A Bio-Based Coalescent for Improving Latex Film Quality and Paint Performance

The present work focuses on the evaluation of a newly developed bio-based, zero-VOC coalescent for improving latex film formation and paint performance. The performance of the developed coalescent was evaluated in terms of film formation quality, and impact on final paint properties compared to a commercially available petrochemical coalescent. Results demonstrated that it is possible to achieve better properties in terms of paint film quality with an optimized environmental profile using the developed bio-based coalescent.
Introduction
The use of coalescents on latex paints is fundamental for ensuring the film formation during the drying process, and generating a paint film with good quality in terms of protection and aesthetic parameters. The coalescent will act during stage II of coalescence, reducing the minimum film formation temperature (MFFT) of the system, and during stage III, when the interdiffusion of polymeric chains and entanglement of neighboring particles occurs, resulting in the formation of a continuous and homogeneous film.1
The selection of a suitable coalescent involves not only the performance evaluation in terms of MFFT reduction, but also the impact on a paint’s performance and sustainable aspects, related to durability, bio-based content, and environmental impact, for example. To meet all the criteria, it is essential to be more assertive during the development or selection of new coalescents. The use of simulation Hansen Solubility Parameters (HSP) for coalescent and polymer compatibility can guide the choice of appropriate candidates in terms of performance. For the eco-efficiency evaluation, the use of Life Cycle Assessment (LCA) is suitable in the search for more sustainable materials.
Theoretical Evaluation — HSP and Polymer Compatibility Simulation
The simulations were performed with HSPiP Software, 5th Edition. The identification of a suitable coalescent involves plotting the HSP values of the candidate molecules with the parameters and radius of the polymer of interest and the parameters attributed to water. Suitable coalescents will occupy the area between the solubility envelope of water and the polymer.2 The most compatible coalescents will be located inside the polymer solubility sphere, which can be measured by the Relative Energy Difference (RED) values. Values lower than 1.0 indicate that the molecule is inside the solubility sphere and, the lower the value, the closer to the sphere center the molecule is located.3 A versatile coalescent should be compatible with different polymer latexes used on architectural coatings formulations. Table 1 presents the simulated values of HSP and the RED values with different chemistries of latexes for the developed bio-based coalescent and for a zero-VOC commercial coalescent, 2,2,4-trimethyl-1,3-pentanediol monoisobutyrate (TMPDMIB).
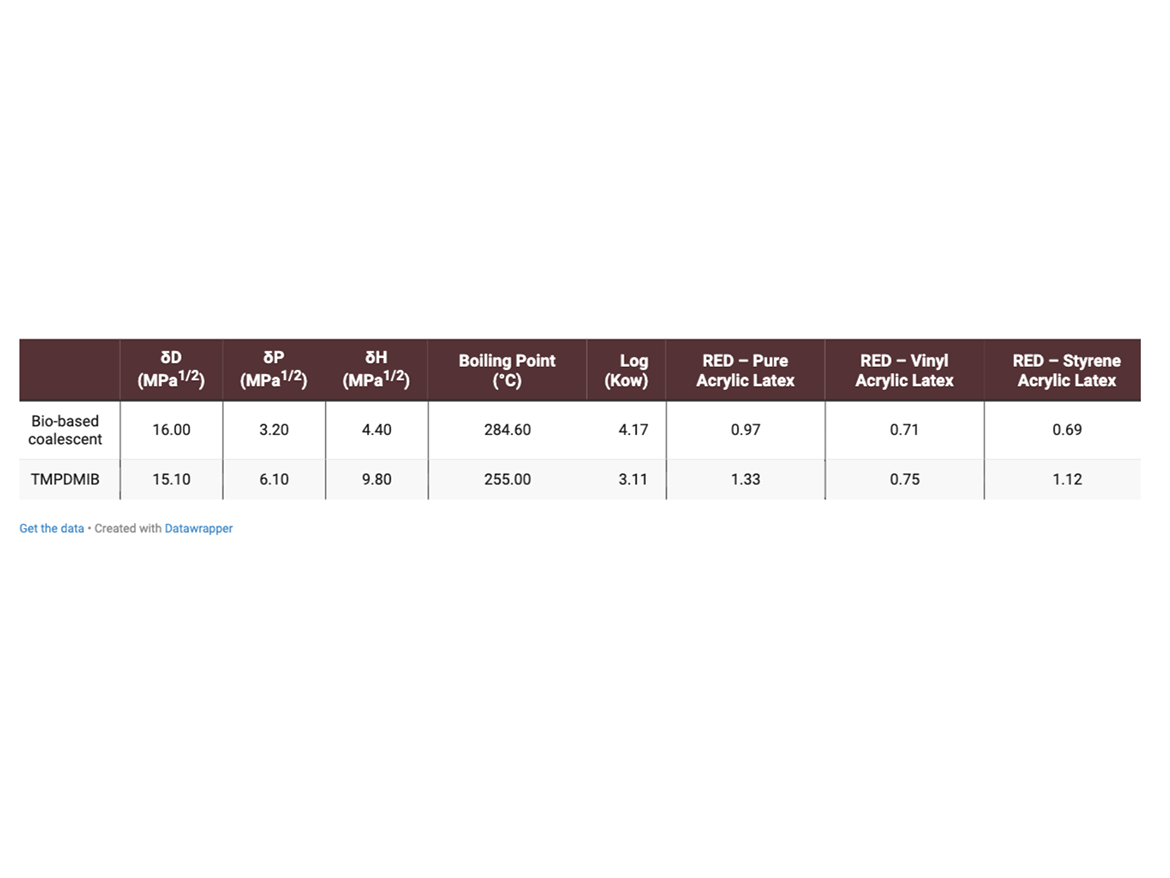
As can be seen in Table 1, the developed bio-based coalescent presents a more hydrophobic character in relation to TMPDMIB. It is also possible to observe that the developed bio-based coalescent presents a higher boiling point, which is interesting for not being considered a VOC according to Green Seal GS-11.4 In terms of polymer compatibility, it is possible to notice that the bio-based coalescent presents RED values lower than 1.0 for the main latexes used on architectural coatings formulations, and lower values in relation to TMPDMIB, indicating that the molecule has a better affinity with these types of latexes than TMPDMIB, which will contribute for generating a more coalesced film with improved quality and properties.
