
The outstanding outdoor weatherability of poly(vinylidene fluoride) (PVDF) solvent paints is well demonstrated by over 30 years of commercial use on premier architectural structures.1 Commercial PVDF paints have a hybrid binder structure, being comprised of about 70 wt% PVDF and 30 wt% of a miscible acrylic resin in a semi-crystalline interpenetrating network (IPN)-type structure. The binder morphology is generated by baking at temperatures above 200 °C, beginning from a non-aqueous dispersion of the PVDF in a latent solvent such as isophorone.2 The PVDF polymer itself is highly inert and is completely resistant to UV-A and UV-B radiation.3 In this context, for highly weatherable paints, it is the acrylic polymer that must be considered the "weak link" of the binder - even though the acrylics that are used are among the most weatherable non-fluorinated organic polymers.
Since the PVDF solvent dispersions contain substantial levels of VOCs and require a high temperature bake, there has been recent interest to develop PVDF-based latex systems that give low-VOC coatings at ambient temperatures, while maintaining the advantageous properties of solvent PVDF paints. In this paper we consider latex systems containing PVDF resins and acrylics, at ratios similar to commercial solvent PVDF coatings. For these latex systems, differing latex morphologies can be obtained through cold blending (blending latexes of differing composition), or by making structured latex particles. The structured acrylic-modified fluoropolymer (AMF) latexes are prepared by a seeded emulsion polymerization process.
In this feature we also discuss the particular advantages that can be obtained for low-VOC coatings, which are based on AMF latexes made with an IPN-type morphology.
Film Formation in Composite Latex Systems
For latex systems containing a single polymer composition, e.g. acrylic latexes, the latex film formation process has been extensively studied, and it can be considered that its fundamental steps are well established, even if some minor points of controversy remain.4-5 The standard model for latex film formation involves three steps: (1) evaporation of bulk water and latex particle packing; (2) particle deformation with collapse of the interstitial voids between latex particles; (3) the interdiffusion of polymer chains across "membranes" between the residual particles leading to full mechanical properties. Polymer properties such as the glass transition temperature,6 particle size and particle distribution,7 molecular weight,8 and particle morphology9 have been shown to have a direct effect on the film formation process. Polymers with low Tg (referred to as soft polymers) are easily deformed and yield excellent film formation properties. However, the film produced will often be tacky, have poor mechanical properties and solvent resistance. High-Tg polymers or semi-crystalline polymers (referred to as hard polymers) yield particles that do not deform easily, and they require solvents as plasticizers to help the film formation.In order to get films with good mechanical and barrier properties while reducing the level of VOC used, composite latex systems involving two or more different polymer compositions can be used. Two ways to make composite latex systems are latex blending, and latexes with controlled particle architecture.
Latex blending has been shown to yield good film formation when hard/soft latexes are used. In this case, the soft latex will form a film and become the continuous phase while the hard particles will act as filler and impart mechanical properties.10-11 However, it is often difficult to obtain such films because in many instances the hard and soft particles are not compatible, the hard particles are not uniformly distributed, or the particle packing is not well controlled. Additionally, good control of particle packing is generally achieved only for a restricted range of hard/soft particle size ratios.
Most widely used are latexes with controlled particle architecture.12 Beginning with a well-defined latex particle morphology, the latex film formation process can be harnessed to obtain films with precisely tailored physical properties. The key requirement is that the latex particle morphology should substantially be maintained during the particle compaction and deformation stages of film formation. Thus, latexes with composites particles have received an increasing level of attention and have found applications as impact modifiers, automotive coatings, architectural coatings, membranes, etc.

AMF Particle and Film Morphology
In this work, we have studied the relationship between latex composition, film formation and final physical properties for films composed of a PVDF resin and an acrylic polymer. In particular we compared three air-dry latex approaches to traditional baked solventborne coatings (Figure 1):- a. Latex cold blend of a hard fluoropolymer latex with a soft acrylic polymer latex;
b. Core-shell morphology AMF latex (hard fluoropolymer core, soft acrylic polymer shell);
c. IPN morphology AMF latex (soft fluoropolymer and soft acrylic polymer).
