The best alternative to the spoken word would be to compile your own record of equipment performances via running lab tests on every available choice of media mill, followed by in-house, long-term testing for complete confidence in your final choice. Although thorough, this method is both very time consuming and costly in material, travel and installation expenses.
For these reasons, it is necessary to eliminate possible vendors and predict results through knowledge of both the theoretical side and practical side of wet grinding and media mill technology. Knowing what to expect and what not to expect before spending money on comparison testing will increase your confidence and reduce your time spent on making a decision.
The First Step
The first step of mill comparison is obtaining readily available product brochures from each considered vendor. The literature should clearly explain the technology of the mill and also display a table of model sizes including chamber volumes and motor sizes. Most vendors will be more than happy to visit your facility to give a full presentation on their technology. This is of no cost to the customer other than about an hour of their time.
After comparing notes from each manufacturer, it will be easy to narrow down to three or four vendors if you know what to look for and what questions to ask. You can then enter product trials with confidence and a reasonable expectation of performance. Having fulfilled your expectations, a final choice should not be too difficult.

Energy Density
Grinding is directly related to energy absorbed in the mill chamber. Simply, the more energy you can input into a batch, the more grinding action is achieved. The heat produced from grinding is removed through the internal chamber surface via cooling water. Every mill is limited in cooling surface area and therefore limited to the maximum amount of energy that can be used for grinding. This dictates the installed motor power for a mill. The nominal energy density of a mill is the available energy per volume of milling chamber.
Energy Density = Installed Motor Power (kW)
Mill Chamber Volume (liters)
Since cooling capacity dictates the installed motor power, by comparing the energy density of each media mill type, it is clear to see which ones have the most cooling capability to allow for the maximum grinding capacity.
Higher energy density is not only beneficial for increased milling capacity, it also allows the grinding of hard particles, which require higher milling energy density for size reduction. The other benefit is that the chamber volumes are much smaller than a lower energy density mill with equivalent installed motor power. The reduced volume can be as much as 10 times smaller. This is a significant saving in grinding media costs.
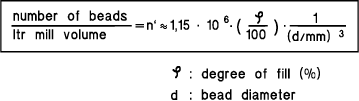
Mass-Specific Energy
Choosing a mill should not be based upon energy density alone. It is even more important to determine how efficient a mill is in turning that energy into particle-reducing mechanical energy. A common measurement for this efficiency is mass-specific energy.The mass-specific energy is the work required to mill a quantity of product to a desired grinding specification. Mass-specific energy can be relayed in a variety of engineering units such as:
- Kilowatt hours per pound,
- Kilowatt hours per kilogram,
- Kilowatt hours per ton,
- Kilowatt hours per metric ton (most common).
The quantity of product is normally expressed in a total slurry weight for coatings and colorant dispersions, and in a dry weight for pigment grinding.
The relationship between mass-specific energy and particle size reduction is illustrated in Figure 1. Figure 1 represents a series of single-pass milling experiments performed on a horizontal mill under fixed media conditions but with varying product flow rates and agitator speeds. The specific energy for each experiment was calculated and plotted versus median particle sizes produced at that grinding condition.
These experiments illustrate the fixed relationship between final particle size and the mass-specific energy of grinding for a given mill and media type. Regardless of flow rate or agitator speed, to achieve a target fineness of grinding, a specific amount of energy is required. Decreasing this energy requirement means having to run at higher milling efficiency. This is achieved by changing the grinding media, process milling method or using a more efficient mill.
The specific energy requirement is the best parameter for choosing the most efficient mill. The mill that works at the lowest specific energy will save you the most time and money over the life of the equipment. The specific energy is ultimately determined through actual product testing, but there are a few indicators to predict which mill will be the most efficient.

Grinding Media Size Effect
The ability to operate a mill with smaller-diameter grinding media greatly increases the mill's efficiency and can overall further increase the quality of product through finer grinding. However, smaller grinding beads are more prone to hydraulic packing due to their decrease in mass per bead. Hydraulic packing occurs when the drag flow from the product pulls the media with the product and ultimately causes media packing around the discharge screen.The grinding beads are the main points of contact where energy from the mill is transferred to the pigment particles, causing reduction in their size. Since the grinding beads are the actual perpetrators of attrition on the pigment particles, by increasing the number of beads or points of contact in the mill one will increase the opportunity for collision and shearing between the bead and the pigment particle.
The number of media beads or contact points per liter can be calculated through the equation in Figure 2. Grinding media diameters with the number of beads per liter at 87% filling load are listed in Table 1. The exponential increase in beads or contacting points as the diameter is reduced gives evidence to higher milling efficiencies.
Figure 3 depicts the effects of media size on grinding times. From this plot, it is shown that a target fineness of 80% < 2 microns is normally achieved in 315 minutes of recirculation milling with 1.0 to 1.4 mm grinding media. By reducing the media diameter to 0.6 to 0.8 mm after 90 minutes, the target grind is achieved in only 150 minutes.
Hydraulic Packing
Hydraulic packing occurs when drag forces on the media due to product flow overcome the natural turbulence of the media, forcing the beads to statically pack around the mill's discharge screen. This phenomenon occurs more easily with lighter and smaller grinding media and also with higher product flow rates and viscosities. Hydraulic packing can occur to a small degree, causing increased mill wear at the discharge end and higher milling temperatures, or can be severe, resulting in immediate pressure buildup followed by mill shutdown.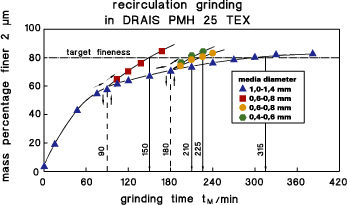
Media Recirculation
The real technology of a media mill is in its ability to prevent hydraulic packing. In horizontal type mills, there are two basic technologies for preventing media packing. One common method is to use reverse-flow pumping discs to force media away from the discharge. This method decreases the effects of hydraulic packing to a degree but at the cost of additional expended energy, resulting in higher milling temperatures. The other, more successful method, uses a blade or rotor in close tolerance to the discharge screen to prevent buildup of media on the screen. Although this method allows higher flow rates than the first method, it tends to cause static packing at higher end milling conditions around the outer shell, thus increasing milling temperatures and wear on the chamber.
The leading technology to prevent packing of beads is now through media recirculation. Media recirculation allows the grinding beads to flow with product towards the discharge under higher product flow rates but then separates the beads from the product through centrifugal force via a special rotor and stator and redirecting the beads back into the beginning of the grinding cycle. This method allows for the highest flow rates using the smallest grinding beads to achieve superior milling efficiency.

