Wollastonite A Versatile Functional Filler
Wollastonite is a naturally occurring calcium silicate mineral with the molecular formula CaSiO3. Known as tabular spar in the late 1700s, it was named wollastonite in 1822 to honor the English chemist and natural philosopher William Hyde Wollaston. Wollastonite remained little more than a curiosity, however, until its first commercial use in 1933, when it was mined briefly in California as a raw material for the production of mineral wool. Wollastonite did not become an important industrial mineral until the 1950s, with growing appreciation of its value in coatings and ceramics during the post-war building boom.
Wollastonite is unique among nonmetallic industrial minerals for its combination of white color, acicular (needle-like) crystal shape and alkaline pH. Commercial grades are typically high in purity because most ores must be beneficiated by wet processing and/or high-intensity magnetic separation to remove accessory minerals. The accessory minerals most commonly associated with wollastonite are quartz, calcite (calcium carbonate), diopside (calcium magnesium silicate), garnet (calcium iron silicate, calcium aluminum silicate) and prehnite (calcium aluminum silicate). These impurities are a natural consequence of the formation of wollastonite through contact metamorphism of silica and limestone according to the following general formula.
CaCO3 + SiO2 = CaSiO3 + CO2<
calcite quartz wollastonite carbon dioxide
The heat that drove the wollastonite reaction in commercially exploited deposits was supplied by magma, which also introduced the aluminum, magnesium and iron for formation of the accessory minerals.
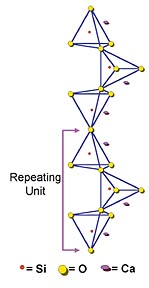
Structure and Properties
Figure 1 shows that the structure of wollastonite is characterized by the repeating, twisted, three silica tetrahedra unit. The chains formed by these silica tetrahedra are connected side by side through calcium in octahedral coordination. Because of this chain structure, wollastonite grows as acicular crystals and will preserve acicularity on cleavage. The high density of the silica chains accounts for the mineral's hardness, Mohs 4.5-5. In nature, trace to minor amounts of calcium may be replaced by iron, magnesium, manganese, aluminum, potassium and sodium. Loss on ignition, the weight of volatiles lost at 1000oC, is very low for pure wollastonite. Commercial products have ignition losses from about 0.5%-2.0%, depending primarily on the content of residual calcite.
Wollastonite surfaces in contact with water will hydrolyze to form calcium hydroxide, which accounts for the alkalinity of wollastonite slurries. Wollastonite is subject to dissociation by mineral acids, especially hydrochloric acid, and certain organic acids (formic, acetic, citric, lactic). Incomplete acid dissolution forms a silica gel. Wollastonite has a strong buffering effect in acid solutions due to the release of calcium ion.
Wollastonite Grades
The filler uses for wollastonite are dictated primarily by the length of the wollastonite needles in the ore and the extent to which this shape is preserved during grinding of the finished products. The acicular nature of wollastonite is measured in terms of aspect ratio - the ratio of particle length to width. The primary classification of wollastonite products is based on particle size and shape.The processing of wollastonite into commercial grades often requires beneficiation to reduce or remove unwanted minerals. The ore is first crushed and milled sufficiently to segregate mineral phases. Garnet and diopside, common discoloring components of wollastonite ores, are weakly magnetic and can be removed with high intensity magnetic separators. Calcite must be removed by flotation.
Powder grades of wollastonite are milled to a low aspect ratio (3:1-5:1). They are produced in impact mills, usually pebble or ball mills, that effectively break the mineral needles widthwise. Standard fine-ground filler grades are 325 mesh, 400 mesh and 10 micron nominal top size.
High aspect ratio (HAR) wollastonite grades are carefully attrition milled to ensure that the very fine, needle-like particles are preserved and recovered. This is accomplished with air mills or hammer mills. Low aspect ratio fines are minimized, as necessary, by air separation. HAR products have aspect ratios in the 12:1 to 20:1 range. Various grades are available with average needle lengths ranging from 200 micrometers in long-needle products, down to 20 micrometers in micro-needle products. Figure 2 is a photomicrograph of a micro-needle HAR wollastonite.
Both powder and HAR grades of wollastonite are readily available with silane and organosilicone treatments to improve compatibility with organic matrices. These grades are treated in blenders that provide uniform contact of the mineral with the concentrated liquid treatment. This is followed by drying at elevated temperatures to ensure a complete reaction and to remove the alcohol byproduct.
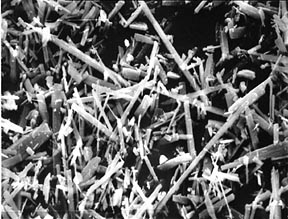
Evolution of a Functional Filler Pigment
Wollastonite was investigated as a functional filler for coatings as far back as the mid-1940s. In 1950, wollastonite was cited as a filler in coatings. Attractive properties of the mineral then included white color, moderate hardness, and a high natural brightness.1In 1979, a review of wollastonite and its suitability in coatings systems noted that this mineral had been important for over twenty-five years as an "extender pigment which contributes to rust resistance, exterior durability, and color retention."2 The article described a study comparing wollastonite to a variety of extenders in both interior and exterior latex paints. Also reported was the use of wollastonite in an acrylic alkyd modified latex paint containing zinc oxide. Using a 400 mesh wollastonite, the zinc oxide was stabilized without a high dispersant demand. Two benefits noted were efficient raw material cost management and better package stability. The author concluded, after comparing extenders in many types of latex paints, that wollastonite offered the following.
