Environment-Friendly Polymeric Binders

Polymeric binders are valuable raw materials in coatings and in the construction industry. Final product properties strongly depend on the type and nature of the applied binder. Because of environmental concerns, powder coatings, 100% UV/EB curing systems and waterborne polymer dispersions are being substituted for traditional resins used in solventborne coatings. Development in the area of polymeric dispersions and radiation-curable materials is the main subject of this paper. Application of the heterogeneous polymers usually described as core-shell structures in paints and cement compositions is discussed. In the radiation-curing area, waterborne systems based on acrylic polymers are presented. A potential application as a binder of radiation-curable heterogeneous polymer dispersions is also highlighted.
Introduction
Traditional coating systems were based mainly on alkyd and alkyd-modified resins used in solventborne formulations or on simple binders like lime, natural oils or glue.1,2 With time, other types of chemistries were involved and polyester, polyurethane, acrylic and condensation resins were applied as a binder in solventborne formulations.3 The properties of these well-formulated paints are excellent, but a remarkable solvent content was responsible for paint flammability and sometimes toxicity.Environmental concern was the main reason to develop other systems like powder coatings, high-solids, radiation-curable and waterborne alternatives to mature solventborne (S/B) coatings. The present paper is focused on radiation-curable and waterborne coatings, so high-solids and powder coatings will not be discussed here.
In the beginning of the 1960s, waterborne systems (W/B) based on polymer dispersions, mainly polyvinyl acetate (PVA) homopolymers or copolymers, were used for interior and exterior decorative paints. Waterborne technology allowed the reduction of the organic solvent content in the paint formulation but, especially at the beginning, some properties of W/B products were inferior to the properties of solventborne types (Table 1). The waterborne market was developing very fast, and polymer dispersions of different chemistry types were created and introduced to various waterborne coating and cementitious systems.4 Acrylic copolymers are used very commonly because of their versatility and tailor-made properties depending on the monomer content and polymerization technique.5
Polymeric dispersions may be used as: a conventional binder drying in the physical process during evaporation; a binder crosslinking by cation-anion interaction between acid groups and bivalent cations; a binder crosslinking in the reaction with hardener molecules; or as a reactive polymeric or oligomeric material prone to crosslinking under the influence of UV/EB radiation. Acrylate chemistry is widely used both in 100%-solid radiation-curable systems as well in the waterborne variety.6,7

UV/EB-Curing Systems
Radiation curing is a very fast chemical process using high-energy sources like ultraviolet (UV) or electron beam (EB) to transform relatively low-molecular-weight liquid formulations into high-molecular-weight, crosslinked, solid and non-tacky coatings. This process is virtually 100% free of VOC emissions and is a very promising alternative to industrial solventborne technology.6-9 The radiation curing market is developing quickly; annual growth rate is estimated between 7-11%. Main advantages and disadvantages of classic and waterborne radcure technology are listed in Table 2. In summary, efficiency, environmental and economic aspects are the main attributes of radiation-curable systems.The first industrially applied UV process gained about 90% of the radcure market. It used different ultraviolet and visible wavelengths absorbed by photoinitiators to produce the free radicals necessary for polymerization initiation. Recently, UV LED technology, offering a narrow UV-wavelength spectrum without harmful shortwave fractions, and UV DC power lamps, yielding higher double bond conversion, were introduced.10
The electron beam process uses high-energy electrons capable of starting an effective free-radical polymerization without need of a photoinitiator. For that reason, EB formulations are about 5% less expensive compared to their UV-cured counterpart.11 Because of high investment costs, EB curing is restricted to a small number of big scale end-users.12
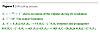
UV-Curing Mechanisms
In industrial practice there are two UV-curing processes applied. The first one is well known and widely accepted – the free radical derived from the initiator reacts with monomers, oligomers or macromers present in the radcure formulation (Figure 1). The process is difficult for highly pigmented coatings and three-dimensional (3-D) substrates and is sensitive to inhibition by atmospheric oxygen. These weaknesses may be overcome by application of special pigments transparent to UV light, a system of lights and mirrors and a curing chamber filled with relatively heavy carbon dioxide to replace oxygen from the reaction spot.13 For the latter case, radicals involved in the initiation of the curing process are not intercepted by oxygen to form unreactive molecules and stop the polymerization reaction.

Conventional crosslinking by Michael reaction is possible in the case of acetoacetylates containing an active methylene group and acrylates in the presence of strong bases like potassium hydroxide.14,15 To use such a mechanism (Michael reaction) in the UV-curing process, a compound that decomposes with a base formation during irradiation should be found.
