Colloidal Microcrystalline Cellulose
Colloidal microcrystalline cellulose (MCC) has been used widely in the food and pharmaceutical industries to suspend dense particles, prevent phase separation and stabilize emulsions. This study demonstrates that colloidal MCC has the same functionalities in waterborne coatings. It significantly improves the in-can shelf stability of waterborne coatings without negatively impacting application properties.
Introduction
Colloidal microcrystalline cellulose (MCC) has been used widely in the food and pharmaceutical industries to suspend dense particles, prevent phase separation and stabilize emulsions. This study demonstrates that colloidal MCC has the same functionalities in waterborne coatings. It significantly improves the in-can shelf stability of waterborne coatings without negatively impacting application properties.
Introduction
Waterborne coating systems are complicated. There are three basic ingredients found in typical coatings: pigments, polymers (also known as binders or resins) and solvents. Each ingredient plays an important role in forming a durable and protective film when the coating is dried.
Pigment dispersion controls hiding, chalking, tint retention, color and sheen uniformity, flexibility, gloss, scrub resistance, color development, corrosion resistance, blistering, touch up and leveling of the coatings. Additives such as dispersants and rheology modifiers are added into the formulation to help disperse the pigments and stabilize the system through thickening and suspending.
Rheology modifiers are important stabilizing agents in waterborne coatings. Although their use level is low, they control the flow of the liquid system and thus affect many coating processes and performance. Rheology-controlled processes in coatings technology are stirring, mixing, pigment dispersion, pouring, pumping, coating application, spreading, sagging, leveling, penetration into porous substrates, and pigment settling. Rheology modifiers also affect coating performance such as storage stability, color acceptance, film build, hiding powder, spatter resistance, scrub resistance, water resistance, etc. Since all rheology modifiers thicken the system, and the thickening agent more or less modifies the rheology of the system, this paper uses both terms interchangeably.
Commercially available rheology modifiers can be divided into two categories: conventional and associative. A conventional rheology modifier thickens the water phase of a coating due to hydrodynamic size (chain entanglement or gelling) and flocculative mechanisms. Examples of conventional thickeners are hydroxyethyl cellulose (HEC), ethylhydroxyethyl cellulose (EHEC), hydroxypropylmethyl cellulose (HPMC), alkali-soluble or swellable emulsions (ASE), biopolymers, and inorganics, such as clays. This class of thickening agents, in general, has the benefit of low cost and convenience in use.
Associative thickeners are water-soluble polymers with hydrophobic groups that interact non-specifically with other coating components to increase viscosity and modify the rheology. They are normally chemically modified polymers, such as hydrophobically modified HEC (HMHEC) or EHEC (HMEHEC), hydrophobically modified ethoxylate urethanes (HEUR), and hydrophobically modified ASE (HASE).
Each type of rheology modifier has its own performance attributes and limitations. For example, modified celluloses such as HEC or HPMEC have been the major products controlling the rheology behavior of acrylic latex paints. However, they negatively affect coating properties such as leveling, spatter resistance and/or gloss development. Hydrophobically modified cellulose (HMHEC) improves spatter resistance properties but has the limitation of biostability. They also tend to absorb onto acrylic emulsions1 or interact with surfactants in the system.2,3 HASE gives good gloss development and easy handling, however, it imparts poor early water resistance and is not alkali-resistant. HEUR overcomes these deficiencies and offers improved flow and leveling, film build, spatter resistance and good sprayability to the paint. The problem is that they convey poor sag resistance, color incompatibility, viscosity drop on tinting and dilution,4 as well as poor storage stability.
This study addresses some of the deficiencies encountered here by using colloidal MCC as a stabilizer and secondary rheology modifier to further stabilize the system. Although the study examines only acrylic gloss paint formulations with HEUR thickening agents as primary rheology modifiers, the application is suitable to other waterborne coating systems with other thickening agents.
Introduction
Waterborne coating systems are complicated. There are three basic ingredients found in typical coatings: pigments, polymers (also known as binders or resins) and solvents. Each ingredient plays an important role in forming a durable and protective film when the coating is dried.
Pigment dispersion controls hiding, chalking, tint retention, color and sheen uniformity, flexibility, gloss, scrub resistance, color development, corrosion resistance, blistering, touch up and leveling of the coatings. Additives such as dispersants and rheology modifiers are added into the formulation to help disperse the pigments and stabilize the system through thickening and suspending.
