Novel, "Breathable" Polyurethane Dispersions
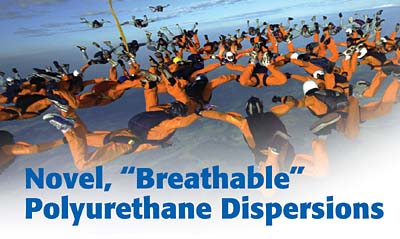

At first glance, the two key requirements for a "breathable" coating seem impossible to reconcile because their demands are inherently opposite. To overcome this apparent incompatibility, several "Maxwell's Demons"1 were invented capable of distinguishing between the two forms of water: liquid and vapor. These "smart" polymeric compositions were branded "breathable." Strictly speaking, products permeable to water vapor should be called "perspirable," but the breathable designation is generally accepted by the textile industry.
The moisture vapor transport rates of various polymers are shown as a continuum in Figure 1. Available products range from virtually impermeable polymers of vinylidene chloride and isobutylene to hydrophobic styrene-butadiene polymers and styrene-butyl acrylate emulsion polymers. There are also somewhat more hydrophilic polymers of ethyl acrylate and vinyl acetate as well as significantly more hydrophilic polyurethane compositions.
The entry threshold for breathable applications is set by industry at a minimum of 500 g/m2/day as tested by the ASTM E 96 upright water cup method. A recent publication2 comparing the various methods for measuring the water vapor permeability of fabrics does confirm that the MVT performance on existing commercial fabrics ranges from ~450 to 1,000 g/m2/day. The inverted ASTM E 96 water cup MVTR results range from ~3000 to 7500 g/m2/day. The constructions in the study included both laminated and coated fabrics with durable, water-repellent finishes.
The demand for moisture management and highly breathable fabric constructions continues to grow and drive innovation worldwide. Another notable stimulus for technology development is regulatory pressure to reduce or eliminate HAPs and VOCs from the manufacturing process.
This paper introduces a new, patent-pending3 line of breathable waterborne PUDs (polyurethane dispersions) that meets the most stringent of technical requirements and provides a virtually VOC-free solution to the customers' challenges. The line is offered under the Permax trade name and represents an attractive alternative to existing high-MVT constructions.
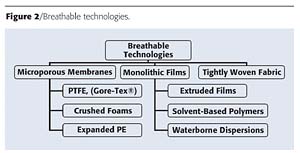
Breathable Technologies
Industrial coaters and laminators use three primary methods to manufacture waterproof and windproof breathable fabrics: microporous structures; monolithic polymer films and coatings; and tightly woven fabrics. The technologies that are used to manufacture breathable fabrics are summarized in Figure 2. They impart both MVT and barrier properties to the fabric.
There are a variety of polymers used in the production of high-performance MVT constructions. These polymers include PTFE, expanded polyethylene, hydrophilic polyurethanes and polyamides. The materials are offered in several product forms - films, direct extrusion resins, solvent-based products and waterborne offerings - and vary in terms of VOC levels depending on the product.

Microporous Technologies
This includes polytetrafluoroethylene (PTFE); expanded polyethylene; crushed foam coatings; and coatings that become microporous during drying, curing, and salt or solvent extraction. One of the industry benchmarks is Gore-Tex® - microporous polytetrafluoroethylene. Two images of microporous breathable coatings are shown in Figures 3 and 4.The pores in the films are large enough for water vapor molecules to pass through freely but small enough - several microns - to exert strong capillary forces that prevent the penetration of liquid water.
Unfortunately, the pores frequently become clogged with dirt and soap in the process of wearing and washing in laundry cycles. Such a propensity ultimately leads to the deterioration of MVT performance over long periods of time. Another drawback of the microporous films is that the very pores that provide for breathability may also allow undesirable vapors, microscopic particulates and liquid to penetrate the fabric. Consequently, a microporous structure alone may not serve as the optimal source of protection in protective apparel applications. Instead, combinations of microporous and monolithic coated films must be used (Figures 5 and 6). Moreover, in the case of laminates, the use of adhesive to bond the film to the fabric is not unusual.