Unfortunately, the alkylamine produced easily from an aminocobalt (III) complex upon exposure to UV light is too weak a nucleophile to catalyze the Michael reaction.15,16 For example, the pKa of triethylamine is 10.7, while the pKa of sodium hydroxide is about 14. Tetramethylguanidine (TMG), with a pKa of 13.6, is a very efficient catalyst according to the literature data.15
Searching for molecules yielding TMG or other strong bases under UV irradiation could open some practical possibilities for the anionic mechanism, which is limited now only to a few examples. A photolatent precursor of tetramethylguanidine is described in the patent literature.17

Other Possibilities for UV/EB Systems
During the last 10 years, powder and waterborne radiation-curable systems were applied successfully in industrial practice. In the case of powder coatings, the melted coat is crosslinked because of the polymerization reaction upon UV or EB irradiation. In industrial applications, free radical and, to some extent, cationic mechanisms were used.7,18,20Waterborne radiation-curable systems combine the advantages of both radiation curing and aqueous dispersion technology. Stable aqueous dispersions of macromers form a dry coating after exposure to a radiation source.21,22 Waterborne formulations are much safer because they do not contain reactive diluents, which are a source of health concerns in the typical acrylate radiation-curable compositions. Waterborne systems are used frequently for coating porous substrates like wood, paper or textiles. Advantages of this technology are reduced odor, toxicity, flammability, good sprayability, easier clean-up and viscosity adjustments.
For complicated 3-D applications hybrid cure systems are a good solution. The formulation, upon radiation exposure, undergoes polymerization by two different mechanisms – free radical and cationic. Initially the cationic composition cures rapidly even in shadow places, which are difficult to access in typical UV free-radical curing.23
Monodispersed silica-acrylate nanocomposites and similar silica-epoxide systems could be prepared in a flexible process, yielding a broad range of reactive monomers and oligomers. Obtained reactive silica-binder nanocomposites containing up to 60% of silica may be applied in a radiation-curing process (free radical for acrylates, cationic for epoxides), giving excellent scratch- and abrasion-resistant coatings.24 Nanometric colloidal silica acrylates are used in radiation-curable organic-inorganic high-gloss thin coatings with improved mechanical properties. In the case of small particles (D = 13 nm), coating gloss is maintained even when the load of silica is as high as 25%.25
Polymeric Dispersions
Development of emulsion polymerization techniques and availability of waterborne polymer dispersions made possible the introduction of these valuable materials as binders in the coatings industry. Because of environmental aspects, the application of dispersions is still one of the main challenges in the coatings industry. The variety of available monomers and their combinations allows us to obtain polymers with very different chemical and physical properties that are useful raw materials in paint formulations. The improvement of properties is often obtained through the modification of the basic polymer chain with a small amount of functional monomers. Functional monomers that contain polar groups like carboxylic, sulphonic, hydroxylic or amminic increase the hydrophylic character of the polymer, thus improving its compatibility with inorganic substrates and making possible additional crosslinking by reacting with the hardener molecule. Ionizable groups are also responsible for easy self-dispersing of polymer powders prepared from polymer dispersions.Because of availability, versatility and the moderate cost of monomers, acrylic polymers are very widespread in the coating and adhesives industries. Chemical stability and durability, and the broad range of glass transition temperatures (Tg) make acrylics the polymers of choice for many indoor and outdoor applications.
Polymer properties depend not only on the monomers used, but also on the polymerization technique. For example, dispersions obtained in mini- or micro-emulsion processes are characterized by a narrow diameter distribution and improved stability because of thermodynamics and not kinetics, like in the standard macro-emulsion process, stabilization system.26 Prepared micro-dispersions are typically nano materials with big development potential as novel raw materials for coatings.27 Nanometric dispersions, together with nanoparticles of fillers and pigments, are improving mechanical parameters of coatings without sacrificing adhesion and elasticity.28,29
Mini-emulsion polymerization was successfully applied for the synthesis of aqueous copolymer dispersions containing in the inner-part (core) inorganic particles like calcium carbonate, titanium dioxide, magnetite and other solid nanoparticles.27 Free radical or polyaddition chemistry is used for polymer synthesis.
A different approach is based on the application of ultrasound to incorporate inorganic particles (metals, metal oxides or sulfides) into a colloidal polymeric matrix.30 Prepared composite materials may be used for improved electrical and mechanical coating properties or as antibacterial or catalytical coatings.