Rheology modifiers are important stabilizing agents in waterborne coatings. Although their use level is low, they control the flow of the liquid system and thus affect many coating processes and performance. Rheology-controlled processes in coatings technology are stirring, mixing, pigment dispersion, pouring, pumping, coating application, spreading, sagging, leveling, penetration into porous substrates, and pigment settling. Rheology modifiers also affect coating performance such as storage stability, color acceptance, film build, hiding powder, spatter resistance, scrub resistance, water resistance, etc. Since all rheology modifiers thicken the system, and the thickening agent more or less modifies the rheology of the system, this paper uses both terms interchangeably.
Commercially available rheology modifiers can be divided into two categories: conventional and associative. A conventional rheology modifier thickens the water phase of a coating due to hydrodynamic size (chain entanglement or gelling) and flocculative mechanisms. Examples of conventional thickeners are hydroxyethyl cellulose (HEC), ethylhydroxyethyl cellulose (EHEC), hydroxypropylmethyl cellulose (HPMC), alkali-soluble or swellable emulsions (ASE), biopolymers, and inorganics, such as clays. This class of thickening agents, in general, has the benefit of low cost and convenience in use.
Associative thickeners are water-soluble polymers with hydrophobic groups that interact non-specifically with other coating components to increase viscosity and modify the rheology. They are normally chemically modified polymers, such as hydrophobically modified HEC (HMHEC) or EHEC (HMEHEC), hydrophobically modified ethoxylate urethanes (HEUR), and hydrophobically modified ASE (HASE).
Each type of rheology modifier has its own performance attributes and limitations. For example, modified celluloses such as HEC or HPMEC have been the major products controlling the rheology behavior of acrylic latex paints. However, they negatively affect coating properties such as leveling, spatter resistance and/or gloss development. Hydrophobically modified cellulose (HMHEC) improves spatter resistance properties but has the limitation of biostability. They also tend to absorb onto acrylic emulsions1 or interact with surfactants in the system.2,3 HASE gives good gloss development and easy handling, however, it imparts poor early water resistance and is not alkali-resistant. HEUR overcomes these deficiencies and offers improved flow and leveling, film build, spatter resistance and good sprayability to the paint. The problem is that they convey poor sag resistance, color incompatibility, viscosity drop on tinting and dilution,4 as well as poor storage stability.
This study addresses some of the deficiencies encountered here by using colloidal MCC as a stabilizer and secondary rheology modifier to further stabilize the system. Although the study examines only acrylic gloss paint formulations with HEUR thickening agents as primary rheology modifiers, the application is suitable to other waterborne coating systems with other thickening agents.

Manufacturing process of MCC.
Colloidal MCC
Microcrystalline cellulose is produced by isolating and disintegrating the crystalline regions of cellulose in an aqueous-based process (Figure 1). It is physiologically inert, odorless and tasteless, making it suitable as a binding agent or disintegrant in the tablet formulations (non-colloidal MCC).
FMC’s patented technology co-processes MCC with various soluble hydrocolloids like carboxylated methylcellulose (CMC), to keep the microcrystals from reaggregating during the drying process. These co-processed MCC are referred to as colloidal MCC because they can be dispersed in water. The hydrocolloids act as barrier dispersants to protect the cellulose from excessive hydrogen bonding during the drying process, and also to aid in the re-dispersion (“activation”) of the dried MCC in the aqueous phase. The technology provides products with specific viscosity, suspension and stabilizing properties, and thus makes them suitable for many industrial applications.
Colloidal MCC was used successfully in waterborne coating systems to stabilize the formulation. It was found to be an excellent stabilizer and dispersing aid, due to its superior suspending functionality and emulsion stabilizing ability. The work described here summarizes some results in acrylic gloss paint systems.
FMC’s patented technology co-processes MCC with various soluble hydrocolloids like carboxylated methylcellulose (CMC), to keep the microcrystals from reaggregating during the drying process. These co-processed MCC are referred to as colloidal MCC because they can be dispersed in water. The hydrocolloids act as barrier dispersants to protect the cellulose from excessive hydrogen bonding during the drying process, and also to aid in the re-dispersion (“activation”) of the dried MCC in the aqueous phase. The technology provides products with specific viscosity, suspension and stabilizing properties, and thus makes them suitable for many industrial applications.
Colloidal MCC was used successfully in waterborne coating systems to stabilize the formulation. It was found to be an excellent stabilizer and dispersing aid, due to its superior suspending functionality and emulsion stabilizing ability. The work described here summarizes some results in acrylic gloss paint systems.
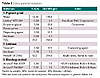
Gloss paint formulation (a).
