Fluorosilicone Hybrid Technology
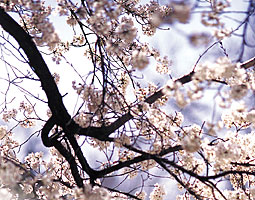
Recent developments in the field of fluorosilicone hybrid coatings and additives have successfully bridged the gap between providing unique performance and satisfying demanding environmental requirements. Fluorosilane anti-fingerprint coatings, hydrosilylation-cure tetrafluoroethylene easy-to-clean coatings, silicone-modified fluoroacrylate textile treatments and fluorosilicone UV-cure resin coatings are some examples of these new sustainable technologies. The synergistic effect of combining fluorine and silicone chemistry results in protective coatings that provide excellent chemical resistance, weatherability, abrasion resistance and thermal stability. Eliminating ozone-depleting chemicals, reducing energy consumption, transforming to water-based systems and resolving PFOA/PFOS regulatory constraints are possible with fluorosilicone hybrid chemistry.
Sustainability Means Good Business
Chemistry brings great value to society. Exciting new materials and technologies, comfort, convenience and economic opportunity are all outcomes of innovation in chemistry. Chemicals are essential to modern life. But how can we balance the need for technological innovation and economic growth with the need to protect human health and the responsibility to provide for future generations? Concern about the impact our lifestyles have on the environment and nonrenewable resources is growing. This concern comes not only from government agencies, but also from industrial users and consumers. Increasing sensitivity to the impact materials have on people and nature is causing proactive responses from industry to ensure the sustainable nature of innovation.
The challenge then becomes the balance of innovation to benefit society and the responsibility to protect our environment. While this may seem like a daunting hurdle, it is, in fact, a very realistic goal. Ensuring our companies have a financially viable future is a natural outcome of maintaining this balance. This was confirmed in a recent international research study conducted for Dow Corning Corporation by the independent market research company Harris Interactive, where attitudes toward sustainability among companies around the world were revealed. One of the key findings was that environmental and sustainability factors have a strong influence on the selection of suppliers in all geographies.(1) This response highlights the need to provide this balance. The result is both sustainable business and a better environment.
![]() |
Figure 1 Click to enlarge |
Energy Conservation
Among the most obvious, and measurable, benefits to the balance of business and the environment are ways to reduce energy usage. Most coating technologies need energy to cure. Heat cure is a common curing method for urethanes, acrylic, fluorine and silicones. Finding ways to reduce the curing temperature or the time to cure will save energy. When doing a coil coating operation, for example, heat is required to cure these coatings; however, the coil itself heats up, absorbing more energy and costing more to operate the coating line.
One of the new fluorosilicone technologies that has recently been developed requires both a lower cure temperature and a shorter cure cycle. The technology uses a hydrosilylation-cure tetrafluoroethylene copolymer (TFEC) in the reaction mechanism described in Figure 1.
This hydrosilylation cure is a common silicone addition cure with the added benefit of having no cure byproduct, which means there is essentially no shrinkage of the cured coating membrane. This cure method requires less energy than traditional melamine or urethane systems and it does not require the engineering systems necessary to capture, scrub, condense or incinerate byproducts. With the Si-H functional crosslinker used in the TFEC hydrosilylation system, the cure mechanism combines breaking the carbon-carbon double bond in the pendant vinyl group on the polymer backbone followed by the crosslink with the Si-H compound.
![]() |
Figure 2 Click to enlarge |
The curing can take place at a temperature as low as 150 ºC. At this temperature this coating takes around 100 seconds to cure (with cure being defined as how long it takes to withstand 100 double rubs with MEK). The cure rate, as a function of time and temperature, needs to be balanced with the rest of the process line. For high-volume processes, this developing fluorosilicone coating can completely cure in 10 seconds at 230 ºC. The cure time relationship is shown in Figure 2. Typical melamine and isocyanate cure coating systems are shown for reference and as a point of relative comparison.
To understand the effect on the overall heat requirement, a simple example will highlight the impact of this lower cure temperature and cure time of the hydrosilylation-cure TFEC coating technology versus polyvinylidene fluoride (PVDF). In 2006 approximately 6.7 million metric tonnes of steel was coated globally in coil coating processes.(2) The cure temperature of the new TFEC coating is 90 ºC lower than PVDF (150 ºC compared to 240 ºC). Using the standard heat capacity of steel as 0.45 J/g/ºK(3) and assuming that 8% of the total coil coating used PVDF coating in 2006(2), the resultant energy savings is 7.9 x 1015 Joules annually. Using a natural gas price of $8.20/MCF(4) yields a net savings of nearly $150M per year.