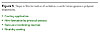
Heterogeneous Copolymer Dispersions
Polymers obtained in a multi-step emulsion poly-merization of different monomer mixtures have been investigated extensively in the last two decades. A multi-step polymerization technique is a powerful tool for polymer structure differentiation and allows preparation of copolymers with the desired balance of physical, mechanical and chemical properties. Different heterogeneous structures like core-shell, inverted core-shell, acorn, raspberry or multi-lobed are described in the literature. From among them, core-shell species are the best-known examples of structured copolymers. Such polymers comprise different polymer parts; for example, an inner part named “core” and an outer part named “shell”. Structured polymers differing in glass transition temperatures (Tg) of core and shell are useful as binders and cement admixtures as aqueous dispersions and prepared redispersible powders.4,5,31-33The differences in core and shell composition influence film forming, as well as mechanical, optical and chemical properties of the polymer. Heterogeneous copolymers containing low-Tg (soft) core and high-Tg (hard) shell with strong ionizable groups, for example sulphonic groups located in the polymer shell, are easily accessible for water and are responsible for improvement of adhesion to inorganic materials. The interaction of acid groups with water also decreases the minimum film-forming temperature (MFFT) due to the water plasticizing effect. Described core-shell copolymers could be easily transformed into redispersible polymer powders. Such copolymers may find application as polymeric powders for special mortars and to some extent as impact modifiers and binders with improved anti-blocking properties.4,31,34
Another concept of core-shell morphology is also used in coating practice – copolymers with soft-shell and hard-core are very efficient binders in low-VOC or VOC-free formulations, in floor varnishes with increased black heel mark-, stain- and abrasion-resistance and in paint formulations with good anti-blocking performance.32
For certain applications coatings have to be very hard and be flexible at the same time. Such properties may be obtained using core-shell polymers with an additional crosslinking process. After formulation application and water removal (by porous substrate or evaporation) a radiation-curing step may be triggered to obtain final properties of the dry coating (Figure 5). Possibly a novel hybrid system may be proposed consisting of structured copolymers with some unsaturations grafted on the polymer shell and reactive in the radiation curing (UV or EB) process. Combination of core-shell and radcure chemistry may allow one to obtain tailor-made formulations and coatings that fulfill the most challenging demands. Such systems, in contrast to highly crosslinked UV-curable classic formulations should have low shrinkage, better adhesion and the required mechanical properties (Table 3).
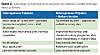
Conclusions
Waterborne and radiation-curable formulations are the most promising environmentally friendly alternatives to traditional coating systems. There are big possibilities for cationic and anionic radiation-curing systems because of toxicological concerns in the case of acrylic reactive diluents used in the classic free-radical process. Waterborne radcure compositions are safe, environmentally preferred alternatives to classic 100% radiation-curable systems.A multi-stage polymerization technique is a useful tool for preparation of tailor-made copolymer dispersions. By use of this method low Tg dispersions and subsequently redispersible powders may be produced. Obtained polymers are very useful binders for zero-VOC paints and flexible mortars.
A hybrid system consisting of a core-shell dispersion with an unsaturation moiety that is reactive in the radiation-curing process may improve the final coating properties without the shrinkage observed in free-radical acrylic systems.
References
1 Umińska, E.; Umiński, M. Farby i malowanie, leksykon
najwazniejszych terminów, Rynek Chemiczny, XVII – XX, No. 1 (2002).
2 Graystone, J.A. Traditional
paints in a modern context, Surface Coatings International Part B:
Coatings Transactions, 85 B2, 159 – 164, June 2002.
3 Lambourne,
R. (Ed.), Paint and surface coatings, Ellis Horwood Ltd
Publishers, Chichester 1987.
4 Umiński, M.; Saija, L.M. Synthesis and application of phase – separated acrylic
copolymer dispersions, Paint & Coatings Industry, 82 – 88, June 2004.
5 Saija, L.M.; Umiński, M. Synthesis and characterization of core – shell acid
functionalized polyacrylate dispersions, Surface Coatings
International Part B: Coatings Transactions 85, B2, 149 – 153, June 2002.
6 Umiński, M.; Saija, L.M. Acrylic monomers for radiation curing, Surface Coatings
International, 78(6), 244 – 249, June 1995.
7 Umiński, M. Waterborne
UV/EB curing systems, Pigment & Resin Technology, 26(3), 149 – 152 (1997).
8 Fisher, W.; Weikard, J.; Lühmann, E.; Fäcke, T. UV – curing urethane acrylates in the spotlight in Synthetic resins – celebrating a century, European
Coatings Journal, 10 – 12, No. 11 (2003).
