Size Matters in the Coatings Industry

There are a huge variety of coating colors and finishes for many different applications, and all of them have one thing in common - their properties are dependent on the size and the mass- or volume-weighted size distribution of the particles used in their ingredients. The average particle size of coating ingredients has decreased over the years from around 0.5 µm to 0.2 µm, in part because of improvements in technology. In addition, there has been a dramatic shift away from solvent-based coatings to water-based coatings in an effort to reduce VOC emissions. The combined result of this has meant a lot of research to find new raw materials and ways to stabilize the rheology of the coatings and maintain their performance. For example, cellulosic thickeners were favored when solvent-based coatings dominated the market, but they were found to decline in performance in water-based systems, and associative thickeners were subsequently developed to achieve the desired rheology.
Having the correct particle size is obviously crucial for reproducible characteristics, and this can be illustrated with one of the most common white pigments, titanium dioxide (TiO2). TiO2 provides opacity and whiteness in, for example, architectural coatings by scattering visible light, and it has one of the highest refractive indices of any common compound.
It is usually treated and coated with a number of organic compounds because it is photoreactive and can catalyze reactions in media that lead to degradation. In addition, the coating can help improve its compatibility and dispersion characteristics. The number of layers of these compounds, their nature and their thickness can all be manipulated to control and alter the optical properties of TiO2.
However, it sounds simpler in theory than it actually is in reality. There is a very fine balance between particle size and optical properties that manufacturers must achieve to get the right result. Up to a point, the more TiO2 particles that a dispersion contains the more light is scattered outwards, making the paint appear whiter. In addition, the variation between scattered intensity and particle size is a complicated function varying at one extreme by the cross-sectional area (size squared) and at the other, for a nonabsorber like TiO2, by size raised to the 1st power. Thus, a point will be reached where further reduction in particle size causes scattering to drop off and transparency results. The optimum pigment volume concentration for a common size distribution of TiO2 is 17% by volume. Too high a concentration is not only costly and may lead to viscosity problems, but also means that neighboring particles are more likely to join together and reduce scattering (opacity).
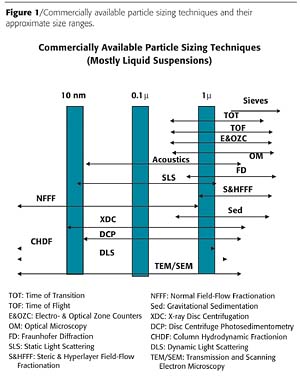
A Rough Guide to Particle Sizing
The points above are overly simplified to illustrate the relationship between coatings properties and particle size, but it is not hard to see how applicable these points are to any system that uses dispersed particles. But how exactly is particle size measured, bearing in mind that particles can be such different shapes? This is a tough question to answer because the size of a completely irregular, 3D object can be defined in a large number of ways, not all of which are correlated to product performance. However, to be useful, particle size often needs to be described by a single, equivalent diameter often called the equivalent spherical diameter, or ESD.Generally speaking, irregular particles include sharp, angular pointy particles, agglomerates of smooth and pointy particles and just about everything in between. In principal, one could think of many characteristics defined by geometrical principles to define particles. In image analysis, there are tens of such definitions, such as longest chord, shortest chord, fractal dimension, radial distribution signatures and many, many more. In fact, there is no general agreement on the minimum set to define the size distribution of a particular sample. Thus, one has to rely on instrumental methods that rely on the ESD concept.
Some property is measured and set equal to that of the equivalent sphere. In practice, this concept has two subdivisions: geometric-defined ESDs and technique-defined ESDs. The geometric-defined ESDs include the diameter of a sphere that has the same perimeter, surface area, volume or projected cross-sectional area of the actual particle. Obviously, such results require an image analyzer and a lot of work to characterize a large, statistically relevant sample. With technique-defined ESDs, the diameter is defined as that of a sphere that resulted in the same property as measured for the actual sample.
In sedimentation, for example, the ESD is the diameter of a sphere that sediments at the same rate as the actual particle. This is referred to as the Stokes diameter. For other techniques, analogous definitions apply. Since the diameter obtained will depend on the technique, ESDs from one technique will not necessarily agree with ESDs from another unless the particle is truly spherical. In fact, the more globular the particle, the more the agreement is to be expected. And the more irregular, the less the agreement is to be expected. Of course, if the particle is quite irregular, then the properties of interest might best be determined using an image analyzer and putting up with all the difficult ways of defining size.
In reality, particles are usually a variety of irregular shapes, and the errors this can cause when determining particle size and distribution can be reduced but not eliminated entirely. The amount by which the error can be reduced depends on the shape of the particle and the sizing technique used. With sedimentation instruments, for example, the ESDs, or Stokes diameter, is close to the geometrically defined, volume ESD. Thus, sedimentation has the advantage that its instrument defined ESD is roughly equivalent to a geometrically defined ESD.
All of this boils down to the fact that no one particle sizing technique is better than another. The method that is chosen will depend on the application, the material under study and what characteristics matter the most. For quality control applications, relative changes from batch-to-batch are more important than accuracy, so reproducibility is the main issue of concern. Another parameter that must be considered carefully is resolution. Can the particle sizing technique under consideration distinguish between different sized particles in the same sample and is the signal-to-noise ratio of the instrument affecting the fundamental resolution? Very broad resolutions can sometimes hide practical and significant information and if the resolution of the instrument is unknown is the information being produced useful?
For the coatings industry, however, accuracy has always been extremely important because of the impact that subtle differences in particle properties can have on the performance of the materials used. Not only must all the sample and instrument variables be known, but good sampling and sample preparation must have been used as well. Figure 1 shows the range of commercially available particle sizing techniques and the size ranges they cover. The coatings industry has traditionally favored sedimentation techniques, as they seem to have a significant edge on accuracy and high resolution.
Particle sizing below a few microns though is not always easy, but for those particles that absorb X-rays, adding a centrifuge into the instrument set-up, as Brookhaven Instruments has done with its BI-XDC X-ray Disc Centrifuge, allows faster, more accurate results to be obtained. And unlike light scattering and diffraction techniques, the mass- or volume-weighted size distribution of the particles can be obtained directly without having to make corrections for the sample's optical properties.
Conclusion
As this article has shown, there are overlapping choices to be considered when choosing a particle sizing technique. There are lots of pitfalls too, the main three being:- trying to satisfy several different requirements with one instrument;
- not understanding, or misunderstanding, the best use for different techniques;
- incorrect sampling and sample preparation techniques when comparing instruments and techniques.
For more information about the applications of particle sizing in the coatings industry, contact Dr. Bruce Weiner, Brookhaven Instruments Corporation, 750 Blue Point Road, Holtsville, NY 11742; phone 631/758.3200; fax 631/758.3255; e-mail info@bic.com; or visit www.bic.com. Or contact Dr Peter McFadyen, Brookhaven Instruments Limited, Chapel House, Stock Wood, Redditch, Worcestershire, B96 6ST, UK; phone + 44 (0) 1386 792727; fax + 44(0) 1386 792720; e-mail info@brookhaven.co.uk; or visit www.brookhaven.co.uk.
Looking for a reprint of this article?
From high-res PDFs to custom plaques, order your copy today!