In all three of the latex approaches studied, acrylic polymers were chosen that are thermodynamically miscible with the PVDF fluoropolymer resin,13-14 so that the latex and film morphology are determined primarily by kinetic rather than thermodynamic factors. This is one difference between these fluoropolymer systems and films made from structured acrylic latexes. Structured acrylic latexes are often based on immiscible polymers.
Because of their different particle structure and hardness, we expected the core shell structure and the IPN structure to yield very different film formation properties. Figure 2 shows the final film structure for the three latex approaches studied, assuming that the latex particle structure is maintained during the particle compaction and deformation stages of film formation. This appears to be a good assumption for all the acrylic-modified fluoropolymer latexes we have examined. For latex cold blends (blends of acrylic and fluoropolymer latexes), several lines of evidence suggest that there is insufficient resin mobility at room temperature to achieve good interdiffusion of the resin components, even using a coalescent.15 For a core-shell type AMF particle morphology (hard fluoropolymer core/soft acrylic shell), film formation tends to lead to "filled" film morphology (acrylic continuous phase with fluoropolymer inclusions).16 For both these cases, since the component resins are thermodynamically miscible, baking at temperatures above the fluoropolymer melting point can create a more homogeneous polymer morphology. For the third case studied, where the AMF latex particles have an IPN structure, a homogeneous film morphology can be generated at room temperature. For fluoropolymer acrylic hybrids, the IPN morphology has a number of practical advantages, particularly in terms of outdoor weatherability.
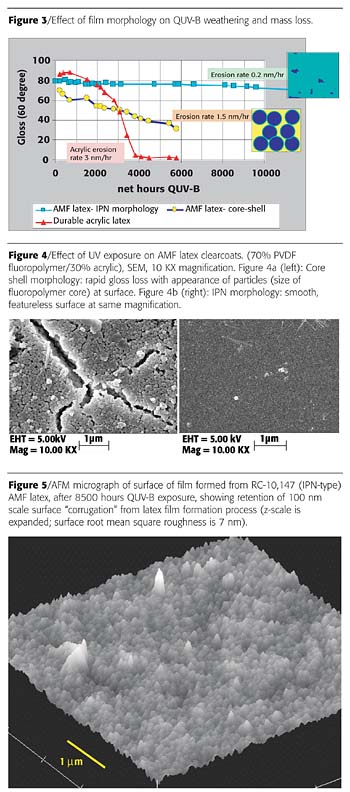
Weathering Advantages of the IPN Morphology
Since UV-B radiation is particularly effective in breaking down acrylics, we have used QUV-B weight loss experiments with clearcoats to probe the effect of film morphology on coating weatherability for fluoropolymer-acrylic hybrids generated from AMF latexes.17 Figure 3 shows results for different coatings, with mass loss rates converted to an effective rate of coating thickness loss (due to erosion or contraction), assuming it is the acrylic that is primarily being degraded. It can be noted that for an IPN-type morphology, the mass loss rate is extremely low - reduced by an order of magnitude from the rate expected based on the result for a bulk acrylic. This demonstrates that the fluoropolymer can have a synergistic protective effect on the acrylic, similar to what is observed in solvent PVDF systems.18SEM and AFM micrographs of the QUV-B weathered clearcoats dramatically illustrate this morphology effect. The film generated from a core-shell-type latex morphology shows a porous network of particles after QUV-B weathering, consistent with the erosion of an acrylic continuous phase, leaving the fluoropolymer cores behind (Figure 4a). Starting from an IPN-type morphology, on the other hand, the film is still featureless after weathering (Figure 4b). Higher-magnification AFM images show that the QUV-B surface erosion is so minimal for the IPN system that subtle, nanometer-scale surface corrugation features resulting from the latex film formation process are still intact after 8500 hours exposure (Figure 5).
The weatherability advantages of the IPN fluoropolymer system can also be seen in Figure 6, which shows Florida exposure results to date for a recent series comparing industrial maintenance paints over an epoxy primer. The acrylic-modified fluoropolymer latex formulations are showing outstanding gloss retention after two years Florida, whereas a number of commercial control paints are already showing significant gloss loss.