- Versatility based on its acicular shape for low sheen, film strength, and overall durability
- Moderate oil absorption for higher PVC contribution
- High dry brightness for cleaner tints
- Moderate hardness for mar and scrub resistance
- Alkaline pH, likely to become one of wollastonite's most important properties, requiring less ammonia and improving rust and mildew resistance
More recently, work was reported on the use of wollastonite surface-treated with reactive silanes, such as amino and epoxy functional types, in corrosion resistant coatings. In this article the role of wollastonite as a "synergist" with inhibitive pigments was described.4 While the mechanism is not fully known, numerous authors have described the synergistic behavior of wollastonite with the majority of primary inhibitive pigments. What is known is that when used in combination with inhibitors, wollastonite, especially the surface-treated grades, enables the inhibitive pigment to be more effective for overall corrosion protection than when the pigment is used by itself. One author, in a study focusing on waterborne epoxy primers and anti-corrosive pigments, reached the following conclusions regarding treated wollastonite and its performance:5
-
PVCs in the range of 38-45%, and a PVC/CPVC ratio of 0.6-0.9, showed the best overall coating performance when the extender package included 150 lbs/100 gal of treated wollastonite and the anti-corrosive pigment level was 100 lbs/100 gal.
- Recoatability was excellent.
- Long-term salt spray and humidity resistance expectations were met.
- Keeping PVC at >38% inhibits blistering and flash rusting.
Chromate and phosphate pigments are being developed to improve the effectiveness of inhibitive metal primers, especially in wash primers, aircraft primers, and automotive primers. To completely replace the performance of hexavalent chromium remains a challenge, particularly at low loadings. Because strontium chromate remains, for many systems, the inhibitor of choice, the formulator looks to wollastonite to reduce the amount of strontium chromate used, without sacrificing corrosion protection. Further, zinc phosphate has been used to help offset dependence upon strontium chromate, and over the last 30 years has become the leading nontoxic inhibitive pigment. For instance, a surface-treated wollastonite has been successfully used to improve the performance of zinc phosphate in alkyds.6
In barrier-type formulations where corrosion resistance is achieved through means other than inhibitive pigments, surface-treated wollastonite has been shown to be effective on its own. Because the protection afforded by these types of systems is more related to controlling ionic permeability and chemical reactivity of the binder with the filler surface, a working hypothesis is that surface-treated wollastonite contributes to performance with its acicular shape and via the chemical bond occurring between the surface treatment and the binder.7
Further, wollastonite's acid solubility can be controlled by proper selection of the surface treatment chemistry. By carefully matching the chemistry to the inhibitive pigment and binder system, the contribution of wollastonite to passivation can be maintained, and the synergistic relationship can be maximized.
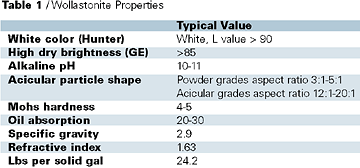
A Versatile Filler
In addition to their use for corrosion control, providing resistance to flash and early rust, high whiteness powder and HAR grades of wollastonite are used in architectural, industrial and marine coatings to ensure film integrity when exposed to physical and environmental assault. The acicular shape of wollastonite particles, even at low aspect ratios, reinforces coating films, providing both durability and flexibility, plus superior scrub and abrasion resistance. HAR wollastonite grades are used in thick build systems - asphalt coatings, coal tar coatings, texture coatings and block fillers - for mechanical strength and durability. Wollastonite is also used in epoxy powder coatings because its low binder demand promotes smooth flow (eliminating orange peel), water resistance, improved adhesion and good chip resistance. Coarser, more acicular grades are used in primers because they provide a micro-textured surface with good tooth for finish coats. In decorative powder coatings, 10 micron and 400 mesh wollastonites are used to provide a smooth satin finish.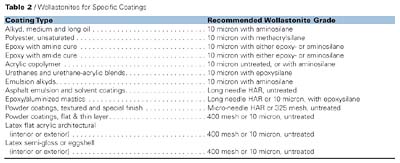
For convenient reference, wollastonite's basic properties of interest to the coatings formulator are summarized in Table 1. The types of coatings that benefit from wollastonite, along with the recommended grades of wollastonite, are listed in Table 2. The properties of wollastonite and their contribution to coatings films are given in Table 3.
For more information on wollastonite, contact RT Vanderbilt Co. Inc., phone 800/243.6064; fax 203/853.1452; visit www.rtvanderbilt.com; e-mail pciullo@rtvanderbilt.com or srobinson@rtvanderbilt.com.
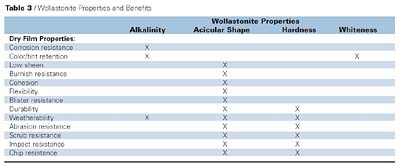
References
1 Ladoo, R.B. "Wollastonite-A New Industrial Mineral." Engineering and Mining J. November 1950.2 Engelhardt, C.L. "Calcium Metasilicate-An Extender Pigment." American Paint & Coatings J. September 10, 1979.
3 Hare, C.H.; Wright, S.T. "An Examination of the Contribution of Functional Extender Pigments to Inhibitive Epoxy Metal Primers." Private publication of Clive H. Hare Inc. 1983.
4 Hare, C.H. "The Evolution of Calcium Metasilicate in Paint and Coatings." Mod. Paint and Coatings. November 1993. Volume 83(12).
5 Jackson, M.A. "An Evaluation of Anti-Corrosive Pigments." J. Protective Coatings & Linings. April 1990.
6 Hare, C.H. "Corrosion Control Using Chromate and Phosphate Pigments." PCI August 1997.
7 Hare, C.H. "Mechanisms of Corrosion Protection with Surface-Treated Wollastonite Pigments." PCI March 1998.
Links
Looking for a reprint of this article?
From high-res PDFs to custom plaques, order your copy today!