Results and Discussion
Coalescent Performance — MFFT and LTC (Low Temperature Coalescence)
A good coalescent should reduce the polymer MFFT during stage II of coalescence with the minimal concentration. Typically, the target MFFT is between 0 °C and 5 °C. Figure 1 presents the results of MFFT as a function of coalescent concentration over polymer solids content (pcp) for different types of latexes.
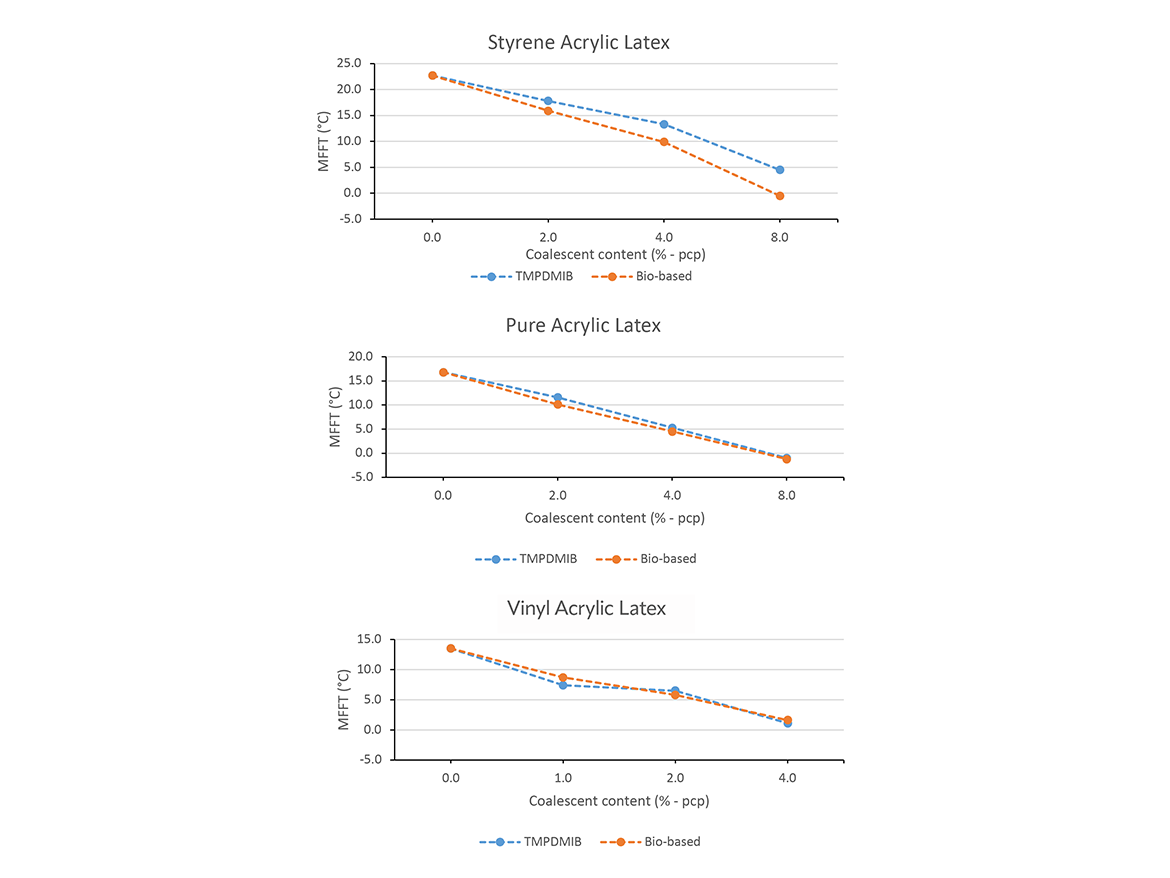
According to Figure 1, the bio-based coalescent presented similar MFFT reduction in relation to TMPDMIB for the pure acrylic latex and for the vinyl acrylic latex, and, presented better performance for the styrene acrylic latex. However, the efficiency and selection of a coalescent cannot be measured only by the MFFT reduction. Another important test to evaluate the effectiveness of a coalescent is the LTC, which simulates real-life conditions under low temperatures. A suitable coalescent should present a balance between polymer affinity, water miscibility, and evaporation rate.5
The tests were performed according to ASTM D7306-7 on a semi-gloss paint — PVC of approximately 32.0%, pure acrylic latex content of 35.0%, and coalescent concentration of 8.0% pcp. The wet film thickness used was 400 µm. Figure 2 presents the photos of the films and the images on an optical microscope, BX-51 — Olympus, with 50x magnitude.