Other Performance Attributes
Outdoor weatherability is one striking advantage of commercial PVDF dispersion coatings, which is maintained in AMF latex coatings with an IPN morphology. In fact, as Tables 1 and 2 show, the AMF latex systems are able to maintain nearly all the favorable properties of high-bake PVDF systems, including good dirt pickup resistance and excellent adhesion to a wide variety of substrates.
Table 2 shows some results for the particular case of a white industrial maintenance-type latex paint, made from RC-10,147, an AMF latex with an IPN structure. The coating was dried at room temperature and contained approximately 240 g/liter VOC (less water). It can be seen that the chemical resistance of the RC-10,147 paint is comparable to that of commercial ambient cure 2-pack solvent urethane systems. In particular, resistance to strong acids, mineral spirits and industrial detergents is excellent.

Conclusions
The advent of the IPN fluoropolymer/acrylic latex provides the coatings industry with a number of interesting and novel opportunities:- Unlike conventional solvent-based coatings, low-VOC (or even zero-VOC solvent-free) aqueous fluoropolymer coatings are now possible. This allows the coatings formulator to consider truly high-performance outdoor, field-applied coatings that are VOC compliant and low in odor. Obvious candidate markets would include aftermarket maintenance coatings, high-performance architectural coatings and low-VOC OEM coatings and paints. Such formulations would be similar in make-up and manufacture as those typically made from conventional acrylic latexes that are currently widely used.
- Also, unlike conventional PVDF dispersion coatings, a baking cycle is not required with the IPN latex to form the essential ‘fluoropolymer/acrylic molecular alloy'. As such, substrates that have heretofore been deemed unsuitable are now open for consideration. Examples include flexible or rigid PVC, synthetic fabric, flexible roofing membranes and thermoplastic polyolefins.
The result is a technology platform that affords the coatings formulator the opportunity to consider markets and substrates that have heretofore been limited by VOC compliance and/or the need for OEM baking conditions.
This paper was presented at the International Waterborne, High-Solids and Powder Coating Symposium, February 2005, New Orleans, LA.
References
1 KYNAR 500® PVDF for coatings has been in continuous commercial production since it was introduced by the Pennsalt Company in 1965.
2 Iezzi, R.A. "Fluoropolymer coatings for architectural application," in Modern Fluoropolymers; John Wiley & Sons Ed., 1997; Chap. 14, 271.
3 Philippart, J.-L.; Siampiringue, N.; Strassel, A.; Lemaire, J. Makromol. Chem. 1989 190 413
4 Winnik, M.A. "The Formation and Properties of Latex Films", in Emulsion Polymerization and Emulsion Polymers; John Wiley and Sons, 1997; Chapter 14, 467.
5 Daniels, E.; Klein, A. Progress in Organic Coatings 1991 19 359.
6 Jensen, D.; Morgan, L. J. Appl. Polym. Sci. 1991 42 2845.
7 Sperry, P.; Snyder, B.; O'Dowd, M.; Lesko P. Langmuir 1994 10 2619.
8 Mazur,S.; Plazek, D. Progress in Organic Coatings 1994 24 225.
9 Kim, H.; Winnik, M. Macromolecules 1995 28 2033.
10 Chevalier, Y.; Hidalgo, M.; Cavaille, J.-Y.; Cabane, B. Macromolecules 1999 32 7887.
11 Winnik et al. J Coat Tech 68 (852), 39,1996 and Polymer 37, 5577, 1996
12 Okubo, M. Makromol. Chem Macrol. Symp. 1990 35/36 307.
13 Veeman, W.; Makromol. Chem Macrol. Symp. 1993 69 149.
14 Hourston, D.; Hughes, I. Polymer 1977 18 1175.
15 Sung, L.-P.; Vicini, S.; Ho, D.L.; Hedhli, L.; Olmstead, C; and Wood. K. A. Polymer 2004 45 6639.
16 Wood, K. A.; Hedhli, L.; Willcox, J. J. Coatings Technology 2002(924) 74 63.
17 Wood, K. A.; Cypcar, C.; Hedhli, L. J. Fluorine Chem 104(1) (2000) 63-71.
18 Wood, K., Macromol. Symp. 2002 187 469.