Impact of Silanes on the Solution Stability of Latex and Ultimate Paint Film Properties

Silanes have been shown to improve film properties of resin systems when they are reacted into the resin or incorporated into a resin solution as additives. The improved properties result from their unique functionality, which allows for inter-polymer crosslinking and chemical bonding to many substrates. Due to the high reactivity of silanes, especially towards hydrolysis and self-condensation, their use as additives in water-based systems can be limited. Therefore, it would be desirable to successfully disperse the silane additive into water-based resin systems and have that system remain stable. Work was done to investigate the structure, functionality and processing conditions to successfully disperse silanes with different functionality and molecular weight in latex resin. Application work was done to test the impact of these silane additives in clear resins and paint formulations. This application work has shown advantages of the dispersed silanes versus standard latex in fully formulated systems. The authors reason that the property enhancements afforded by this new technology should be applicable to DTM, architectural and industrial maintenance coatings.
Alkoxysilane Structure and Chemistry Overview
Structure and Chemistry Impact on Crosslinking
The chemistry and reaction of alkoxysilanes have been well studied. 1 Depending on the alkoxysilane type and reaction conditions, the alkoxysilane reaction could be difficult to control. However, under optimum conditions, alkoxysilanes can be a valuable option to enhance properties of the systems they are used within.
The use of alkoxysilanes in solventborne systems has been in place for some time. They have been reacted into solvent-based resins or blended into solvent-based resin with relative ease. However, use in water-based systems requires more consideration. 2 As shown in Figure 1, alkoxysilanes will react with water to generate the silanol. Depending on the reaction conditions, the hydrolyzed silanol can quickly crosslink with itself to form a silane network. Conditions such as pH, temperature, presence of catalyst, amount of functionality within the silane and other functional groups can all impact the stability of the hydrolyzed silane.
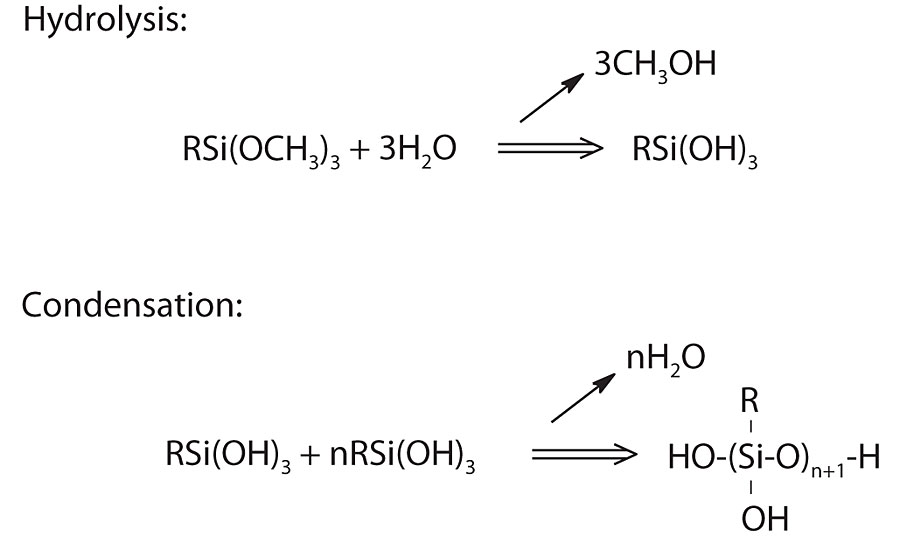
Structure and Chemistry Interaction with Substrate
Once the silanol is formed it can readily react with itself, with a functional resin or with a substrate. Each of these possible reactions may need different conditions for it to proceed. A catalyst is typically all that is needed for the self-condensation of the silanol. Catalyst and/or heat is often used for reaction with other resins and substrates. Figure 2 shows condensation onto a substrate. A substrate with existing hydroxyl groups is needed for this reaction. The first step involves a hydrogen bonding coordination, then heat is used to drive the coupling.
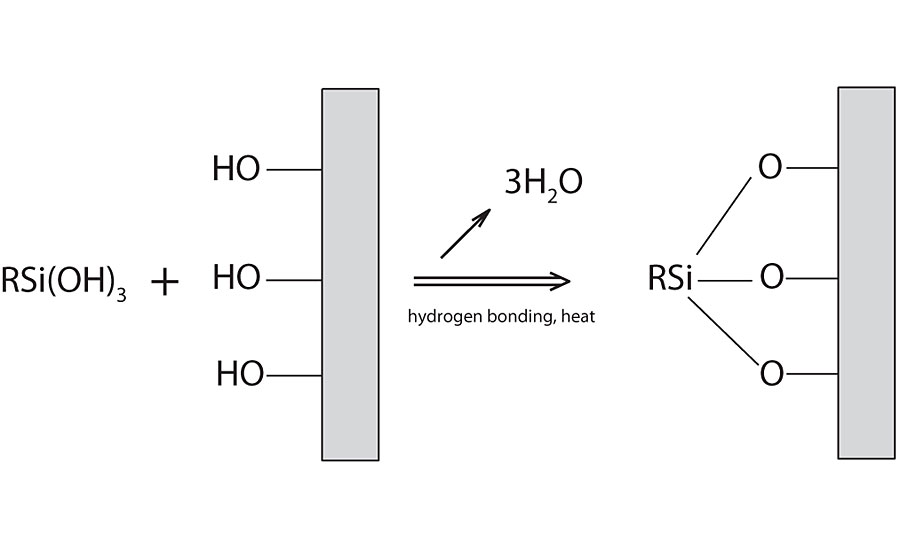
The condensation step is the critical step that provides (1) the bonding/adhesion to the substrate, and (2) the intermolecular crosslinking within the film for improved film properties.
Dispersion in Water
Much industry work continues in the area of creating stable dispersions of alkoxy silanes in aqueous solutions. Alkoxy silane monomers with functional side groups have been successfully converted to the stable silanol and used in water-based systems. 3 Work has also been done with hydrophilic components on the silane monomer types. Our work is focusing on higher-molecular-weight, higher-branched alkoxy silanes, adding more silanol functionality. The challenge with higher-molecular-weight, higher-functional silanols is not only the aqueous stability, but also the stability when used in water-based resins and/or the fully formulated paints and inks.
As previous work has shown, the success of the alkoxy silane dispersion in water is enhanced by conversion to the corresponding silanol. This has been done in various pH conditions, but typically in the 4-7 pH range. The use of the proper surfactant is also beneficial in the dispersion and stability of the aqueous solution. Even with these controlled conditions, there are limits to the stability of a higher molecular weight with higher functionality. Thus, we have seen not all silanes will be successfully dispersed in water.
In our work we looked at two different levels of molecular weight and alkoxy silane functionality. Compound 1 was a lower-molecular-weight, highly-branched, highly-functional alkoxy silane. Compound 2 was a higher-molecular-weight, lower-branched, lower-functional alkoxy silane. See Figure 3 on the previous page for general formulas. Both compounds were successfully dispersed in water by adjusting the pH into range, using surfactant and applying the necessary mixing. These stable aqueous dispersions were used to test in a latex resin.
