the number of times the slurry enters the process zone anew in a recirculation process. A high turnover number creates the homogeneity required.
Bühler’s solution is a self-pumping device featuring a high-power density process chamber with pin counter-pin configuration and a parallel gap arrangement of 10 gaps, each 1 mm wide. This arrangement provides a hard-to-block bead separator without the flow limitations created by gap separators in usual bead milling equipment.
Typical flow rates of 10-20,000 L/h (45-90 gpm) depending on the viscosity of the product are available at low pressure, resulting in a large turnover number during recirculation processing. The high flow rate ensures that all particles are exposed to the process chamber for nearly the same (residence) time. The chamber is loaded with 3 mm beads, which provide a particular efficient size reduction of particles in the range of 1 mm-100 µm.
How Does It Work In Practice?
Using its global labs, Bühler has trialed MacroMedia with customer processes to establish the benefits of this advanced pre-dispersing methodology with different aggregates.
Proof of Concept with Limestone
In a first example, the lab considered the processing of limestone because it is a model for any product with a hard, coarse tail. Using a limestone fraction with a d 50 of 450 µm and a large tail with d 90 of 900 um and a d 100 at 1.2 mm, the MacroMedia device was loaded with the typical configuration of 60% (50-70%) 3.0 mm Y-stabilized ZrO 2 beads. Water with 0.1% guar gum was circulated and limestone was added to form a 39 wt% slurry. The maximum rotor speed of 10.2 m/s was chosen and the device drew 10-11 kW at a flow rate of 22,000 L/h (97 gpm). Within 10 kWh/t introduced energy the d 90 was reduced from 900 µm to below 100 µm. For a 1,000 lb (450 kg) batch, this would be accomplished in just 25 min. This particle size is small enough so it could be subsequently fine milled with 0.3 mm media without issues (Figure 4).
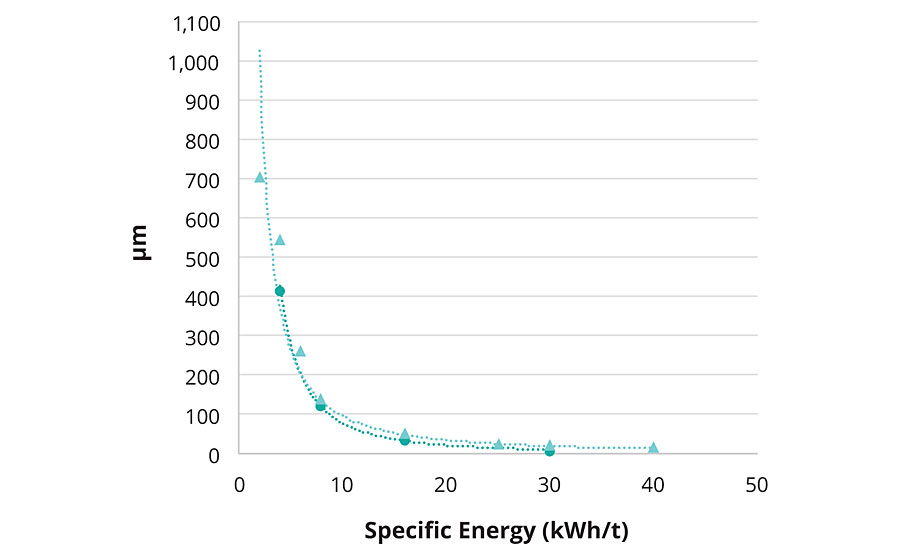
65% Increase in Productivity for Violet Printing Ink
In a second example, the lab optimized the dispersing process of a violet pigment in a NC-based printing ink. The starting particle distribution features a fineness of grind of 500 µm while the target size was at 3 µm, so the required size reduction spans more than two orders of magnitude.
The established process involved pre-mixing using a high-speed dissolver and subsequent fine-grinding with 1.0-1.2 mm beads to quality. Milling was carried out with a net productivity rate of approximately 100 kg/h (220 lb/h).
The new advanced production process was considerably faster. Using the advanced pre-dispersing concept for preparing the slurry meant that smaller, 0.7-0.9 mm beads could be employed in fine grinding, which was carried out using a high-performance mill and in re-circulation. All quality criteria were reached at a net productivity rate of 165 kg/h (364 lb/h), an increase of 65% productivity.