9 Decker, C. Linked
by light,
European Coatings Journal, 28 – 32, No. 7 – 8 (2005).
10 Meine, D. Latest
from radiation curing, European Coatings Journal, 88 – 90, No. 9 (2004).
11 Connolly, E.; Mulach, R.; Yoshikawa, S. Radiation curable coatings, Specialty Chemicals SRI
International, January 1990.
12 IAL Consultants Ltd, An
Overview of the European radiation curing market, London, December 1990.
13 Beck, E. Into
the third dimension, European Coatings Journal, 32 – 39, no. 4 (2006).
14 Del Rector, F.; Blount, W.W.; Leonard, D.R. Applications for acetoacetyl chemistry in thermoset
coatings,
Journal of Coatings Technology, 31 – 37, 61, No. 771 (1989).
15 Clemens, R.J.; Del Rector, F. A
comparison of catalysts for crosslinking acetoacetylated resins via the Michael
reaction,
Journal of Coatings Technology, 83 – 91, 61, No. 770 (1989).
16 Kutal, D.; Weit, S.K. New
inorganic photoinitiators for deep – UV resist materials, Journal of Coatings
Technology, 63 – 67, 62, No. 786 (1990).
17 Stanssens, W.A.D.;
Jansen, J.F.G.A. Anionic
photocatalyst, US Pat. 6124371 (1998).
18 Dietliker, K.; Misteli, K.; Jung, T.; Contich,P.; Benkhoff, J.;
Sitzmann, E. Novel chemistry for UV
coatings,
European Coatings Journal, 20 – 24, No. 10 (2005).
19 Dogan, N.; Klinkenberg, H.; Reinerie, L.; Ruigrok, D.; Wijnands,
P. Finishing in the fast lane, European Coatings
Journal, 36 – 40, No. 11 (2005).
20 Knoblauch, M.
To a brighter future, Polymers Paint Colour Journal, 24 – 26, February
2006.
21 Ravijst, J.P. Can
water-based resins be used in radiation cure applications?, Polymers Paint Colour Journal, 7 – 12, October 1995.
22 Mestach, D.; Twene, D.R. Keeping
the weight up, European Coatings Journal, 126 – 131, No. 4 (2005).
23 Bloch, D.R. Vinyl
ether and epoxide monomers for radiation curing, Modern Paint and
Coatings, 44 – 46, August 1994.
24 Roscher, C. Tiny
particles, huge effect, Paint & Coatings Industry, 46 – 52, October
2003.
25 Vu, C.; LaFerté, O.; Uranian, A. Colloidal silica acrylates use in UV coatings, European Coatings
Journal, 64 – 70, No. 1 – 2 (2002).
26 Bortel, E.; Kochanowski, A.; Witek, E.; Pazdro, M. Nano-metric latexes prepared from mini- and
microemulsion polymerizations, paper presented on the VIIth
conference “Synthesis, application and analysis of aqueous dispersions and
solutions of polymers”, Oct. 9 – 10 (2003), Szczyrk, Poland.
27 Landfester, K. Designing
particles,
European Coatings Journal, 20 – 25, No. 12 (2005).
28 Sawitowski, T.; Schulte, K.; Hellmann, H. Ready for use nano – particle masterbatches improve
scratch resistance and UV stability, European Coatings Journal, 98 – 101, No. 3
(2005).
29 Pilotek, S.; Tabellion, F. Nanoparticles
in coatings,
European Coatings Journal, 170 – 176, No. 4 (2005).
30 Hain,
J.; Pich, A.; Adler, H-J.P Multitalentierte
Nanohybridpartikel, Farbe und Lack, 30 – 34, 112, 12(2006).
31 Saija, L.M.; Umiński, M. Water- redispersible low Tg Acrylic powders for the
modification of hydraulic binder compositions, Journal of Applied
Polymer Science 71, 1781 – 1787 (1999).
32 Garzon, A. Waterborne
meets solventborne, European Coatings Journal, 56 – 64, No. 9 (2003).
33 Hartmann, J. A
versatile core – shell nanoparticle a solution to coating challenges, Paint & Coatings
Industry, 106 – 108, October 2003.
34 Umiński, M.; Saija, L.M. Preparation and characterisation of re-dispersible
acrylic powders, Pigment & Resin Technology, 364 – 370, 32, No. 6
(2003).
This paper was presented at ACT 06 (Advances in Coatings Technology), November 2006, Warsaw, Poland. For more information, contact the author at muminski@wp.pl.
Looking for a reprint of this article?
From high-res PDFs to custom plaques, order your copy today!