Experimental: Materials
The materials used in this study and their suppliers are listed in Table 1. There are several grades of colloidal MCC products available from FMC Corporation to provide a wide functionality range with different activation requirements. The one used in the study was Lattice® NTC 80 (colloidal MCC) because it was found to be the most suitable for coating applications.
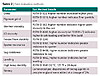
Paint evaluation methods.
Coating Formulations
The general coatings composition used in this study is also summarized in Table 1. The pigment dispersion was prepared with a Cowles-type mixer at high speed until Hegman 7.5 was achieved. ASTM method D 1210 was used to test the Hegman fineness of the grind. Other ingredients were then added at low mixing speed. Evaluation methods are summarized in Table 2.
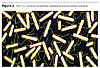
The 3-D network created by colloidal microcrystalline cellulose.
Functional Properties of Colloidal MCC
Properly dispersed colloidal MCC sets up into a 3-dimensional network that imparts stability in the finished product. The network is held together with hydrogen bonding between the particles of MCC and CMC. Unlike many rheology modifiers or thickening agents in the market, this is an insoluble 3-dimensional matrix (Figure 2).
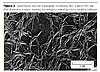
Transmission electron micrograph of colloidal MCC (Lattice NTC 80) after dispersion in water, showing the network created by microcrystalline cellulose.
The transmission electron micrograph (TEM) in Figure 3 clearly shows that the water-dispersed colloidal MCC is a fiber-like submicron sized material. More than 70% of these nano fibers have particle size about or less than 0.2 micron as measured by a light scattering method. They form the physical 3-D network whereby various colloidal grades of MCC provide the following unique functional properties.
Thixotropy: Gels made with colloidal MCC thicken the system and readily break down with shear. When the shear is removed, the gel will reform over time with minimal loss to viscosity.
Thixotropy: Gels made with colloidal MCC thicken the system and readily break down with shear. When the shear is removed, the gel will reform over time with minimal loss to viscosity.
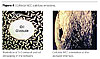
Colloidal MCC stabilizes emulsions.
Emulsion stabilization: Colloidal MCC forms a 3-dimensional network of particles when properly dispersed in water. This network sets up at the oil-water interface and physically prevents the oil globules from coalescing. Figure 4b illustrates the MCC on the interface.
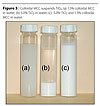
Colloidal MCC suspends TiO2; (a) 1.5% colloidal MCC in water; (b) 5.0% TiO2 in water; (c) 5.0% TiO2 and 1.5% colloidal MCC in water.
Particle suspension: Similar to stabilizing the emulsion, the 3-D network of colloidal MCC also effectively prevents the solid particles from agglomerating or precipitating. It is able to suspend the particles in a low-viscosity system. In Figure 5, the TiO2 was suspended in a colloidal MCC dispersion without any other dispersing aids and the system was stable for at least 28 days at 45 °C.
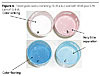
Tinted gloss paints containing HEUR (a&c) and with HEUR plus 0.2% Lattice (b&d).
Use of Colloidal MCC in Acrylic Latex Gloss Paint
HEURs are a rheology modifier class that have been widely accepted in coatings formulation with highly desirable performance. In this study, Colloidal MCC was evaluated as a secondary rheology modifier in the presence of the HEURs. The initial Stormer viscosity of all the formulations was adjusted to about 105 KU.
The first experiment compared the in-can shelf stability of gloss paint with or without Colloidal MCC (0.2% of Lattice® NTC 80). The gloss paint was tinted with red iron oxide (pink) or phthalo blue (blue) tinting base, and placed at 125 ºF (52 ºC) for 2 months. Figure 6 shows that the pink control had color sink (settling), and the blue control had color floating. Both paints with 0.2% Lattice® NTC 80 had very little color separation. It clearly demonstrated that Lattice® NTC 80 is compatible with the HEUR gloss paint system, and the 3-D network effectively suspended and stabilized the color pigments.
The first experiment compared the in-can shelf stability of gloss paint with or without Colloidal MCC (0.2% of Lattice® NTC 80). The gloss paint was tinted with red iron oxide (pink) or phthalo blue (blue) tinting base, and placed at 125 ºF (52 ºC) for 2 months. Figure 6 shows that the pink control had color sink (settling), and the blue control had color floating. Both paints with 0.2% Lattice® NTC 80 had very little color separation. It clearly demonstrated that Lattice® NTC 80 is compatible with the HEUR gloss paint system, and the 3-D network effectively suspended and stabilized the color pigments.