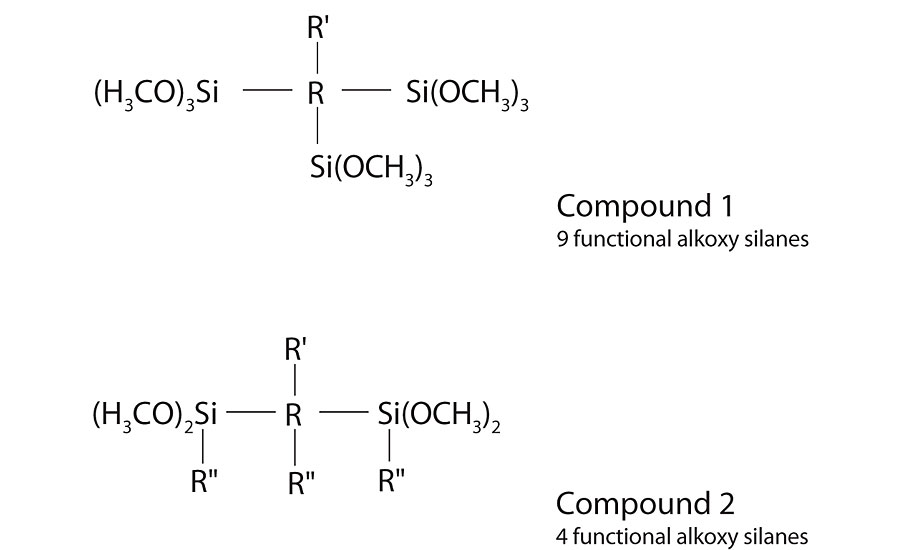
Processing Conditions Impact on Dispersions into Latex
Various processing conditions were tested to optimize the incorporation of the stable aqueous dispersion into the latex. The three conditions we studied were temperature, mixing speed and addition time of the silanol solution.
The first set of conditions tested was meant to simulate addition to a latex during production. The temperature was 40 °C, mixing was 150 rpm (with standard paddle blades) and the silanol was added over ~30 min. This represents a low-shear mixing process. While trying to incorporate Compound 1 into the latex under these conditions, we noticed a build-up of resin onto some of the glassware. After the addition was complete and held for two hours, the material was poured out through a paint filter. Figure 4 shows the solids that developed. It was thought that a lower-molecular-weight species would have easily dispersed into the resin. It is believed the high level of functional groups reacted into an insoluble species. Compound 2 was easily incorporated into the latex under these conditions. There was no residue in the flask or in the paint filter.
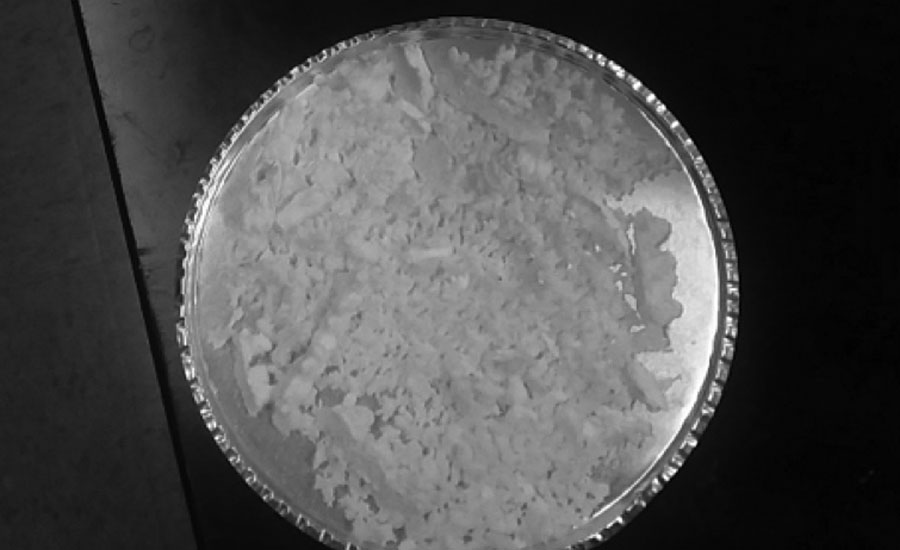
The second set of conditions were meant to simulate addition during paint or ink formulation. The temperature was ~25 °C, mixing was 1,000-2,000 rpm (Cowles blades) and the silanol was added quickly to the vortex. This represents a high-shear mixing process. Both compounds appeared to mix in well, and no significant particle development was observed in the formulation vessel or paint filter. However, upon sitting, Compound 1 developed a fine particle that led to a hard settling, while Compound 2 stayed in solution.
Overall, under two standard application processes, Compound 2 was easily incorporated into the latex and has maintained its stability over time.
Latex Resin Considerations
Two types of latex resin were used in this work. One product was a styrene acrylic latex with a low Tg and a pH less than 7. The second product was a 100% acrylic latex with moderate Tg and a pH greater than 7 (Table 1). Compound 1 was incompatible with the low-pH latex and partially compatible with higher pH latex. Compound 2 was compatible with both latex products. The more acidic environment of the styrene acrylic latex is believed to have contributed to the increased instability of the highly functional silanol. Therefore, all the clear film testing and paint film testing focused on the Resin 2 binder.
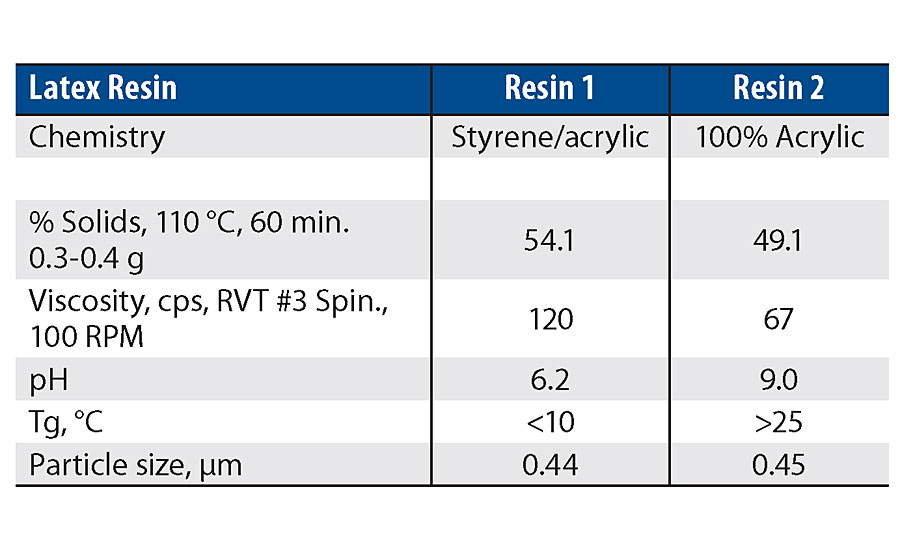
Latex Testing, Clear Films
Physical Solution Properties
Work on our clear films and paint films used the 100% acrylic resin with the silanol pre-blended into the latex. A ladder study was performed to test the effects of the silanol at different levels. Table 2 shows the wet property results of these blends. There is not a significant change in wet properties with the increase in silanol.