The U.S. Department of Energy (U.S. DOE) surveyed residential houses in 2005 and found that the average annual energy consumption per house is 1 x 1011 Joules.(5) Therefore the potential energy savings with the new TFEC coating is enough to supply approximately 80,000 residential houses in the United States each year. When energy savings can be described in a manner that has a concrete foundation the impact is clear even to the most casual observer.
![]() |
Table 1 Click to enlarge |
The impact on the environment from this decreased energy use is a reduction of 975,000 metric tonnes of CO2 emitted to the atmosphere annually. It has been reported that 1 MCF of natural gas requires 0.18 tree to offset the CO2 produced in the decomposition of this 1 MCF.(6) This new TFEC coating technology, using the assumptions above, converts to a savings of 7.3 million MCF of natural gas. To offset the CO2 produced from this amount of natural gas would take 1.3 million trees to convert the CO2 to oxygen.
An even more efficient cure method is using UV energy from bulbs with a specified wavelength, instead of fossil fuel-produced heat energy. These new UV-curing systems require much less heat energy and also cure significantly faster. Improvements in both energy usage and productivity are achieved with UV cure coatings. The new UV-cure fluorosilicone-hybrid hardcoat technology gives the benefits of cost savings from lower energy demands as well as improved hydrophobic, oleophobic and easy-to-clean properties as shown in Table 1.
This new UV-cure hybrid technology can reduce the energy demand for the same quantity of coil coated by 1.76 x 1016 Joules/year, or 16.2 million MCF of natural gas. If, hypothetically, all the PVDF coating used for coil applications were replaced with the new UV-cure coating technology the reduction in natural gas combustion could yield a reduction of 2.2 million metric tonnes of CO2. This savings would result in 2.9 million fewer trees needed to convert this amount of CO2 to oxygen. The energy savings from using the UV cure coating technology would heat approximately 180,000 houses in the United States each year.
Improving Air Quality
The Earth’s ozone layer protects all life from the sun’s harmful radiation, but human activities have damaged this protective layer. Less protection from ultraviolet light will likely, over time, lead to higher skin cancer and cataract rates as well as crop damage. In response to the prospect of increasing ozone depletion, the governments of the world created the 1987 United Nations Montreal Protocol as a global means to address this global issue. As a result of the broad compliance with this Protocol and industry’s development of ozone-friendly substitutes, the total global accumulation of ozone-depleting gases has slowed and has now begun to decrease.(7) This has reduced the risk of further ozone depletion. Now, with continued compliance, recovery of the ozone layer is expected by late in the 21st century. There are currently 191 countries phasing out the production of ozone-depleting substances in an effort to safeguard the ozone layer.
For more than 50 years, chlorofluorocarbons (CFCs) were thought of as miracle substances. They are stable, nonflammable, low in toxicity and inexpensive to produce. Over time, CFCs found uses as refrigerants, solvents, foam blowing agents and in other, smaller applications. CFCs are now being regulated out globally specifically to reduce their negative effect on depleting atmospheric ozone.
However, not all chemicals containing fluorine are considered ozone-depleting substances. Fluorochemicals that do not contain chlorine are excluded from the definition of materials that have been found to add ozone depletion. Specifically, new families of fluorosilicone hybrid technologies have been developed with improvements to their impact on the environment from the choices of solvents that are used.
One of the newest of these coating innovations is a water-based penetrating stain repellent that completely eliminates solvent by using water as the diluent. This water-based fluorosilane material gives water and oil repellency to porous construction materials. Applied as a post treatment it provides both hydrophobic and oleophobic characteristics to substrates such as pavers, mortar, grout and natural stone. These new fluorosilane anti-stain treatments can replace existing solvent-based penetrants to give the desired surface functional performance while at the same time eliminating the VOCs that are used in traditional treating agents. Performance and durability have been optimized to ensure the application requirements are being met. Treated substrates retain their original appearance with reduced dirt pickup and subsequently easier cleaning.
In other recently developed fluorosilicone hybrid coating systems that still use solvents care has been taken to deliberately select solvents that are low in VOCs and do not contain hazardous air pollutants (HAPs). For example, the hydrosilylation-cure TFEC coating technology uses n-butyl acetate (n-BuOAc) as the solvent at a high solids content of 55-60%. Research has also shown that the use of t-butyl acetate (t-BuOAc) can be used in this system, further improving the environmental footprint of this coating. In 2004 t-BuOAc was designated as VOC-exempt by the U.S. Environmental Protection Agency (U.S. EPA).(8) This revision modifies the definition of VOC to say that t-BuOAc will not be a VOC for the purposes of VOC emissions limitations or VOC content requirements.