The thickening mechanism of most rheology modifiers involves either use of high molecular weight or structuring interactions due to polymer hydrophobic tails. Surfactants, latex particles, pigment and fillers can all increase the number of possible associate sites for the polymer hydrophobic tails5 and thus change the rheology profile of the system with the addition of the tinting base. Colloidal MCC forms a 3-D network in the system with physical interaction, which minimizes the interaction and rheology profile changes once the network is formed.
During the study, it was found that about 30% less time was needed to grind the pigment dispersion to Hegman 7.5 when Lattice® NTC 80 was used. It may be possible to reduce the dispersant level in the formulation as well.

Colloidal MCC system is stable over a wide range of temperatures.
In contrast to many synthetic rheology modifiers, colloidal MCC is insoluble but dispersible in the aqueous phase. Depending on the colloidal MCC used, it needs to be activated with water through the milling stage, or through high shear agitation. Once activated, the colloidal MCC system is very stable under a wide range of temperature conditions (Figure 7). The insoluble 3-D matrix has the ability to trap both solid particles and liquid particles and protect them from collapse after freeze/thaw cycles. Thus reduced use level of freeze/thaw agents is possible with the use of colloidal MCC. This will help meet the VOC requirements in coatings systems.

Replacement of Acrysol RM 8W with Lattice NTC 80.
Replacement of HEURs by Colloidal MCC in Acrylic Latex Gloss Paint
In further studies, Lattice® NTC 80 was used to replace the Acrysol® RM 8W and RM 2020 in acrylic latex gloss paint. The use level of Lattice® NTC 80 was 0.2% – 0.3% w/w versus two standard Acrysol® control systems. The evaluation results are summarized in Tables 3 and 4.

Replacement of Acrysol RM 2020 with Lattice NTC 80.
The Stormer viscosity data suggests that the colloidal MCC didn’t significantly change the viscosity of the paints. However, it stabilized the system and maintained the viscosity even after aging at elevated temperatures. For example, formula F2705.3 has 14% viscosity reduction after aging at 125 °C for 28 days, while an extra 0.2% Lattice® NTC 80 reduced the viscosity reduction to 6%. The tinting system also had much less separation, similar to the results mentioned before.
Both tables show improved sag with Lattice® NTC 80 (colloidal MCC) @ 0.2% without any negative effect on both leveling and gloss. This may be due to the colloidal MCC’s slow structure rebuild or thixotropy (viscosity recovery), thus allowing good flow-leveling to occur. This phenomenon is very similar to what happens in the organo-clay thickening system.7
Both tables show improved sag with Lattice® NTC 80 (colloidal MCC) @ 0.2% without any negative effect on both leveling and gloss. This may be due to the colloidal MCC’s slow structure rebuild or thixotropy (viscosity recovery), thus allowing good flow-leveling to occur. This phenomenon is very similar to what happens in the organo-clay thickening system.7
These data agree very well with Ma’s studies8 on phase behaviors of latex dispersions. Ma’s research found that the small amount of colloidal MCC can eliminate the phase separation completely from the paint having an optimized Stormer viscosity. This result is in contrast to the use of HEC, HASE or clay as a secondary thickener, which imparts poor flow and leveling, although they can also minimize the phase separation. This may be due to the lower density and the stronger Brownian movement of colloidal MCC particles versus other typical systems. The water insoluble feature of colloidal MCC also contributes improved scrub resistance to paints over other commercial thickeners.
Conclusions
Colloidal MCC forms a 3-D network in water-based systems when fully activated by shear. This network is extremely effective in suspending solid particles and stabilizing emulsions. When it is used in coating systems, colloidal MCC helps to eliminate phase separation and improve storage stability, color compatibility and sag. As a stabilizer and secondary thickener, colloidal MCC provides minimum viscosity to waterborne coating systems at low use level while it significantly improves in-can shelf stability and color tinting ability without viscosity loss. The ability to suspend solid material at low viscosity also makes colloidal MCC especially suitable in the viscosity sensitive systems such as stain applications.
This paper was presented at The Waterborne Symposium, Advances in Intelligent Coatings Design, February 2007, New Orleans, LA. The Symposium is sponsored by The University of Southern Mississippi School of Polymers and High Performance Materials.
Lattice is a trademark of FMC Corporation.
Acrysol, Primal are trademarks of Rohm & Haas Company.
Texanol is a trademark of Eastman Chemical.
Lattice is a trademark of FMC Corporation.
Acrysol, Primal are trademarks of Rohm & Haas Company.
Texanol is a trademark of Eastman Chemical.
Looking for a reprint of this article?
From high-res PDFs to custom plaques, order your copy today!