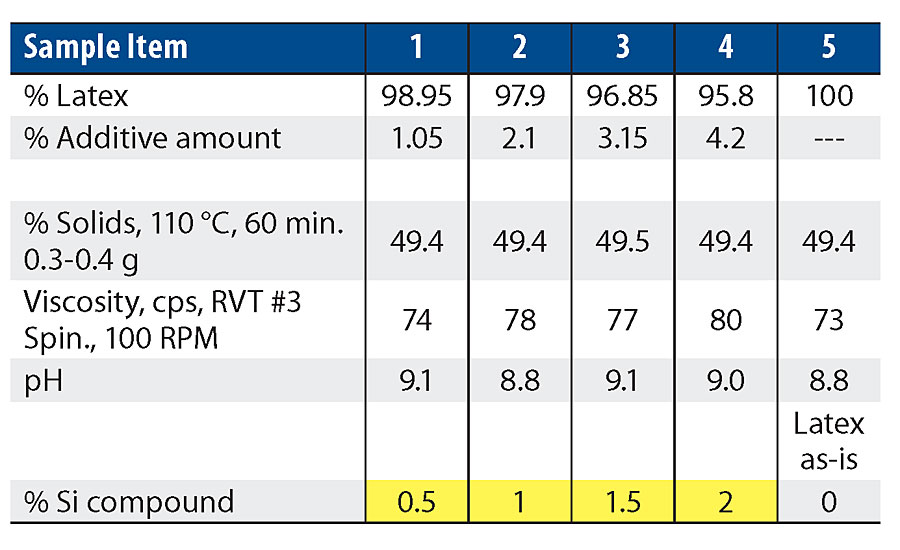
Clear Film Results
All the samples from Tab. 2 were applied to Leneta charts, aluminum panels and cold rolled steel (CRS) panels at a wet film thickness of 6 mils. Physical data was taken after 7 days in a controlled environment room. The following figures highlight the significant findings from this work. The 0% data point is the latex without additives, the control.
Figure 5 shows the 20° and 60° gloss results. As more silanol is added, the gloss values are trending down. The 2% level shows the most significant drop from the control.
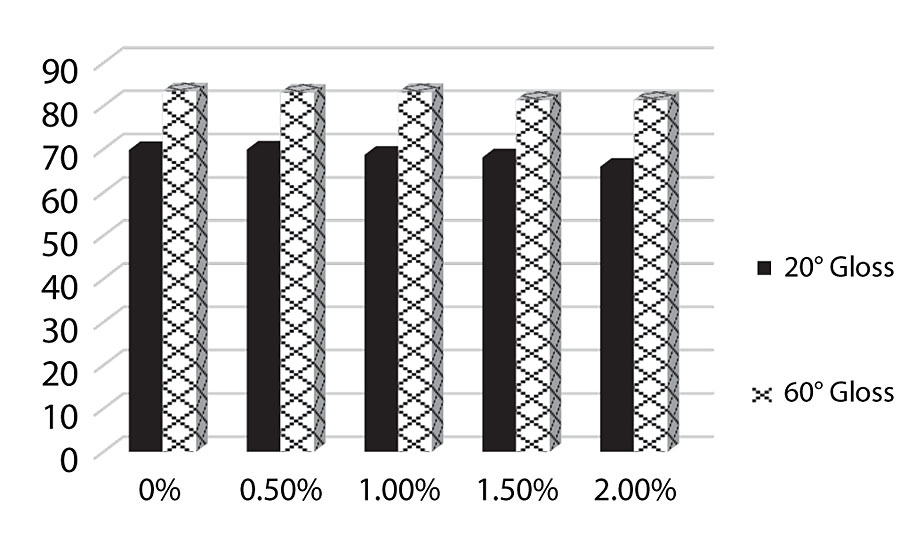
Figure 6 shows an increase in block resistance as 1% of the additive is approached. After 1% we see the block resistance reverting back towards the control. The silane should improve block resistance, which we see up to 1% silane added. However, after 1% it appears the film is softening.
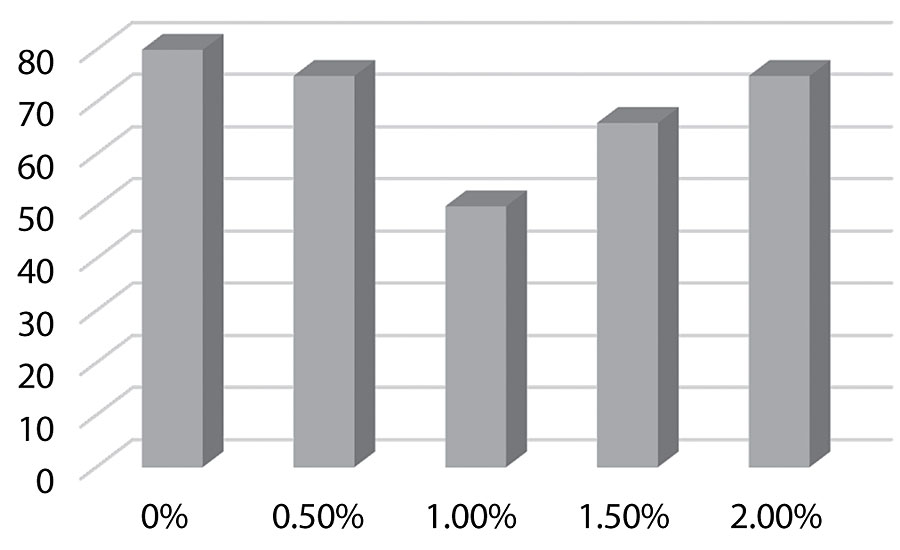
Figure 7 on the following page shows the surface energy dropping as more silane is added as would be expected. This could predict improved dirt pick-up resistance.
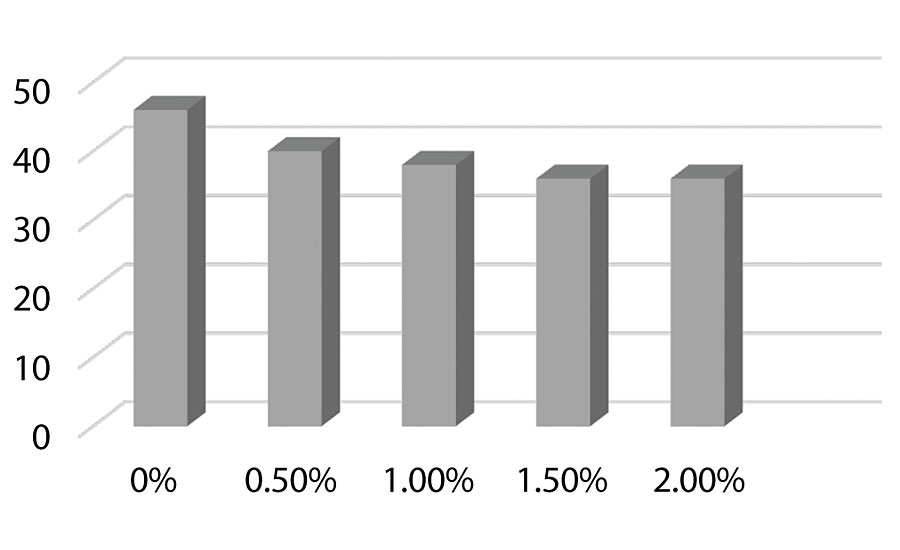
Figure 8, Figure 9, and Figure 10 show hardness testing. Both pencil hardness and Koenig hardness were used to monitor this property. We see improved pencil hardness on aluminum at lower levels of silanol. On cold rolled steel, we see equal hardness to the control until the higher levels of silanol. The Koenig hardness is similar to the CRS results. Similar hardness is observed to the control until the higher levels of silanol are reached.
