

In polymer industries, as well as material science, the use of inorganic fillers becomes more and more important. For many decades, low-cost fillers (e.g. those based on CaCO3, clay, quartz, carbon black) have been used to reduce material costs of monomers or polymers. In addition to this cost-reducing effect, suitable inorganic fillers can provide an improvement of material properties like tensile strength, hardness, abrasion resistance, thermostability, coefficient of expansion and gas-barrier effect. However, the applicability of such fillers, particularly in coatings, is restricted because of some major disadvantages (e.g. loss of transparency, high viscosity build-up, sedimentation). A solution for this problem can be the use of monodisperse nanoscaled SiO2 particles that don't inherently have these drawbacks.
Currently, silica nanoparticles suitable for industrial use are mainly made from silicon tetrachloride by a flame hydrolysis process, followed in many cases by a silane treatment of the SiO2 surface.1 The size of the primary particles of this fumed silica is in the range of 7 to 40 nm, but during the flame hydrolysis much larger agglomerates are formed. These agglomerates are only partially redispersable to the original primary particles, which is the reason for the dramatic viscosity increase and clarity loss of most dispersions containing fumed silica in amounts of more than a few percent. Therefore, the achievable filler load is usually limited below the level desirable from a performance standpoint. Surface modification of the silica with organofunctional alkoxysilanes together with mechanical aftertreatment may help up to a certain level and shows promising results,2,3 but at the expense of significantly increased cost and certain processing inconveniences.
An alternative route to nanoscaled particles is the preparation of colloidal silica sols after the sol-gel method.4 Because of the expensive starting materials and the complicated processing, the utilization of this procedure is limited and of low practical use in the industry.
The most inexpensive SiO2 nanoparticle sources are silica sols prepared from aqueous sodium silicate using ion exchange techniques. Sodium silicate solutions are commodities, and the sols produced thereof provide narrow particle size distributions within a range of 7 to 70 nm. UV-curable silica nanocomposites made from these sols have shown highly improved material properties.5-7 However, to get the silica particles from the aqueous into the hydrophobic organic environment without causing agglomeration is a major challenge. Therefore, until recently, marketed products were only available in a very limited number of solvents and used acrylic monomers, which show numerous incompatibilities when formulated.
hanse chemie recently developed a new and highly efficient methodology of particle synthesis and subsequent organic surface-modification, which delivers versatile silica nanocomposites (SNC) that contain colloidal silica with particle diameters of about 20 nm. Previous investigations revealed that Epoxy-SNC highly enhances the material properties of thermally or UV-cured epoxy resins.8 A new product group of SNC includes a very broad range of acrylic monomers and oligomers (polyester-, epoxy-, urethane- and melamine-acrylates) and offers the possibility to enhance the material performance of radiation-curing acrylate coatings. With silica concentrations of up to 60%, these products are as clear as the starting resins. Small Angle Neutron Scattering (SANS) examinations show that very narrow particle size distributions are characteristic for these products (Figure 1), which proves that they are monodisperse and non agglomerated. This is additionally confirmed by TEM photography (Figure 2).

Nanocomposites
Fig 1&2
By Christof Roscher, Ph.D. / Group Leader of R&D and Technical Service of Nanocomposites/hanse chemie AG, Geesthacht, Germany
Details on Examined Materials
Comparison of Rheological Properties of Fumed Silica and Nanoscaled Silica
In order to verify that colloidal non-agglomerated SiO2 nanoparticles do possess the assumed benefits, investigations were made to show the reduction in viscosity build-up and improvement of scratch and abrasion resistance compared to fumed silica.
For this purpose, the 50% silica-containing Product A was diluted with the base resin (SR 494) to give samples containing different amounts of SiO2. Comparative examples were generated by incorporating commercially available methacrylate-modified fumed silica into the pure binder using a dissolver. The mixture was then dispersed on a pearl mill. With all silica concentrations the obtained formulations were as clear as the starting resins. Figure 3 shows the dependence of the viscosity on the SiO2 content. It is clearly revealed that, in comparison with fumed silica, the fluidity of SNC containing equal amounts of filler is drastically improved (30% fumed silica causes a viscosity increase equivalent to 50% colloidal silica).
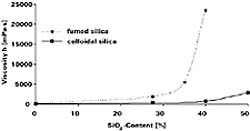
Manual Scratch Resistance
Thin films (50 mm) of the above formulations were prepared on melamine-impregnated paper and polycarbonate by using a doctor-blade coater. Passing these samples (containing 2% of photoinitiator Lucirin TPO-L) at velocities of about 6 m/min through two consecutive medium- pressure Hg lamps (120 W/cm; residual O2 < 200 ppm) proved sufficient to achieve equivalent UV curing. In all cases excellent adhesion was attained, and the presence of silica apparently had no impeding effect on the cure rate.
Already a simple manual scratch test disclosed a distinctly improved scratch resistance. After 60 double rubs with a steel wool, the unfilled coating was totally destroyed. Two samples containing 28% fumed and colloidal silica respectively to some extent already showed an improvement of scratch resistance. However, the incorporation of 50% colloidal silica leads to an improvement otherwise unachievable, and the coating remains virtually untouched (Figure 4).