There has also been an intentional effort to select the most eco-friendly solvent at the lowest solvent concentration in the UV-cure hybrid hardcoat technology. Implementing propylene glycol monomethyl ether (PGME) as the carrier fluid for this system, at a solids concentration of nearly 40%, achieves the objectives of both creating a high-performing coating and in an environmentally conscientious manner. Higher solids content formulations, and those containing no VOCs, can be accomplished using UV-cure technologies.
There are two primary benefits to air quality. The first one is by developing high solids coating formulations, where the absolute value of VOCs emitted per unit of coating is reduced. Consider that more than 2500 metric tonnes of coating are projected to be sold in the U.S. protective coatings market by 2011.(2) The potential impact of increasing the solids content from the typical solvent-based heat cure system of around 30% to a high solids UV cure coating at 55% solids results in a theoretical reduction of nearly 600 metric tonnes of solvent annually.
The second advantage to air quality with UV-cure materials is the reduction in energy needed to cure the coating, and the corresponding reduction in emissions from generating the energy required to cure the coating. A recently completed study by the U.S. DOE has quantified energy savings from converting a heat cure coating to a UV-cure coating on aluminum cans.9 Ultimately, this report concludes that an industry-wide potential annual energy savings of 2.3 x1012 Joules by the year 2010 is possible if UV cure coatings completely replace heat-cure coatings on aluminum cans.
![]() |
Table 2 Click to enlarge |
Fluorine-Based Polymer Regulations
In recent years studies conducted by government agencies have recognized concerns regarding the bioaccumulation of perfluoroocanoic acid (PFOA) in humans. PFOA is an unintended industrial byproduct from the production of “C8” fluoropolymers. A C8 telomer is a chemical compound that contains the perfluoroalkyl group C8F17. The chemical formula for PFOA is C8F15O2H. In practice the terms PFOA and C8 (so called because of the carbon chain length) are often used interchangeably and include the principal salts of the acid (e.g., ammonium salt, alkali metal salt).
Many water and oil repellents that contain C8 telomer compounds are used for long-term protection of textiles and carpets. PFOA is very stable in the environment, so it does not readily degrade. Once it enters the body it is eliminated very slowly so PFOA may remain in the body for relatively long periods of time. The half life in humans is about 4.5 years. Although current research does not establish that the levels of PFOA found in the environment cause adverse human health effects, studies do indicate that PFOA causes adverse effects in laboratory animals that have been given high doses over a long period of time.(10)
In 2000, the U.S. EPA became concerned about data that indicated PFOA is found in human blood in the general population. Since then, the U.S. EPA and the fluorochemical industry have cooperated in studies, and collected and shared information regarding PFOA. In January 2005 the EPA published a draft risk assessment on PFOA based on the available studies and data at that point.(11) It was at this time the EPA announced the creation of the “2010/15 PFOA Stewardship Program” and asked fluorochemical manufacturers to participate in the program. To participate in this program the fluorochemical manufacturers committed to the following goals.
- Reduce product content and facility releases of PFOA, precursors of PFOA and higher homologue perfluorinated substances (e.g., C9, C10, C12) by 95% by 2010 (from a year 2000 baseline).
- Work toward the elimination of such chemicals from environmental releases and products by 2015.
- Publish annual reports on progress toward these goals.
Response to the concern over PFOA has resulted in a new developing family of fluorosilicone water-based hybrid emulsion textile treatment chemicals based on C6 chemistry. The U.S. EPA has characterized the data for C6 fluorochemicals as showing “different and less toxic” than PFOA. This is the same statement that is also used to compare C4 fluorochemicals to C8 fluorochemicals.(10) The EPA has also concluded it considers C6 and C4 compounds comparable in toxicity based on current toxicity studies.
The result of a joint development project between Dow Corning and Daikin Industries is a new fluorosilicone hybrid treatment chemical that is PFOA-free.
The problem with existing fluorine and silicone chemistry initially presented a significant technical challenge. Typically the use of a copolymer with dimethylsilicone side chain causes a reduction in oil repellency. Silicones are oleophilic by nature so a simple blend of silicones with fluorochemicals impedes the oil repellency of the fluorochemical component. Organofunctional silanes are commonly used to add softness to textiles. However, when these compounds are added as a blend to the fluorochemical treatment, the oil repellency is negatively affected. In addition, the stability of the emulsion can be an issue.