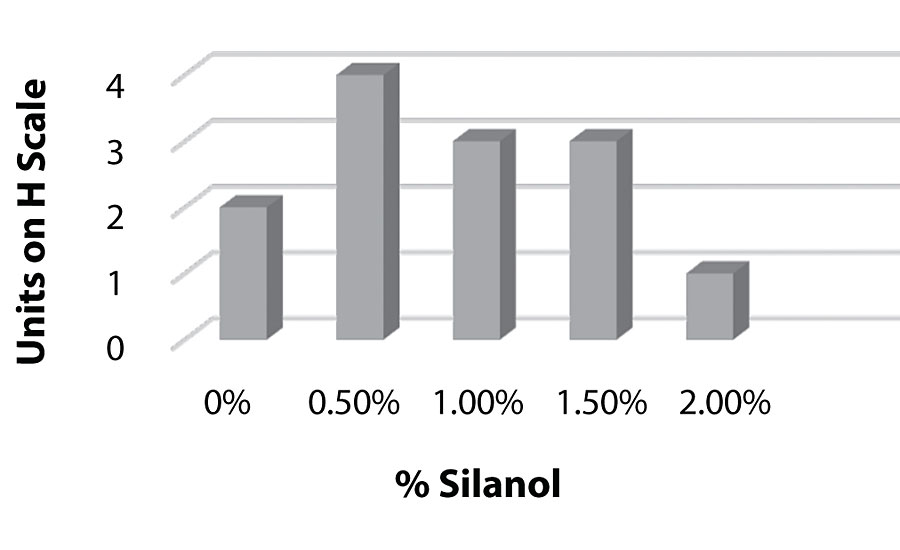
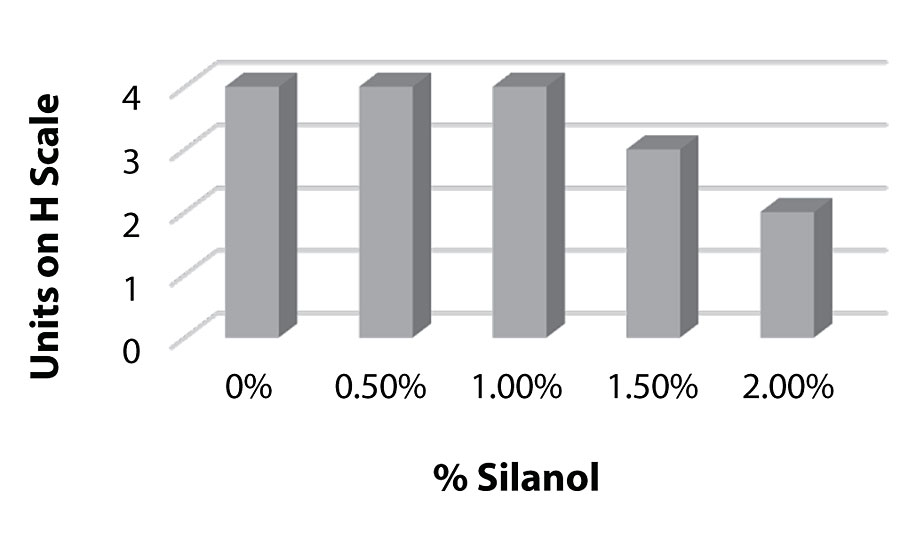
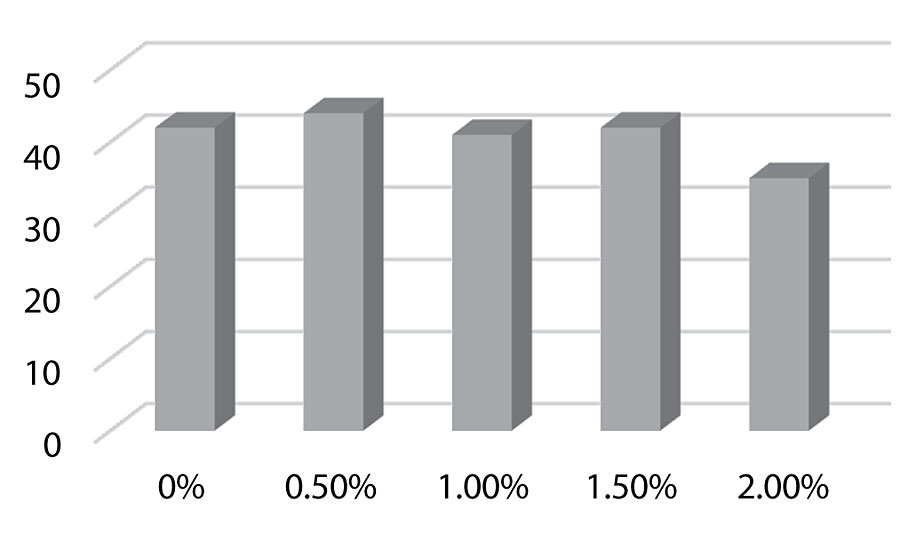
Figure 11 and Figure 12 show the effect of the silanes on adhesion. We do see a drop of adhesion at higher levels of silane on aluminum. However, there is no drop-off in adhesion on steel.
