Blocked Isocyanates and Their Usage in Plastic Coatings Applications
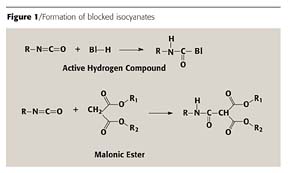
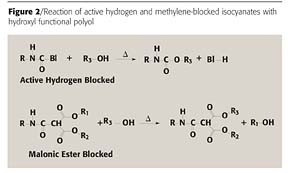


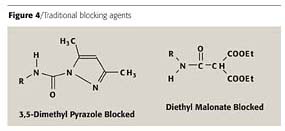

Experimental
A series of three clearcoats were prepared using the two developmental blocking agents as well as a DMP blocked system as the control. All systems contained 0.5% solids on resin solids of a standard tin catalyst and were crosslinked with the same acrylic/polyester polyol blend. These clearcoats were then sprayed to a dry film build of 40-50 mm over an olefin-based substrate to allow removal of free films after curing. Each clearcoat was then subjected to cures of 110-150 deg C in 5 deg C increments. Stress/strain curves and gel content were then collected on the free films.
Gel Content
Free films of approximately one-inch square were placed in a pre-weighed, 100-mesh stainless steel net screen and re-weighed (Photo 1). The free-film-containing screens were then placed in a heated glass flask and refluxed with acetone at 60 deg C for 7 hours (Photo 2). The screens were removed from the acetone, rinsed and dried for 16 hours at 40 deg C before being re-weighed. The difference in weight of the free film after refluxing from the original weight was then used to determine the gel content.
Stress/Strain
Free films were acclimated at 74 deg F and 50% relative humidity for 24 hours prior to testing. Test samples were then cut with a 0.5-inches-wide by 5-inches-long straight die. Films were pulled at a rate of 2 inches/minute. Tensile testing was conducted on an Instron 4444 tensile tester from Instron Corp.
Results and Discussion
The results of gel content testing are shown in Figure 5. As a system undergoes crosslinking, the amount of extractable material decreases and the gel content increases. The maximum gel content expected for these clearcoat systems is approximately 98% as they all contain around 2% of extractable additives such as catalyst and flow aids. The DMP blocked control system does not approach these maximum values until temperatures greater than 125 deg C, and performs poorly at temperatures of 120 deg C or less. The DIPA blocked system gave results very similar to those seen for DMP. However, the BEBA blocked system appears to perform much better at lower temperatures and reaches near maximum levels at just above 120 deg C.
Similar trends can be seen in the results of stress/strain testing. As a system undergoes crosslinking, its tensile strength increases and its elongation will decrease. These results are shown in Figures 6 and 7. The DMP blocked control system does not reach maximum tensile strength or minimum elongation values until 135 deg C. The DIPA blocked system again appears very similar to the control in tensile strength development, but reaches minimum elongation values at a slightly lower temperature. However, this testing again shows improved crosslinking with the BEBA blocked system. Minimum elongation values are now seen at 120 deg C, while tensile strength values obtained at 125 deg C are of a level not seen by the DMP blocked system until 135 deg C.
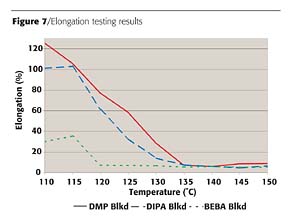
The performance of the developmental blocking agents, including BEBA, drops dramatically below 120 deg C, indicating they would not perform well in underbake conditions. The reaction rates of the systems in this study have potential to be improved by several means. Hydroxyl functional co-reactants will have improved rates of reaction when primary functional groups are present rather than secondary or tertiary groups.3 Also, the choice of catalyst and the level in which it is added can have an effect on reaction rate. Optimization of these parameters may improve reaction in underbake conditions.
Conclusions
Two developmental blocking agents were tested in clearcoat formulations for improved low temperature reaction. Of these two blocking agents, only the BEBA blocked isocyanate approaches a fully cured state by 120 deg C. The DIPA blocked isocyanate gives results similar to the DMP control, requiring temperatures of 130-135 deg C to reach the same cure level. Both developmental blocking agents form urethane linkages when reacted and are expected to give better overall performance than ester linkage forming DEM blocked isocyanates. By optimizing reaction partners and both catalyst type and level, reaction temperatures potentially could be reduced further, improving underbake condition results as well as general performance at any given temperature. In addition, future identification of new classes of blocking agents may continue to reduce required reaction temperatures for 1K polyurethane coatings. A continued reduction in reaction temperature of blocked isocyanates will facilitate their future usage on temperature-sensitive plastics.Acknowledgements
The author would like to give special thanks to Julie Operchal and Keith Bauman for preparing films and testing results and also thank Lanny Venham and Mike Jeffries for preparing the resins used in this study.
References
1 Ryntz, R. Adhesion to Plastics; Molding and Paintability. Global Press, p. 12, 1998.
2 Wicks, Z.; F. Jones; S. Pappas. Organic Coatings: Science and Technology, Volume II. John Wiley & Sons, Inc., p. 143, 1992.
3 Wicks, Z.; F. Jones; S. Pappas. Organic Coatings: Science and Technology, Volume I. John Wiley & Sons, Inc., p. 189, 1992.
Looking for a reprint of this article?
From high-res PDFs to custom plaques, order your copy today!