Monolithic Film
The monolithic film approach utilizes continuous hydrophilic coatings, or laminates, that rely upon the rapid diffusion of water vapor through a membrane. The high MVTRs are afforded through the incorporation of up to ~40% poly(ethylene oxide) (PEO), a very hydrophilic, water-soluble polymer.4,5 Due to the lack of specific interactions between PEO and water, the diffusion of water molecules through the PEO-modified films is very rapid.Thermoplastic Polyurethanes (TPUs)
Hydrophilic polyurethanes are widely used in the production of highly breathable monolithic films. Their market share in breathable applications has grown as a result of superior mechanical properties such as strength, resilience, abrasion resistance, durability, a combination of elasticity and toughness, excellent adhesion to a variety of polar and non-polar substrates, oil and solvent resistance, and the ability to tailor products for a wide variety of applications through a virtually unlimited selection of starting materials. Particularly, polyurethanes offer an excellent means by which to tailor soft fabrics and provide a more favorable cost performance than PTFE.For example, Noveon, Inc., has a series of new Estane® high-MVT TPUs that are available in the form of dry resin for extrusion or blown film applications. The MVT properties of monolithic films made from these products exceed those of commercial copolyesters, copolyamides and conventional TPU.
The breathable TPU film and sheet products offer distinct advantages over traditional microporous products in combining higher barrier properties against moisture with excellent tear and puncture resistance. In coated fabrics applications, these TPU products offer soft hand, excellent wash resistance and low-temperature flexibility.
Solventborne Systems
Solventborne systems are the volume leaders in North America's high-MVT market. Solventborne coatings customers often use different solvents or solvent blends because manufacturing sites are permitted to emit only one type of solvent. Required emissions reporting, flammability hazards, permissible exposure values (as low as 10 ppm TWA), and suspected carcinogenic status are some negative factors associated with the use of solventborne coatings. Environmental6 and economic pressures at several coatings operations are forcing changes to be made.
Solventborne coatings incur a multitude of costs that waterborne systems do not. There are several economic drivers that encourage the transition from solvent to waterborne textile coatings: federal, state, and local EPA regulations on record keeping of VOC and HAP emissions; capital investment in MACT (maximum achievable control technology); explosion-proof process equipment; flammable warehouse storage; hazardous waste disposal; and increasing energy, maintenance and insurance costs for solvent recovery or incineration processes. Similar environmental and cost pressures are found around the world in varying degrees. In the United States, the EPA has issued new compliance rules to cut HAP emissions in the textile coatings, finishing and printing industries by 60% by May of 2006.6

Polyurethane Dispersions
Although extrusion-grade and solventborne polyurethanes have successfully established themselves in the breathable arena, the demand for easy handling and VOC-free formulations dictates the development of waterborne products. A waterborne, high-MVT polymer offering would be beneficial at many fabric coaters provided it had the desired performance characteristics. A successful waterborne product would also expand the number of potential coatings lines beyond those equipped with explosion-proof solvent-handling equipment.PUDs can be produced by several processes.7 All of them include two main steps: making oligomers or polymers and then dispersing them in water. Attempts to make PUDs with a high PEO backbone content failed due to the dramatic swelling of these polyurethanes in water. In order to produce dispersions with manageable viscosity, a large amount of water must be used. As a result, it is impossible to produce breathable PUDs with PEO in the main chain with practically acceptable solids content.
One way of minimizing the swelling is to combine two different hydrophilic groups in the same polymer chain: one main-chain PEO in the soft segment and another ionic group in the hard segment.8
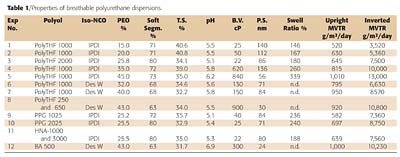
In the course of developing the product, we asked ourselves: "What would happen if we changed the topology (architecture) of macromolecules and moved PEO from the main chain into the side chain?" (Figure 7). We speculated that the two functions would be separated. The main-chain backbone, while remaining very hydrophobic, would stay "dry," resist overall swelling in water and ensure good physical properties. The tethered hydrophilic side chains would become plasticized with water upon contact with humidity, and provide breathability.
Polyurethanes with side-chain PEO are well known in the PUD industry in the form of nonionic PUDs.7 A few examples of the monomers that can be used to prepare nonionic PUDs are shown in Figure 8.9-11
The finding that most surprised us was how much side-chain PEO we could incorporate into the polymer and still be able to make relatively high-solids dispersions (~30-40+%) and durable coatings with high breathability. In experiments using the same level of PEO in the main-chain, all dispersions gelled.