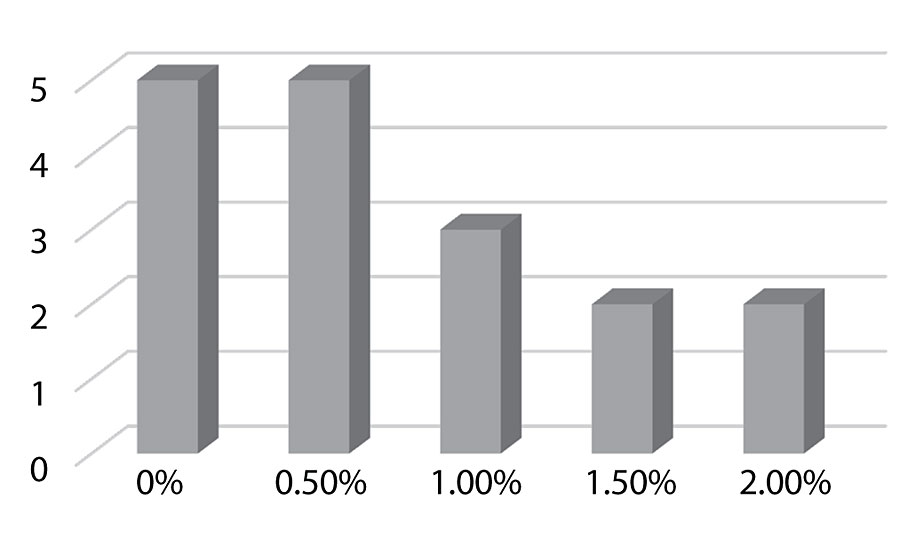
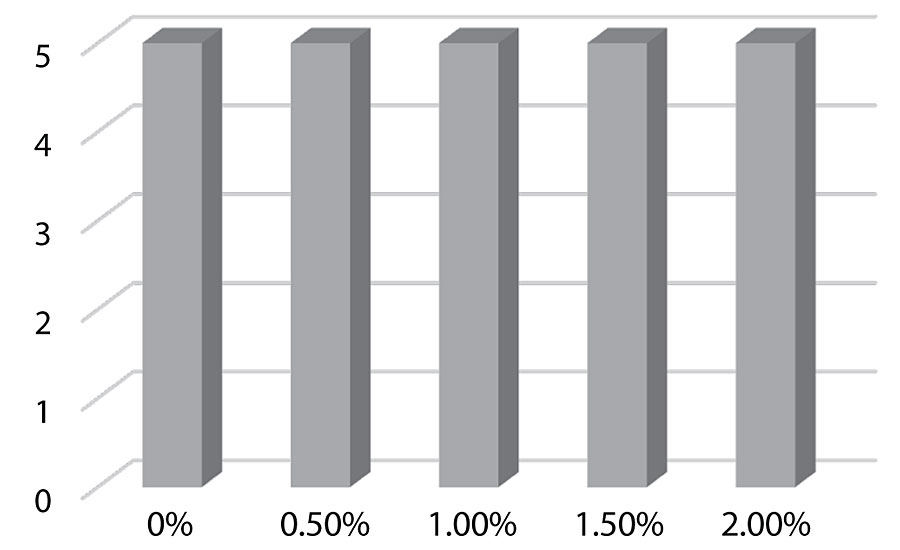
Impact on Paint
Physical Paint Properties
A high-quality custom white semigloss paint was formulated and used as a base for each concentration of additive. The paint formulation used is listed Table 3. Note, the 1.5% level in the latex was not used in the paint testing. The wet properties for these formulations are listed in Table 4.
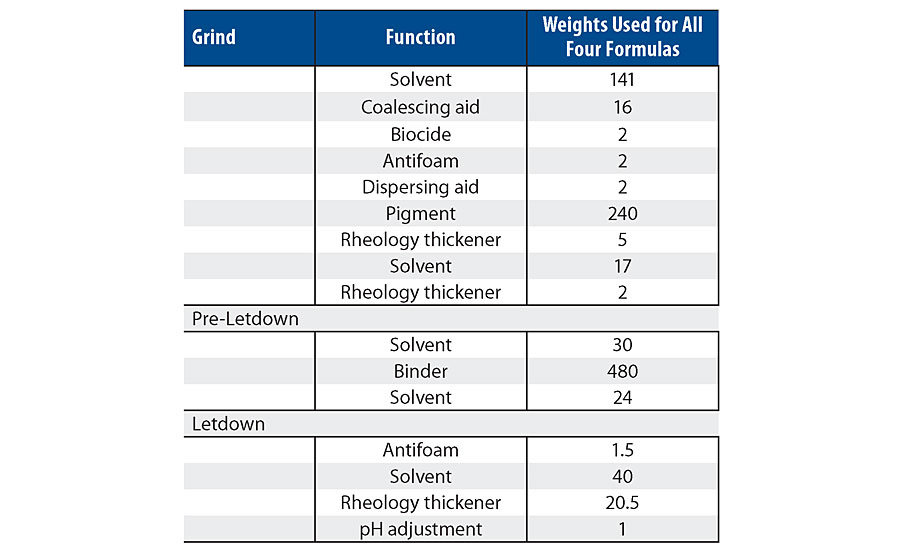
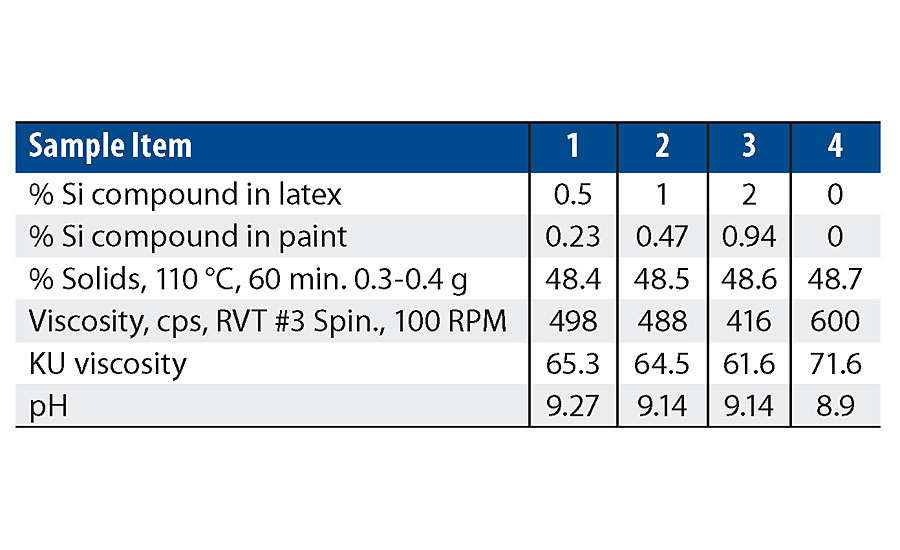
As can be seen in the RVT and KU viscosity data in Tab. 4, there is a viscosity drop as more of the silanol additive is used. Figure 13 shows the viscosity trend via Brookfield viscosity. The additive may be acting as a dispersing aid in the paint formula since there was no significant viscosity trend in the latex. More work is needed to understand this trend.
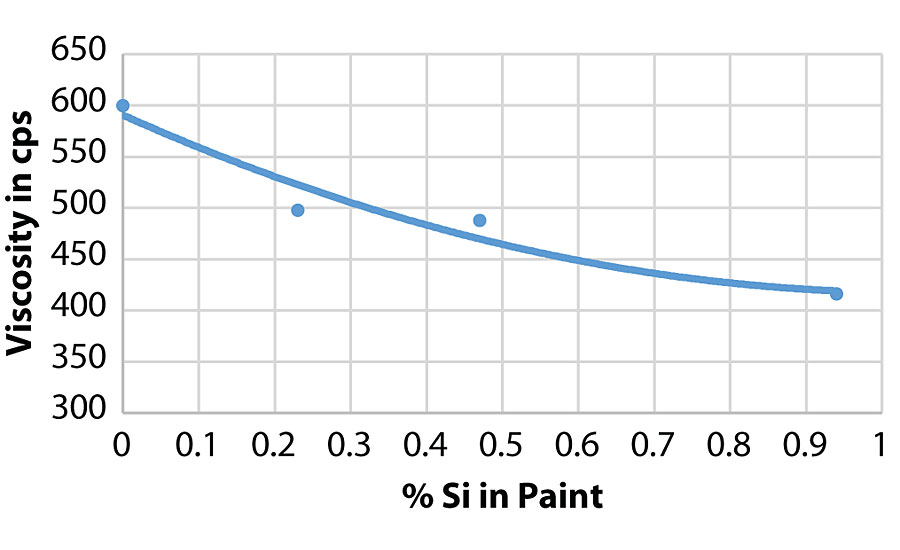
Paint Film Results
All the samples from Tab. 3 were applied to Leneta charts, aluminum panels and CRS panels at a wet film thickness of 6 mils. The data was collected after 7 days in a controlled environment room. The following figures highlight the significant findings from this work.