S-42 Taber Abrasion Resistance
In order to obtain more qualified data, S-42 Taber abrasion resistance of the test panels was determined. The weight loss was recorded after 50 cycles under a 500 g load using CS-0 calibrase wheels covered with S-42 sandpaper. Additionally, the diamond scratch hardness was determined according to DIN 53799. The results demonstrate a significant performance improvement of the SNC samples compared not only with the unmodified SR 494 but also with the fumed-silica-containing formulation (Table 1). The latter result is the more remarkable considering the significantly lower viscosity and the much more convenient processing of the SNC. It was also revealed that the improvement increases parallel to the silica concentration and doesn't reach a maximum. At a level of 50% silica the weight loss decreases from 51 mg to as low as 6 mg, which is equivalent to a reduction of about 88%!
CS-10 Taber Abrasion Resistance
The very aggressive sand paper method suits well for demanding applications like parquet flooring. In order to obtain suitable data for less-demanding applications, a less-severe test was performed, this time using the more-gentle CS-10 rubber wheels. After 100 and 500 abrading cycles under a 500 g load, the resulting haze (difference of gloss before and after the test) was measured using a reflection densitometer (60 deg). The results of both measurements again show a significant performance improvement of the SNC samples (Table 2). At a level of 28% colloidal silica, the haze reduction is equivalent to about 83% (after 100 cycles) and 88% (after 500 cycles). Surprisingly, this time the improvement reaches a maximum at this filler concentration and isn't further increased by incorporating higher amounts of silica.
Universal Suitability of Nanoscaled Silica
The type of binder present primarily determines coating properties, and a reinforcement strategy must therefore harmonize with the widest possible variety of systems. To demonstrate the versatility and universal suitability of nanoscaled silica, SNC from a selection of different UV resins were tested to comprise a wide parameter range concerning functionality, reactivity and viscosity as well as, with regard to the unmodified samples, network density, hardness and flexibility.The various varnishes (containing 4% of photoinitiator Darocur 1173) were applied to black glass plates at a dry-film thickness of 90 µm and cured under a 160 W/cm mercury lamp at a speed of 10 m/min.
For determination of the scratch resistance of the different samples, a scrub-resistance tester for emulsion paints (DIN 53 778) was used. The standard brush was replaced with a Scotch-Brite pad (type 07442), which was loaded with a 350 g weight and pulled 200 times across the coated test panels. Once more the resulting haze was measured. Elasticity and hardness examinations of the coatings were also carried out. Table 3 shows the results with five different acrylate systems. It is clearly revealed that the colloidal silica substantially improves the scratch resistance regardless of the chemical nature of the binder and its intrinsic elasticity and hardness. The microhardness (DIN 50 359) is also significantly increased while the pendulum hardness (DIN 53 157) surprisingly remains unaffected.
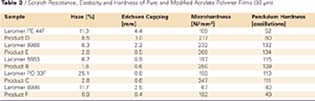
Summary
A new method of particle synthesis and subsequent organic surface-modification delivers silica nanocomposites consisting of monodisperse, non-agglomerated SiO2 nanoparticles and radiation-curing resins. The manufacturing process is very flexible and allows the production of nanocomposites based on an extensive range of acrylic monomers and oligomers as well as cationic curing epoxies. The resulting products are stable, highly transparent and exhibit a remarkably low viscosity despite silica loads of up to 60%. On the basis of the results presented, it has been shown that the scratch and abrasion resistance of UV-cured SNC are substantially improved, making these nanocomposites a useful and versatile raw material source suited for all kinds of high-performance applications.Acknowledgement
The author thanks Dr. H. J. Gl?l from the IOM Leipzig and Mr. M. Biehler and Mr. K. Menzel from BASF for their efficient work on the coatings. We gratefully acknowledge the assistance of GKSS Geesthacht for SANS analysis an ISC W?rzburg for TEM.For more information, contact Anderson & Associates, LLC, hanse chemie's North American sales agent, 86 Summit Ave., Summit, NJ 07901; phone 800/678.3024; e-mail info@Andisil.com.
This paper was presented at the 7th Nurnberg Congress, European Coatings Show, April 2003, Nurnberg, Germany.
References
1 Frahn, S.; Ettlinger, M.; Ladwig, T.; Mendel, W. Eur.Coatings J. 2001, 3, 98.2 Gl¿l, H. J.; Bauer, F.; Ernst, H.; Findeisen, M.; Hartmann, E.; Langguth, H.; Mehnert, R.; Schubert, R. Macromol.Chem. Phys. 2000, 201, 2765.
3 Frahn, S.; Valter, F.; Leger, G. Conference Proceedings 6th N¿rnberg Congress 2001, 145.
4 Corriu, R.; Leclercq, D. Angew. Chem. 1996, 108, 1524.
5 Lewis, L. N.; Katsamberis, D. J. Appl. Polym. Sci. 1991, 42, 1551.
6 Mistra, M. Guest,G.; Tilley, M.; Surf. Coat. Inter. 1998, 12, 594.
7 Vu, C.; LaFert¿O.; Eranian, A. Eur. Coatings J. 2002, 1-2, 64.
Adebahr, T.; Roscher, C.; Adam, J. Eur. Coatings J. 2001, 4,144.