Results and Discussion
Table 1 summarizes the compositions and properties of experimental breathable dispersions. These new polyurethane dispersions can be synthesized with various types of main-chain polyols as well as aromatic or aliphatic isocyanates. The MVTR values measured by a water cup method similar to ASTM E 96, on nylon coated fabric at approximately 1.0 oz/yd2 (33 g/m2) coating (Figure 9), ranged from 500 to 1000+ g/m2/day.
Figures 10 and 11 show the effect of PEO content on the MVT rates of dried coated fabric. As expected, moisture permeability is a strong function of PEO level. By far, it outweighs all other variables, including the type of isocyanate, polyol and soft segment content, in controlling the MVTR.

An unexpected finding was the dependence of breathability on the length of the side-chain PEO (Table 2, Figure 13). We hypothesize that as the length of the side-chain PEO increases, it becomes more and more incompatible with the polymer backbone, especially as PEO becomes plasticized with water upon contact with humid air. As a result, the PEO phase separates into highly water compatible and, therefore, permeable channels, which provide for higher MVT rates. This phenomenon is analogous to electric conductivity; water molecules diffuse through paths of least resistance, just as electrons flow through paths of highest electrical conductivity.

Product Performance
Several Permax products were fully compounded to a spectrum of cost-performance balances and applied onto fabric in production scale trials. The coated fabrics performed competitively with commercially available high-MVTR-coated and laminated products (Figure 14).
Wash durability in 25 hot-water home laundry cycles was tested with fully formulated coatings on nylon fabric. Hydrostatic head resistance was measured by a Mullen type tester.12 Figure 15 shows the results of this testing. The choice of formulation ingredients, crosslinking agents, coating weight and methods greatly affected wash durability. In many cases, the failure mode was a tearing of the nylon fabric in places where the coating was stronger than the fabric. The Mullen burst retention after 25 laundry cycles ranges from 25% to 65% of the original unwashed fabric values. The typical initial Mullen burst pressure ranged from 80 to 120 psi. Such a performance is equivalent to or better than the waterborne controls tested in this study.
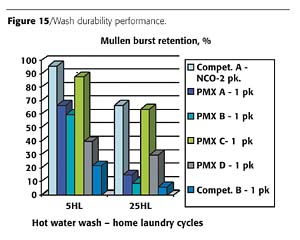