Figure 14 shows the 20° and 60° gloss results. As more silanol is added, we see the gloss levels drop slightly but it does not appear significant at these levels.
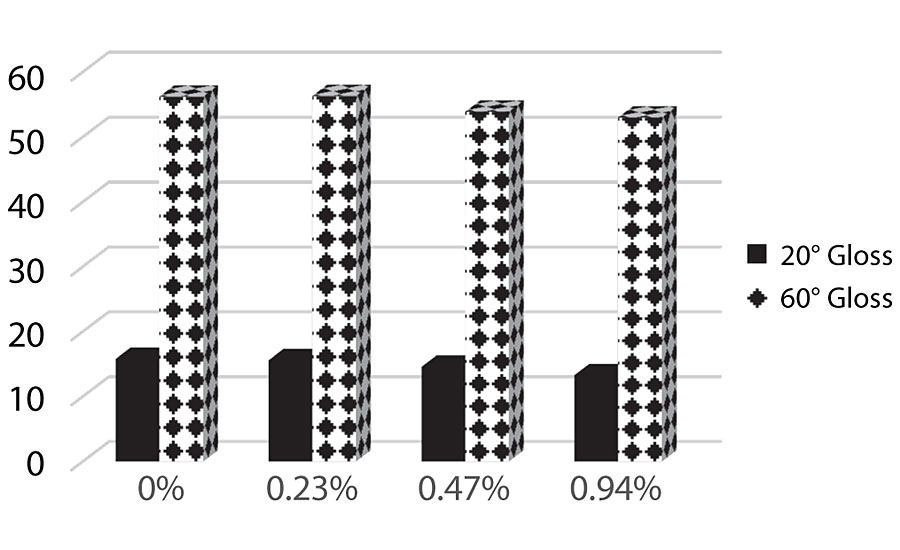
Figure 15 shows an increase in block resistance even at 0.23%. The improvement in block resistance continued with each increase in silanol versus the control. At the highest level studied there was no film removal, as seen in Figure 16. These results are based on one test run. Work is planned to test the results in other colors.
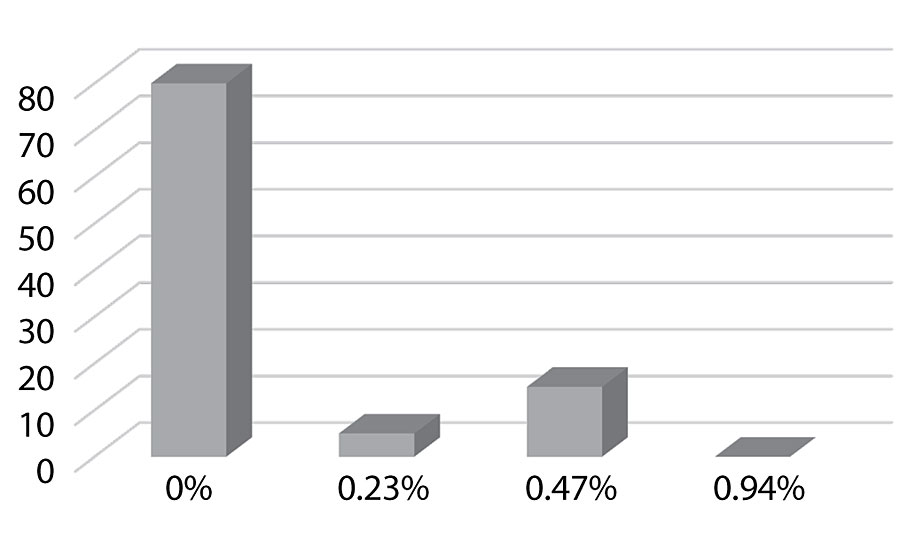
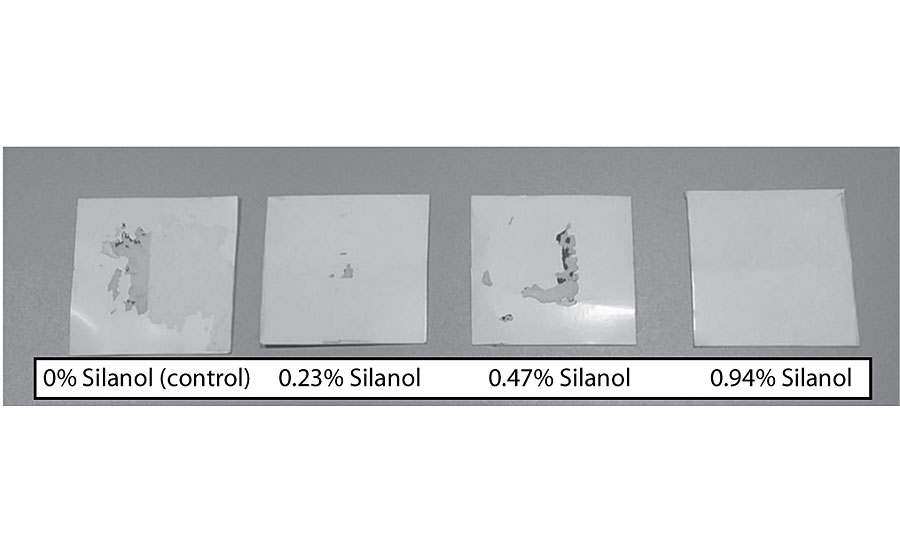
Figure 17 shows the surface energy dropping as more silane is added as would be expected. This could predict improved dirt pick-up resistance in the paint.
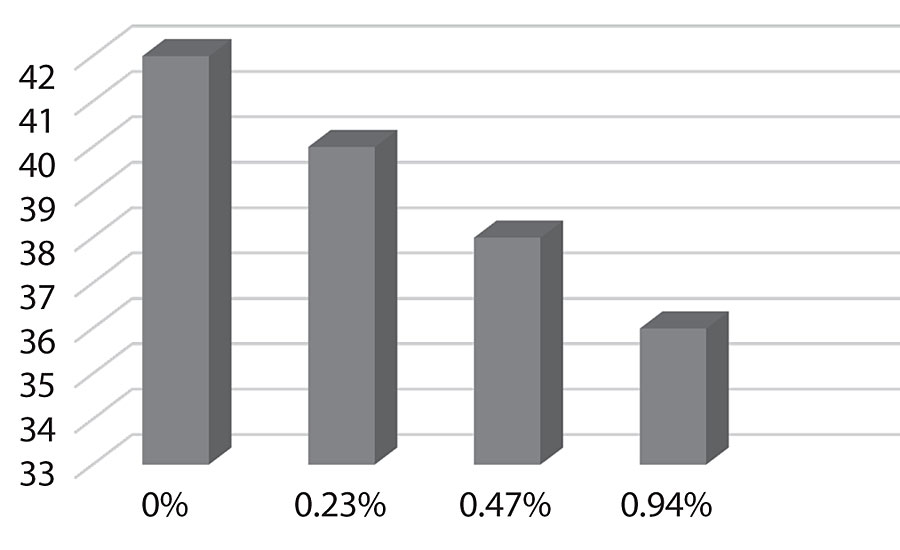
Figure 18, Figure 19, and Figure 20 show hardness testing on paint. Both pencil hardness and Koenig hardness were used to monitor this property. We see similar pencil hardness on aluminum compared to the control, up to 0.94%, and then we see the hardness drop. On cold rolled steel, we see similar hardness to the control at all levels. The Koenig hardness shows an increase trend up to the 0.94% level, then a drop in hardness is observed. This work suggests a softening effect on the film at higher levels.