When taking the additional time required for the advanced pre-dispersing into account, the process is still more efficient: The MacroMedia was used to introduce 20 kWh/t prior to fine grinding, which was accomplished at a production rate of 500-600 kg/h. The productivity for the MacroMedia plus fine-grinding process totaled at 128 kg/h (283 lb/h), still a net productivity increase of 28% over the original grinding step, not even counting the time previously required on the dissolver.
128% Higher Productivity for Black Varnish
In this example, the established production process involved a pre-grinding step with larger media before fine grinding with smaller media after pre-mixing with a high-speed dissolver: (1) a batch of black varnish was pre-ground using 1.2-1.4 mm beads in a pass operation with a throughput of 277 kg/h (610 lb/h) and approx. 70 kWh/t; (2) this pre-milled slurry was then fine-ground; (3) using smaller 0.8 mm media in recirculation to a fineness of grind of <7 µm, which required 140 kWh/t and was accomplished at a rate of 110 kg/h (140 lb/h). The overall grinding net productivity including pre- and fine-grinding amounts to 70 kg/h (157 lb/h).
The new process makes one of these grinding steps entirely obsolete: Introducing 40 kWh/t in the advanced pre-dispersion process instead of using a high-speed disperser enables the use of 0.5 mm beads in just one fine grinding step, which was now carried out in one single pass operation directly to quality (Table 1). The throughput of the fine grinding was with 300 kg/h (660 lb/h) – in the same order as the previous pre-grinding step. Because no additional grinding step was required, the combined process has a much higher productivity of 160 kg/h (350 lb/h), an increase of 128% over the established process. The shorter time is ultimately due to the much reduced total energy of 110 kWh/t compared to 235 kWh/t.
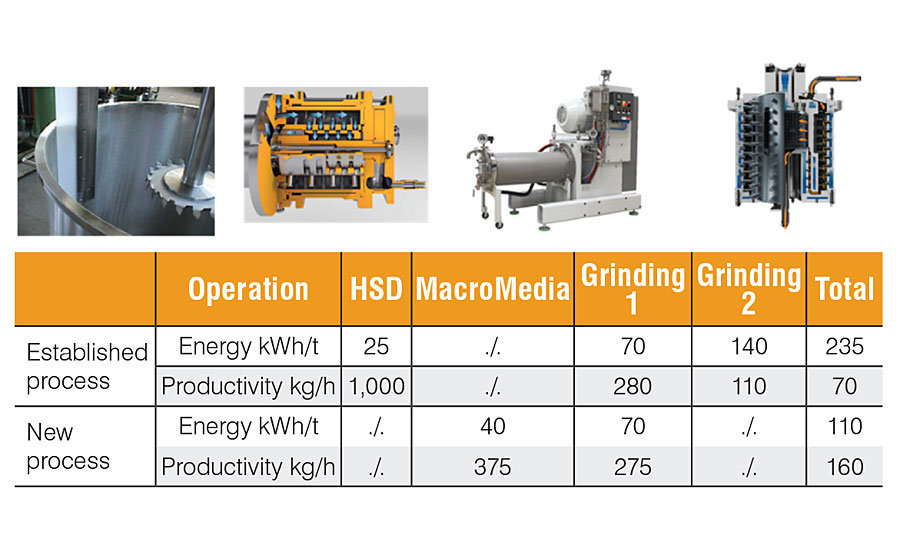
This example shows several characteristics of the new process:
- The advanced pre-dispersion process enables the use of smaller beads in fine grinding, resulting in a much more efficient fine-grinding operation.
- Unlike a bead mill, this equipment has been specifically designed for a pre-dispersion process. The larger bead size targets the tail-end of a particle size distribution over-proportionally, effectively tailoring the slurry distribution for optimized bead milling.
- The subsequent fine-grinding step is not limited to the use of a high-performance mill; rather, as in this example, pass operation on a full volume disc mill does also benefit greatly from using smaller beads after advanced pre-dispersion.
75% Increased Productivity for Sub‑Micron Grinding
In a final example, the lab evaluated the new process in sub-micron grinding. In order to satisfy the requirements for an ink-jet application, the target particle size d 90 was <140 nm, which requires the use of small media of max. 0.3 mm and high-efficiency grinding equipment in order to reach the target quality with a useful productivity rate.