Residence Time Distribution
It is of general knowledge that particle size in milling is directly related to the residence time of that particle in the mill. What may not be well understood is how the residence time distribution of particles flowing through the mill effects the particle size distribution. The engineers of DRAISWERKE designed a horizontal mill experiment to study the effects of product flow rate, agitator tip speed and slurry concentration on residence time distribution. The trials were performed on a 10-liter horizontal mill connected to two feed tanks and fitted with a g-ray emitter and scintillation counter to measure the solids concentration at the discharge pipe. All experiments were run using the same media at 80% load.Each experiment began with running pure water from feed tank #1 through the mill. The solids content at the discharge registered 0 mV, which corresponded to 0% solids. Beginning at time zero, a slurry of known concentration from feed tank #2 was introduced into the mill. The outlet concentration was analyzed via the g-ray emitter and scintillation counter over time.
Figure 4, a sample plot of the outlet's change in concentration per time, shows that the outlet of the mill is registering particles at time zero when the slurry is first introduced. This suggests that some particles immediately pass through the mill as others spend significantly more time. For this experiment the flow rate would normally fill the chamber in nine minutes. As seen from the plot, it takes 30 minutes to achieve steady-state concentration in both step functions.
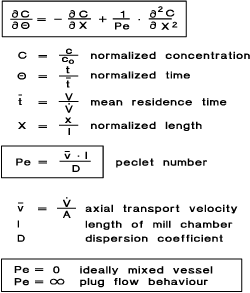
Peclet Number
The data gathered from these experiments can be applied to a simplified mathematical model for axial dispersion in a continuous-flow, stirred vessel as described in Figure 5. The Peclet number is a dimensionless transport parameter used in the dispersion model that is found to be dependent upon the milling parameters.The variables in the designed experiment were the slurry flow rate through the mill, the agitator tip speed and the slurry concentration. The graph in Figure 6 shows the relationship of each of these milling parameters to the Peclet number.
Upon analysis of the particle size distribution for each milling condition, the relationship is confirmed between the Peclet number and the residence time distribution. Figure 7 is a sample of various milling conditions under different Peclet numbers and their corresponding particle size distributions. This plot confirms that high product flow rates yield tighter residence time distributions and thus tighter particle size distributions.

High Flow Rate Efficiency
From studying the residence time distribution of higher flow rates, it can be found that multiple passes at the highest flow rates possible will result in tighter particle size distributions over slow single- or dual-pass operations and thus result in more efficient milling. At high specific-energy-requirement products, high-flow recirculation grinding is ideal for the fastest milling times.
Specific Flow Rate
A good indicator of how suitable a mill is for high-flow recirculation is their nominal specific flow rates. This data can normally be obtained from product literature or through a vendor representative. The specific flow rate is the ratio of the maximum flow rate to the chamber volume. The higher the specific flow rate, the more efficient recirculation grinding is expected.Conclusion
With a better grasp on the theories behind high-performance media mills, the process of determining the best media mill for your application will not be so painful. There are also questions you can ask an equipment supplier on the practical functions of the mill to judge the maintenance and operator friendliness of the unit for further differentiation.For more information, contact Jeffrey Pawar, Draiswerke, Inc., 40 Whitney Rd., Mahwah, NJ 07430; phone 201/847.0600; e-mail salesinfo@draiswerke-inc.com;or visit www.draiswerke-inc.com.
References
1. Dr. Stehr, Norbert (1984), Residence Time Distribution in a Stirred Ball Mill and Their Effect on Comminution", DRAIS News Vol. 1, No. 2.
Single-Pass Versus Recirculation Grinding Processes
Start from premix tank and pass through the mill into a finished tank. Flow rates are generally slow enough to achieve target fineness in one pass. For multiple pass, the finished tank is manually positioned into the premix tank position. Two to three passes maximum. For low specific-energy requirements.
Start from one tank and mill one complete pass into another tank. By opening and closing valves, the second tank is passed through the mill back to the first tank. Repeat pendulum passes until target fineness is achieved. For medium to high specific-energy requirements.
Start from premix tank and slow pass through two mills in series into a finished tank. Generally the first mill uses larger media than the second for breaking down large agglomerates. This method is for low to medium specific-energy requirements.
Start from mixing tank and pass through the mill and back into mixing tank. Requires ideally mixed tank. Ideal for high specific energy requirements and high recirculation flow rates. A minimum of 5 to 10 theoretical tank turnovers is required to prevent unmilled particles left in tank.