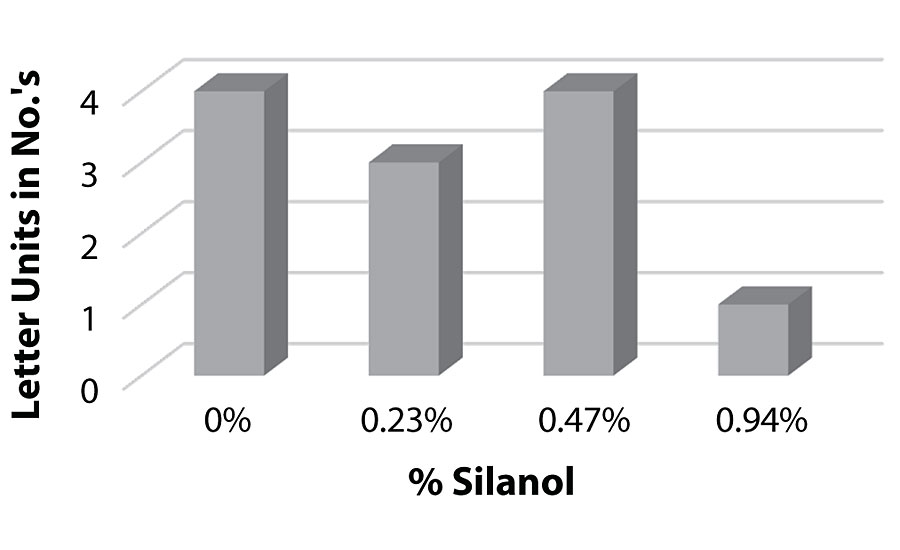
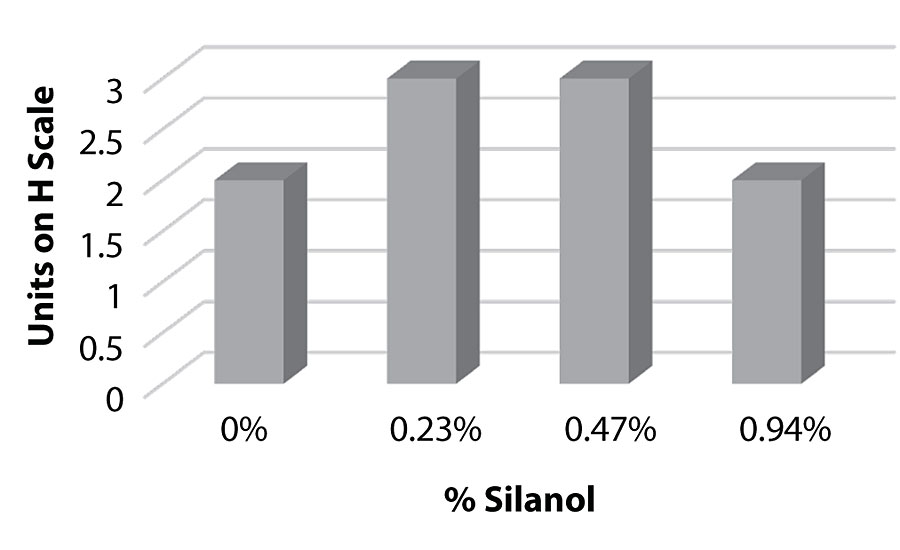
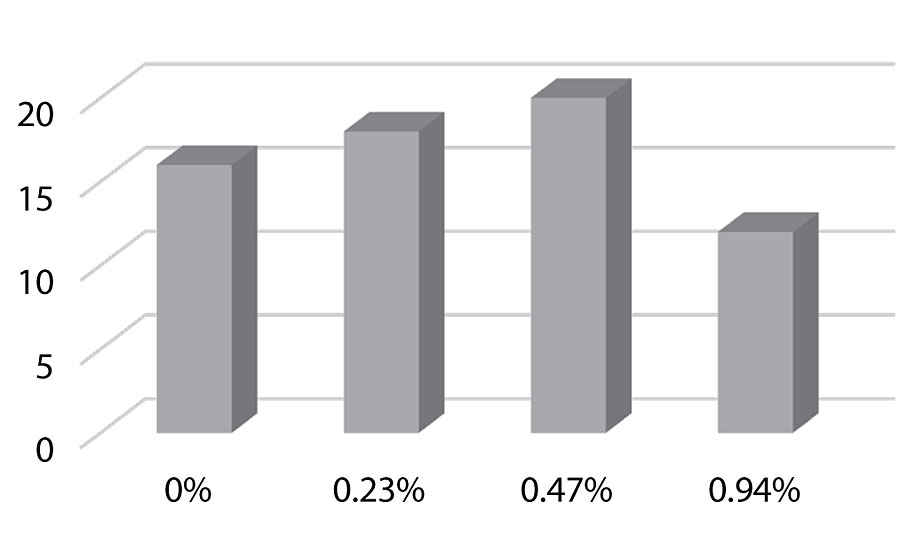
Figure 21 and Figure 22 show the effect of the silanes on adhesion. We do not see a drop of adhesion at higher levels of silane in the paint over aluminum or CRS. The crosshatch adhesion images in Figure 23 show the test results on aluminum
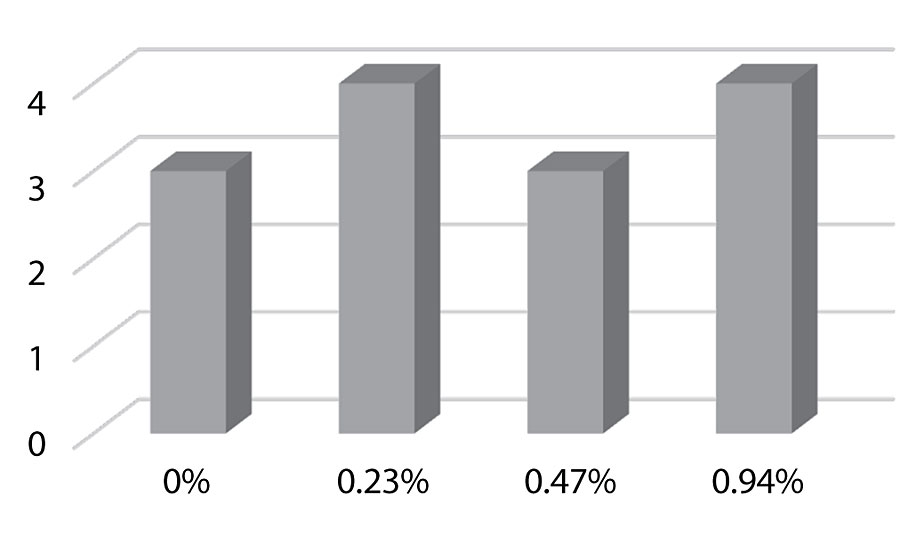
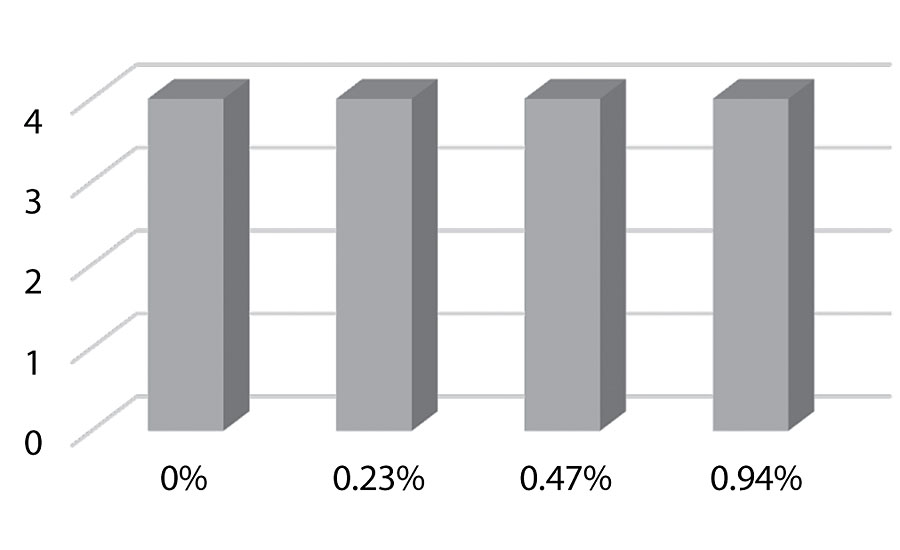
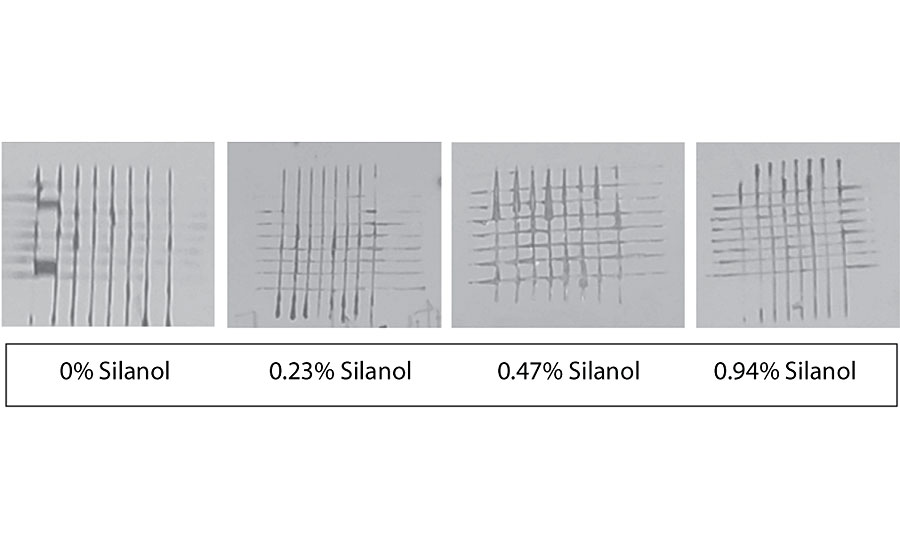
Dirt pick-up testing was performed by pouring a mixture of carbon black and black iron oxide onto a section of the coated Leneta chart. The powder was then spread with a brush to cover the whole section. It was baked at 50 °C for 1 hr, then cooled for 1 hr. The powder was rinsed with water and wiped off with a damp cloth.
We see an observable improvement with the incorporation of the silanol additive. As seen in Figure 24, the improvement is most significant at the 0.94% level. This work was qualitative. Optimization of the dirt pick-up test will be done by using a spectrophotometer to obtain quantitative results in future testing.
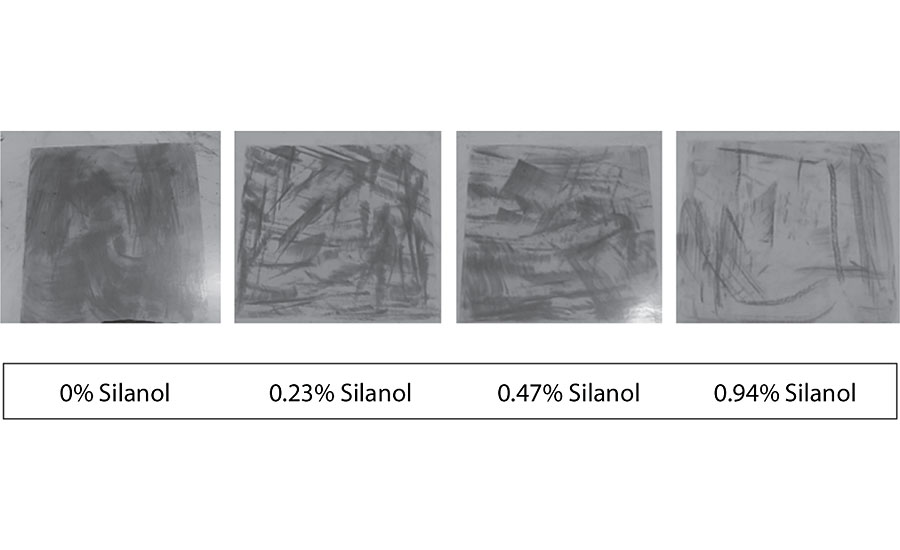
Conclusions
The goal of this work was to improve paint properties with the addition of higher branched/functional silanols. Silanols have been shown to improve adhesion to substrates, and depending on functionalities, increase coupling to the resin. This coupling can improve other film properties like chemical resistance, stain resistance and corrosion resistance. The silanol could also self-crosslink, providing