Table 3 lists the properties of the commercially available Permax dispersions.
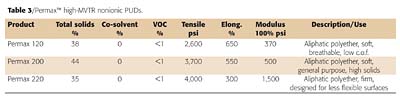
Conclusions
The demand for moisture management and highly breathable fabric constructions is growing. Regulatory pressures to reduce or eliminate HAPs and VOCs remain strong globally. Permax hydrophilic nonionic polyurethane dispersions and compounds offer a viable alternative to solvent-containing, high-MVT systems and laminates used to produce waterproof breathable fabrics. The incorporation of hydrophilic pendent PEO groups into the polyurethane backbone provides highly breathable compositions when used at the appropriate level and with the proper length. The robust colloidal stability and functionality of these products provide new tools to meet the changing technical demands of the coated fabrics market. The environmentally friendly Permax technology opens a window of opportunity into a broad range of applications that go beyond the textile coating industry.Acknowledgements
The authors would like to thank the organizers and sponsors of the 31st International Waterborne, High-Solids, and Powder Coatings Symposium, 2004, for the opportunity to present this paper, and Noveon, Inc., for the permission to give the presentation. We are also grateful to Sharon O'Brien and Dennis Malaba for their synthesis support and Valerie Woodward for the microscopy work.
For more information contact Alex.Lubnin@noveoninc.com.
References
1 To get acquainted with this tricky fellow, please go to: http://www.auburn.edu/~smith01/notes/maxdem.htm2 McCullough, E.A.; Kwon, M.; Shim, H. Meas. Sci. Technol., 14, 1402 (2003).
3 Lubnin, A.; Snow, G.; Varn, R.; Anderle, G. U.S. Patent Application No. 20030195293 (2003).
4 Johnson, L.; Samms, J. J. Coat. Fabrics, 27, 48 (1997).
5 Krishnan, S. WO 94/02526, February 3, 1994.
6 Federal Register, Thursday May 29, 2003-Part II, Environmental Protection Agency 40 CFR, Part 63, National Standards for Hazardous Air Pollutants: Printing, Coating, and Dyeing of Fabrics and other Textiles; Final Rule. http://www.epa.gov/ttn/atw/fabric/fabricpg.html
7 Dieterich, D. Progress in Organic Coatings, 9, 281 (1981).
8 Schutze, D.I. The 8th International Conference on Textile Coating and Laminating, Frankfurt, Germany, Paper 11 (1998).
9 Coogan, R.G.; Vartan-Boghossian, R.; Lapkin, M. U.S. Patent No. 4,992,507 (1991).
10 Noll, K. U.S. Patent No. 3,905,929 (1975).
11 Reiff, H.; Lienert, J.; Nast, R.; Witt, H.; Gupta, P. U.S. Patent No. 3,920,598 (1975).
Standard Test Methods for Coated Fabrics - ASTM D 751-95 section 38-41 Procedure A., American Society for Testing and Materials.
Experimental
PUD Synthesis
Dispersions were synthesized by a prepolymer process. The first stage (prepolymer) was conducted under a blanket of dry nitrogen in a 1-quart paint can (disposable reactor), which was equipped with a temperature-controlled aluminum water jacket and placed on a hot plate. The jacket and the hot plate were both controlled by the same temperature controller. The sequence of monomer addition varied. If isocyanate-reactive compounds were added in stages, then each previous stage was reacted to below the theoretical content of NCO before the next monomers were added. After the prepolymer was fully reacted, it was cooled to a desired temperature and dispersed in water. A defoamer was used if excessive foaming was observed. After mixing for about an hour, the chain extender was gradually added. The residual NCO was consumed by a reaction with water at ~50 ºC.
Fabric Preparation
Dispersions were compounded with the Printrite™ PM thickener available from Noveon in order to achieve sufficient viscosity for knife coating application. Each coated fabric sample was prepared using a 18x10-inch swatch of Style 306A Filament Nylon 6,6 Semi-Dull Taffeta from Testafabrics, Inc. The swatch was mounted and stretched on a pin frame equipped with springs to apply tension to the fabric. A thin coat (~0.15 to 0.20 oz/yard2) of the thickened polyurethane dispersion was applied to the entire available surface of stretched fabric using a floating/tight knife. The entire assembly was placed in a circulating air oven at 212 ºF until dry (typically about 5 to 15 minutes). The fabric still mounted on the pin frame was stretched over an elevated glass plate on an aluminum frame. The thickened polyurethane dispersion was applied twice at the 2-millimeter thickness using a Bird applicator. The frame and the fabric were placed again in the 212 ºF oven and dried. The dried coated fabric was removed from the pin frame and dried further for 5 minutes at 300 ºF. The final dried specimen typically had about 0.5 to 1.25 oz/yard2 of dried polyurethane coating.
MVTR Measurement
A 4-oz. Ball Mason jar was filled with demineralized water to within 1/2 inch of the jar's top. The jar mouth was coated with silicone grease. A 3-by-3-inch test specimen (larger than the diameter of the jar mouth) was placed across the greased jar mouth with the coated side facing inside the jar. The test specimen was locked into place across the jar mouth using a screw top lid with a gasket and having a circular opening. The complete assembly was weighed and placed in an upright or upside-down position in a conditioned room (~70 ºF and 50% relative humidity). A fan was used to blow air across the jar at ~500-575 linear feet per minute for 24 hours. After the test, the entire assembly was reweighed and the MVTR was calculated in grams of water lost per square meter of surface exposed to water vapor per 24 hours (gram/m2/day).
Hydrostatic Resistance
Hydrostatic resistance as a function of wash cycles was measured by a Mullen type tester.12
Swell Ratio
Swell ratio was measured as the weight of the polymer specimen after soaking in DM water for three days divided by the weight of the dry sample, expressed in percent.
Total Solids Content
Total solids content (T.S.) was measured by a Moisture/Solids Analyzer LabWare 9000™ (CEM Corporation).
pH Measurements
pH measurements were taken using Acumet Basic pH Meter (Fisher Scientific).
Brookfield Viscosity
Brookfield viscosity (B.V.) testing was performed using a Brookfield RV viscometer and spindles #3 to #6 (depending on viscosity) at 20 rpm and ~77 ºF.
Particle Size and Distribution
Particle size and distribution measurements of the dispersions were obtained by a Submicron Particle Sizer AutodilutePAT Model 370 (NICOMP Particle Sizing Systems).
Tensile Property Measurements
Films were prepared by casting dispersions on Mylar® film using a 10-mil drawdown bar. The films were allowed to air dry thoroughly at room temperature and then at 300 ºF for three minutes. The Mylar film with dried film on it was cut into 1-inch-wide strips and the cast film was removed from the Mylar film. Tensile strength, elongation and modulus tests were taken on an Instron.
Looking for a reprint of this article?
From high-res PDFs to custom plaques, order your copy today!