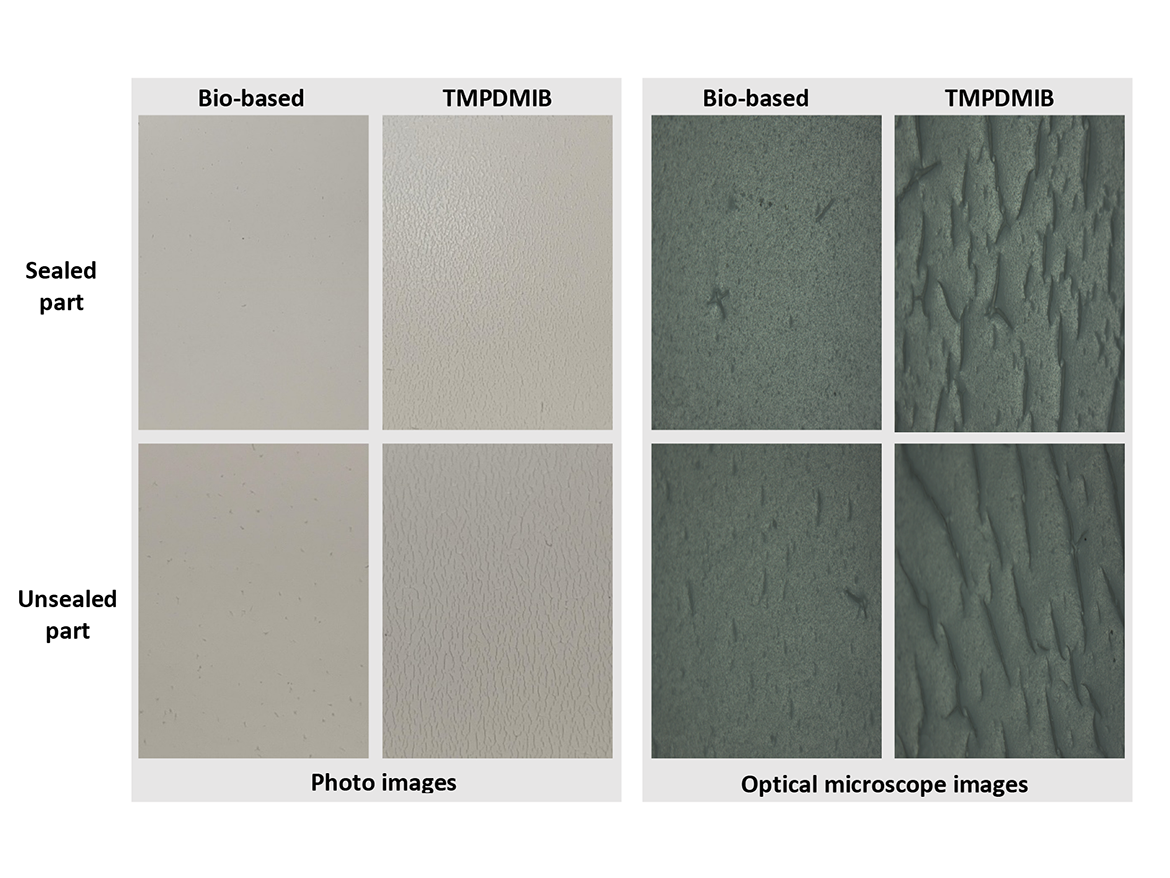
Figure 2 shows that the paint formulated with the bio-based coalescent presented a better film formation in relation to the same paint formulated with TMPDMIB. On the sealed part, the paint formulated with the bio-based coalescent presented no cracking (rate 5), while the paint prepared with TMPDMIB presented severe cracking (rate 1). These results demonstrate that, in a real-life condition, the concentration of TMPDMIB in the paint formulation should be increased for ensuring the film formation, differently from the results observed for the MFFT test on an acrylic latex. The evaluations on the unsealed part show a worsening for both coalescents, more critical for TMPDMIB. These results reinforce the higher polymer affinity that the developed bio-based coalescent presents in relation to TMPDMIB, as predicted by using HSP simulation.
Film Quality Assessment Using Atomic Force Microscope (AFM)
The coalescent will impact the film formation process not only during stage II, but also during stage III of coalescence. For this reason, it is important to evaluate the impact of the coalescent on the film formation quality and on paints properties.
As a first evaluation, films of a pure acrylic latex and a styrene acrylic latex were evaluated by AFM using a commercial setup — NX-10, Park Systems, Korea. The assessment was realized for film latexes without and with coalescents. The films were applied on a Leneta P121-10N substrate with a 150 µm drawdown bar and dried for 7 days in an air-conditioned room with controlled temperature (25 ± 2 °C) and relative humidity (60 ± 5 %). After this period, the films were evaluated by AFM. The obtained topographic and phase images are presented in Figures 3 and 4, and the statistical values, average height, average roughness (Ra), and root mean square roughness (Rq) are presented in Table 2.