another means to improve film properties. In theory, a lower-molecular-weight, higher-branched functional silane could provide the best film property enhancement. However, those are the most difficult molecules to both incorporate into a water-based solution and remain compatible in the resin/paint system.
Our work has shown that higher-molecular-weight, higher-branched/functional silanes can be made into a stable silanol in aqueous solution. The structure and level of functionality of the silane can determine the stability of the aqueous silanol in latex, and ultimately in the paint. When the stabilized silanol solution is created, it can be added during the latex production stage under lower shear mixing or during the higher shear paint formulation stage. Once incorporated into the latex and/or paint at the optimum level, improved properties can be obtained.
References
1 Altmann, S.; Pfeiffer, J. The Hydrolysis/Condensation Behavior of Methacryloyloxyalkylfunctional Alkoxysilanes: Structure-Reactivity Relations, Monatshefte für Chemie 134, 1081-1092 (2003).
2 Hallings, R.A. U.S. Patent 5,552,476, 1996.
3 Arkles, B.; Steinmetz, J.R.; Zazyczny, J.; Mehtas, P. (1992) Factors Contributing to the Stability of Alkoxysilanes in Aqueous Solution, in Silanes and Other Coupling Agents (ed. K.L., Mittal), CRC Press, pp. 91-104.
This paper was presented at the 2020 Waterborne Symposium in New Orleans. For more information, e-mail Sims.Michael@dystar.com.
Looking for a reprint of this article?
From high-res PDFs to custom plaques, order your copy today!