The lab strived to use 0.1 mm media in a high-performance bead mill operated in re-circulation for this application, which required installation of a 0.05 mm (50 µm) gap size screen as a bead separator. As a consequence, the pre-dispersion process had to deliver a particle size distribution with a d100 <50 µm.
This goal was achieved by introducing 100 kWh/t at a net productivity rate of 100 kg/h, as shown by laser diffraction and also a wet-sieve test, where no residue was found when passing the slurry through a 50 µm filter bag.
This was confirmed by the subsequent fine grinding, which was carried out with 0.1 mm media in re-circulation without issues. Fine-grinding required an additional 800 kWh/t to reach the final target of d 90 <140 nm, which for a 15-liter mill equates to a productivity of approx. 35 kg/h. Another sample of the pre-dispersed slurry was milled with 0.2 mm media under similar conditions. Due to the larger media, this process required 1200 kWh/t at a rate of 20 kg/h, making the fine-grinding process using 0.1 mm media 33% more energy efficient and 75% more productive.
Creating a Compact Mini-Plant Ready for Deployment
Bühler has developed a general production concept based on this combination of advanced pre-dispersion and fine grinding with smaller media (Figure 5). This pre-engineered mini-plant is a versatile tool for many wet-grinding applications and consists of a batching tank, a milling tank and a let-down tank connected with MacroMedia and fine-grinding mill. The complete concept is realized in a compact standard layout requiring a footprint of 7.2 x 7.0 m (24 x 23 ft) and a height of 5.5 m (18 ft).
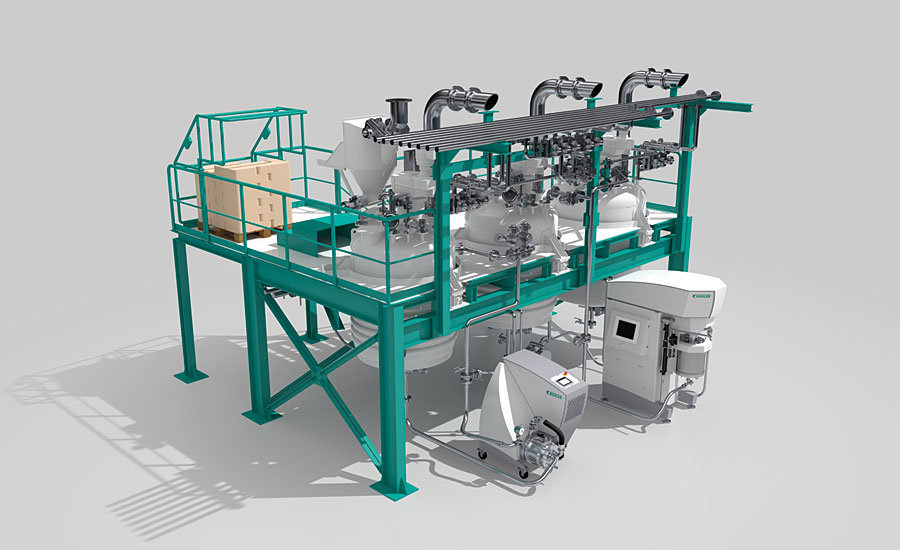
After pre-dispersing, the batch is transported in a transfer pass into the milling tank from which the fine-grinding is started, either in re-circulation or in pass operation. The product is collected and finalized in the let-down tank.
Time for Coatings Manufacturers to Embrace Advanced Technology?
Patrik Maeder, Director of Sales Grinding and Dispersing in Plymouth, MN, says that these trials suggest that this new advanced pre-dispersal technology could help most manufacturers in color dispersal and wet milling to improve quality and save money. He says, “The headline benefit is undoubtedly being able to use less energy to create a higher productivity process. That should be enough in itself. But MacroMedia advanced pre-dispersal also gives customers greater control. This makes achieving homogenous, high-quality end products with just the right color, consistency and longevity – and less waste – much easier. That’s an exciting prospect for the industry.”
Looking for a reprint of this article?
From high-res PDFs to custom plaques, order your copy today!