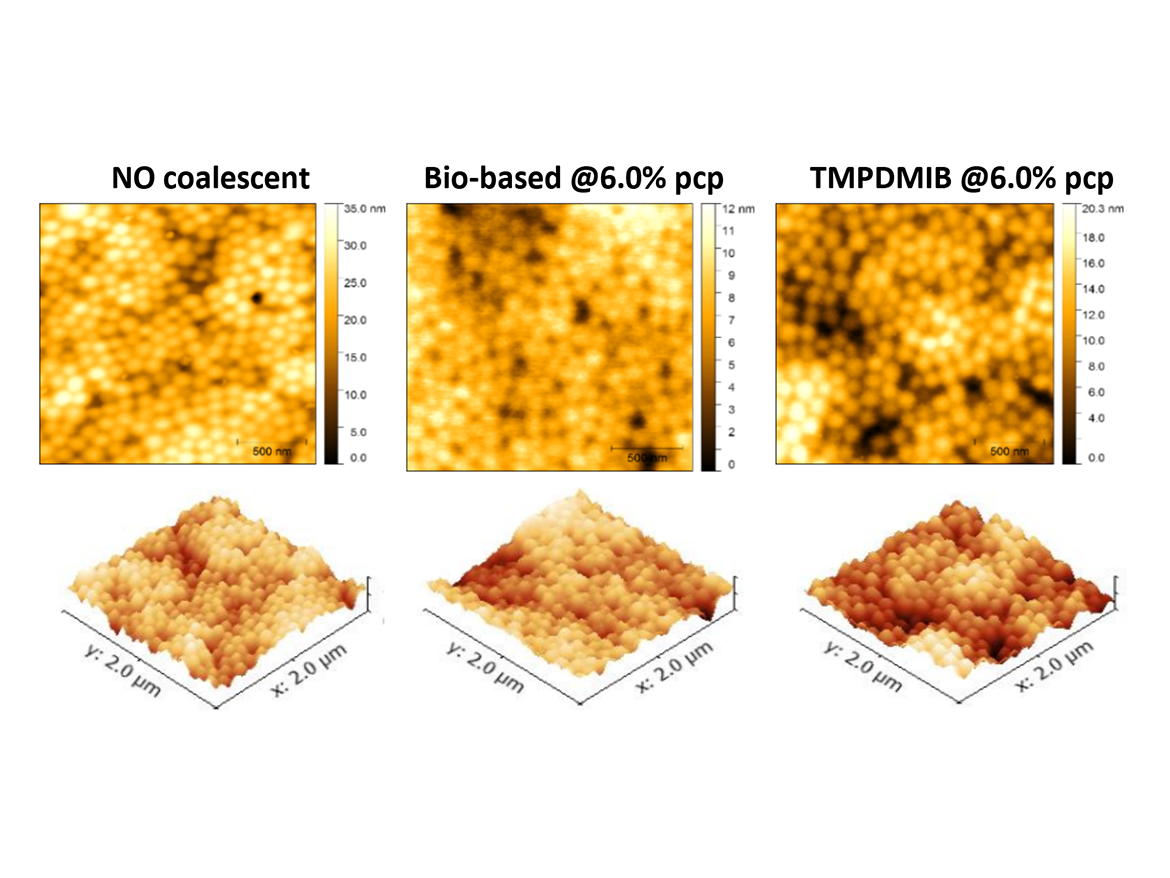
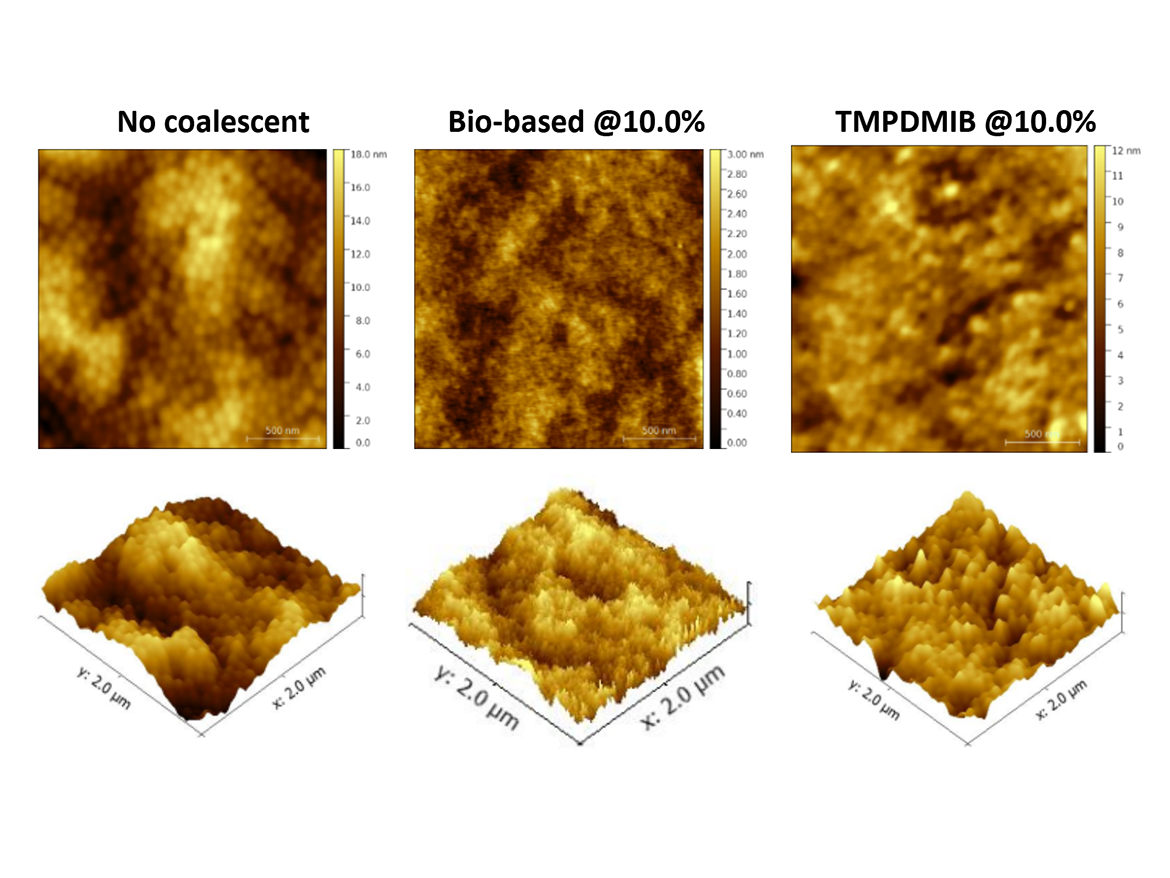
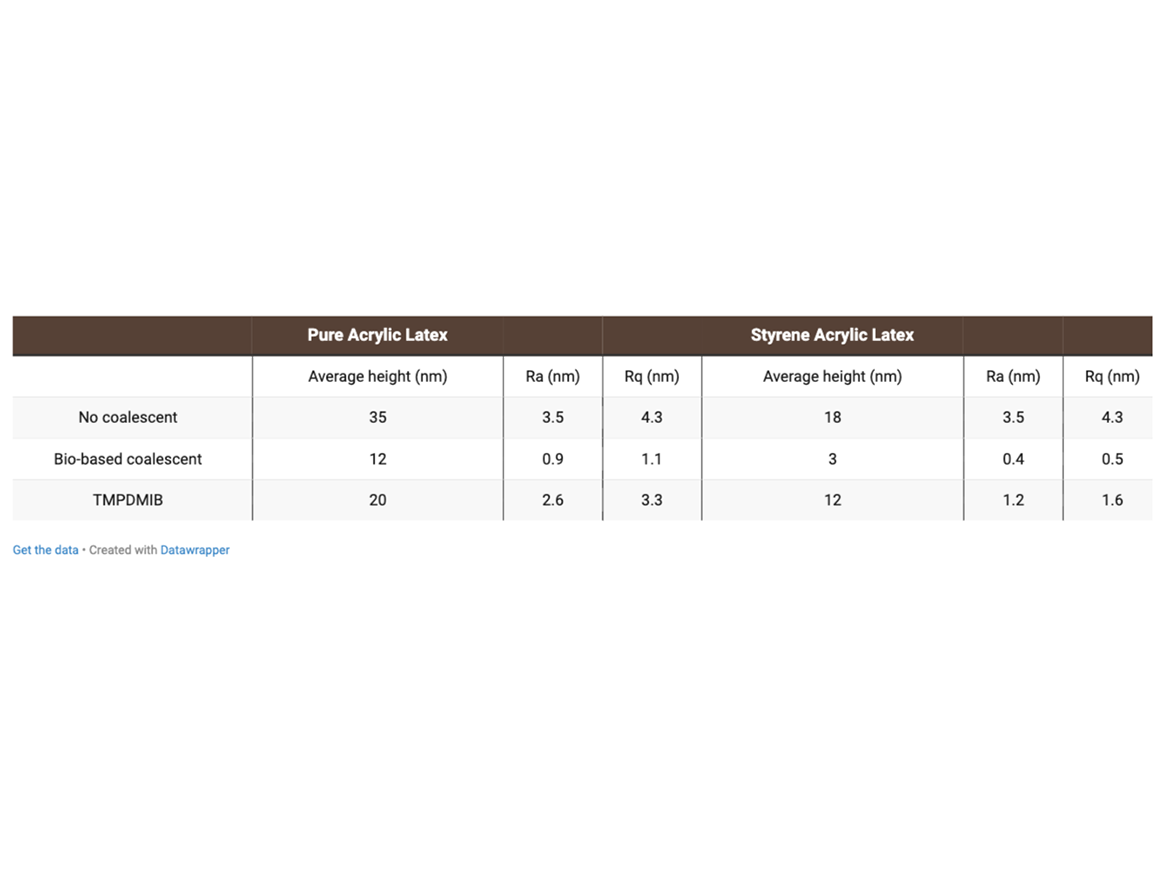
As can be seen on 2D images in Figures 3 and 4, the latex films without coalescent presented visible particle domains. For the pure acrylic latex, the domains can still be seen for the coalesced films but are smoother for the film with the bio-based coalescent in relation to TMPDMIB. The same behavior is observed for the styrene acrylic latex films, however, for the film with the bio-based coalescent, it is not possible to observe particle domains, indicating a better coalescence process. The 3D images in Figures 3 and 4, and the statistical values presented in Table 2, corroborate this behavior — latex films without coalescents presented a rougher surface in relation to the coalesced ones. Comparing the results between the coalescents, it is possible to observe that the films with the bio-based coalescent presented a smoother surface in relation to TMPDMIB. These results indicates that the bio-based coalescent presents a better interaction with the polymers and, consequently, a better coalescence process, which results on an improved film quality.
Coalescent Performance on Paint Formulations
The coalescent performance on paints was evaluated for different properties in different types of formulations, varying the paint PVC, chemistry of latex, and raw materials. In this article, the focus is to show only some results to demonstrate the performance improvement when the developed bio-based coalescent is used in key properties related to film formation quality. Other application results are available.
The scrub resistance test was performed with paints prepared at different PVCs and with different latexes according to ASTM D 2486 Method A. The results are presented in Figure 5.
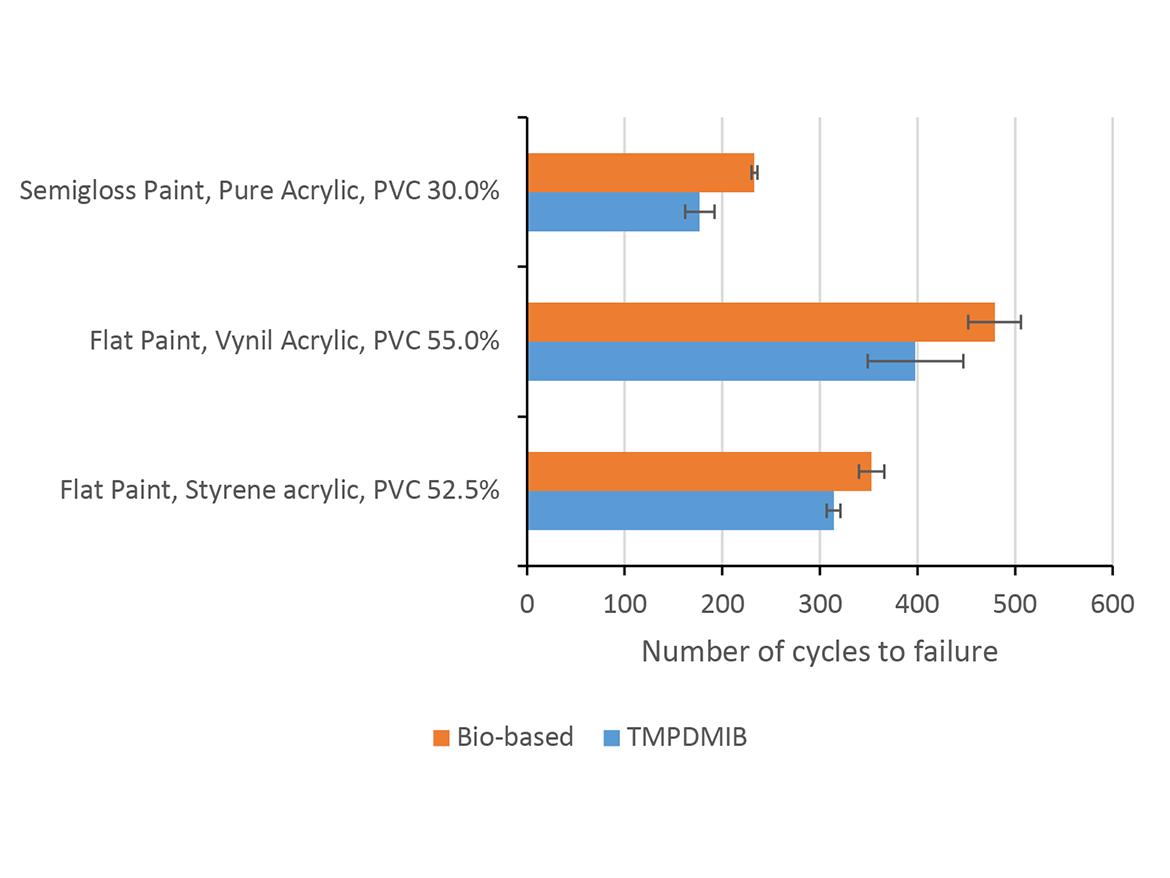
As can be seen in Figure 5, at different PVCs and with different latexes, the use of the bio-based coalescent in a paint formulation improves the scrub resistance performance in relation to TMPDMIB. These results are in accordance with the best film formation observed for the latexes coalesced with the bio-based coalescent in the AFM evaluation.
For the leaching resistance evaluation, a flat paint formulated with a styrene acrylic latex, PVC 52.5%, coalescent content of 10.0% pcp, and tinted with a blue pigment concentrate at 2.0% (weight over paint) was used. The performance was evaluated according to an internal methodology — the paints were applied on a Leneta P121-10N with a drawdown bar of 150 µm and dried in a cold chamber at 5 ± 2 °C for 4 hours. After this period, the color was measured, and the paint films were immersed in demineralized water for 30 minutes. After the immersion, the paint film was removed from the water and dried in an air-conditioned room. The color was measured, and the water on which the paints were immersed was collected for surface tension measurements. The results in terms of color change, ∆E, and surface tension are presented on Table 3.
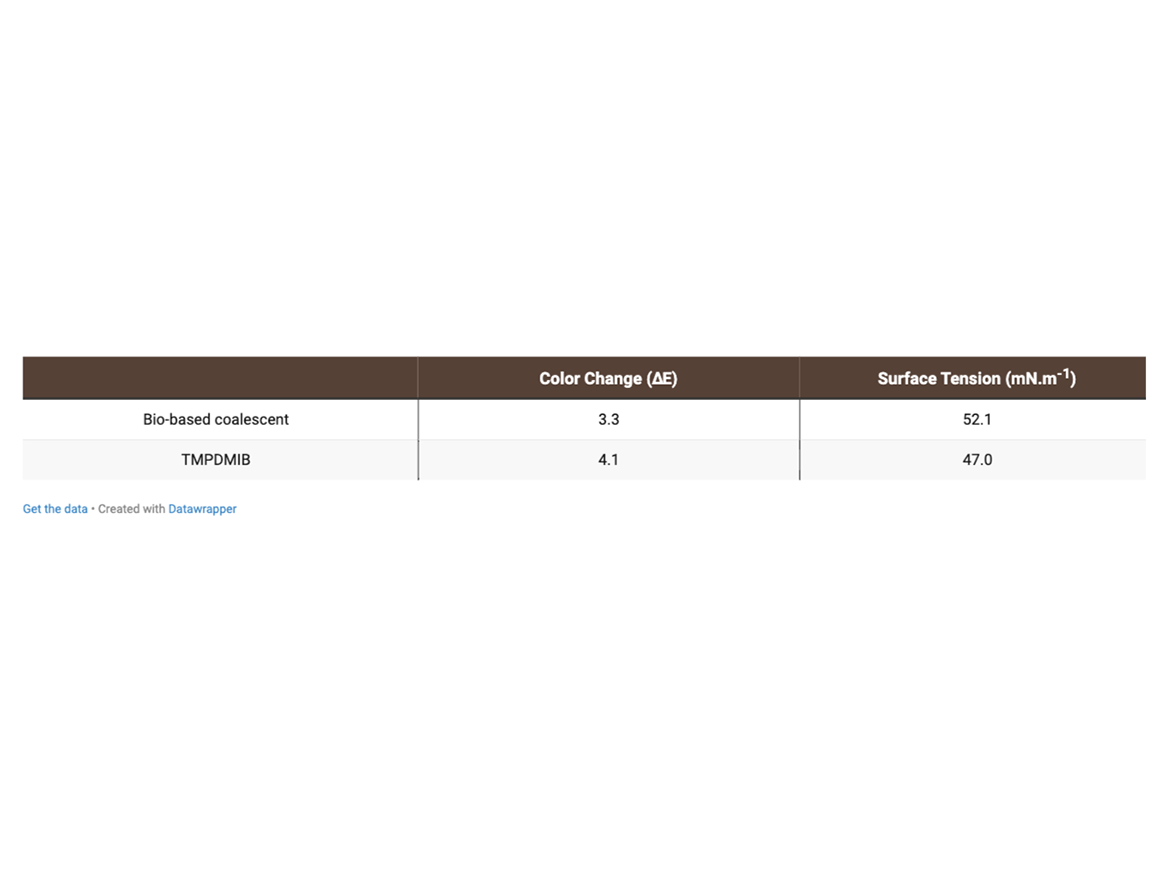
As can be seen in Table 3, the paint formulated with the bio-based coalescent presented a lower color change, indicating better water resistance in relation to the paint formulated with TMPDMIB. In terms of water surface tension, it is possible to observe that the water in which the paint formulated with the bio-based coalescent was immersed presents a higher value in relation to the water in which the paint formulated with TMPDMIB was, indicating that a lower amount of leachable materials migrated from the paint film to the water.
Life Cycle Assessment (LCA)
The developed coalescent presents a bio-based content of 100% according to ISO 16128, however, for a more appropriate evaluation, the eco-efficiency analysis was based on the use of the environmental performance assessment tool, the LCA, with approach 'cradle-to-grave' and for a Functional Unit of “to protect a surface of 50 m2”. The product system models have been generated by primary and secondary data, and no multi-functional situations were identified. The comparison considered impacts in the form of climate change, human toxicity, water depletion, fossil depletion, and smog. The complete study was the theme of a master’s dissertation and can be downloaded.6 Figure 6 presents the environmental profiles of the different paints in relative terms.
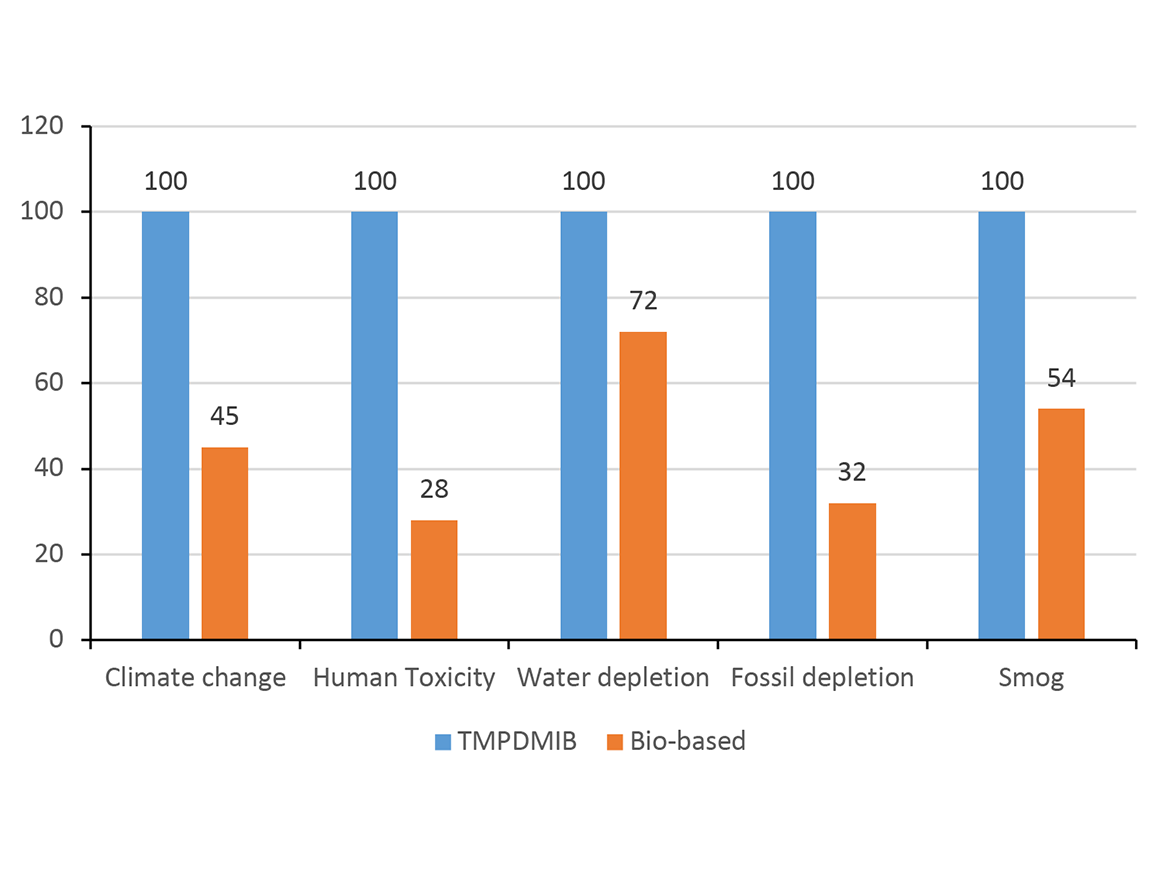
Figure 6 shows that, for all the categories evaluated, the use of the developed bio-based coalescent in the paint formulation contributes to improving the environmental profile. Improvements were observed on impact categories more related to the stages that precede the transport of the coalescent to the paint factory, that is, from the cradle to the gate (climate change, human toxicity, water depletion and fossil depletion) and on a category more influenced by the stage of use of the product exactly where emissions of coalescents occur (smog).
Conclusions
The present work demonstrated that the use of simple prediction tools can speed up and improve assertiveness of new product development and choice. The assessment of film quality by complementary techniques can be employed for a better understanding and correlation with paint properties — it was observed that latexes with an improved coalescence process generated paints with improved properties. The work also showed that, beyond the eco-efficiency gain, bio-based products can outperform petrochemical products, as showed in the comparison performed on latexes and paint formulations between the new bio-based coalescent developed and TMPDMIB.
References
1 Rafael, C. J.; Salvato, P.S. [et al]; [coordenação de Silmar Barrios]. Manual Descomplicado de Tecnologia de Tintas: um guia rápido e prático para formulação de tintas e emulsões. Blucher, 2017.
2 van Loon, S.; Fricker, B. Using HSP to select coalescents and improve film formation, SpecialChem, 2020. Available on https://coatings.specialchem.com/tech-library/article/using-hsp-to-select-coalescents-and-improve-film-formation, accessed in April 2021.
3 Abbott, S.; Hansen, C.M.; Hiroshi, Y. Hansen Solubility Parameters in Practice. Hansen-Solubility.com, 2020.
4 Green Seal GS-11, Green Seal Standard for Paints, Coatings, Stains, and Sealers, Edition 4.0, September 2021.
5 Palasz, A. Water miscibility differences in coalescing agents and the influence on latex paint properties. PCI Paint & Coatings Industry, 2021, 37.
6 Rosa, F. Eco-efficiency analysis of coalescent substitution in decorative paint formulation. Masters’ dissertation. Escola Politécnica, Universidade de São Paulo. São Paulo, 2019.
Looking for a reprint of this article?
From high-res PDFs to custom plaques, order your copy today!