![]() |
Figure 3 Click to enlarge |
This led to the concept, and eventual successful development, of a hybrid graft copolymer. The silicone backbone of this new copolymer gives the softness to the textile while the fluorine component imparts the oil repellency and durability. Ultimately the synergy of this novel chemistry solution is achieved in this hybrid system. Table 2 demonstrates the product performance of this new C6-based textile treatment as compared to current C8 and C4 fluorocarbon treatment chemicals.
Keeping Surfaces Clean
Body oils contain lipids, salts, peptides, water and maybe even bacteria, and these components are often inadvertently transferred to surfaces that are touched. An increasing number of electronic devices now offer touch screen displays to enhance the user-device interface, where fingerprints can deposit unwanted contamination to the surface. Because of the prevalence of touch screens being used more in public places the implication for potential transfer of fingerprints, and the compounds in the fingerprint, is growing, too. Applications for public use touch screens include ATMs, check-in kiosks in airports, directional displays in shopping malls, and on public transportation. In every one of these applications undesirable contaminants may be unknowingly deposited on the surface of these displays, awaiting the next user who can be easily contaminated with these unwanted species. The need for cleaning these surfaces for hygienic reasons is obvious, but the practicality of cleaning them is very time-consuming and expensive.
In industrial settings the use of shared safety glasses presents another challenge. The U.S. Occupational Safety and Health Association (OSHA) has prescribed that decontamination of shared personal protective equipment (PPE) between uses is mandatory to disinfect the surfaces.(12) Decontamination methods must (1) physically remove contaminants; (2) inactivate contaminants by chemical detoxification or disinfection/sterilization; or (3) remove contaminants by a combination of both physical and chemical means. This process is a non-value-added cost to industry where improvements to surface chemistry may positively affect the health of the user.
Rather than trying to find a suitable decontamination method, a new fluorosilicone surface coating has been developed that actually repels fingerprints and the associated contaminants that may be contained in skin oils. This patented oleophobic and hydrophobic alkoxysilane functional perfluoro-polyether (PFPE) compound could help in keeping these surfaces cleaner. This material is especially useful on display screens and hygienic ware to reduce the accumulation of fingerprints and any natural skin oils. The comparative effect on the surface cleanliness is shown in Figure 3.
This new hybrid polymer exhibits the oleophobicity of fluorine and hydrophobicity and durability of silicone. Improved durability over current chemical treatments is obtained by the use of a monofunctional terminal alkoxy silane modification to the linear PFPE polymer. The alkoxy silane reactive end will covalently bond to the surface via hydrolysis and condensation reactions to either hydroxyl- or silanol-containing compounds. This covalent bond gives the needed durability in touch screen display applications and eyewear. The resistance to wear by rubbing makes the surface cleaner for a longer period of time, which is a major improvement of this new technology. When the surface needs to be cleaned all that is needed to remove surface dirt and oil is to simply wipe it off. The easy-to-clean surface comes from the very low surface energy of the alkoxy silane functional PFPE polymer. That means the need for cleaning chemicals to remove oil, water and dirt is minimized, and the aesthetics are improved.
Solar panels can also benefit from having the surface of the top glass sheet chemically treated. By using a fluorosilicone coating to lower the surface energy of the glass, water and dirt do not bond as easily, which means more of the sun’s energy is converted into electricity. The more physical barriers there are between the sun and the photovoltaic cell, the lower the operating efficiency. In the world of solar panels the drive now is to maximize the solar panel performance to optimize energy output. Increasing energy production in a solar panel reduces the overall cost of energy, so every incremental improvement becomes an important factor. Solar panels are expected to last up to 30 years in most applications, so a small gain in performance is magnified because of the expected service life.
The overall trend of solar energy costs, as measured by price per watt peak, continues to decline with the Solar Module Retail Price Index most recently at $4.61/watt in the United States and €4.48/watt in Europe.(13) Technologies that can continue to positively affect this trend will likely be adapted in the photovoltaic industry.
Conclusion
Innovative fluorosilicone technologies offer many improvements to the environment. Positive impacts from this new range of coating chemistry include reduced VOCs and HAPs, elimination of PFOA-containing C8 chemicals, and energy savings from coatings with reduced energy needed for curing. Less energy for curing means a reduction in fossil fuel needed to produce this energy and a resultant reduction in CO2 production.
The hybrid approach combining fluorine and silicone chemistry actually results in materials that exhibit the best characteristics of each technology. Silicones, with their natural polymer flexibility and UV resistance, and fluorine, with durable and oleophobic surfaces, are synthesized in many different polymers and resins in coating applications.
Keeping surfaces clean and making them easy to clean are not solely for aesthetic purposes. These cleaner surfaces may also reduce person-to-person contamination. Innovative fluorosilicone materials are a foundation for sustainable business and offer concrete solutions to positively affect the environmental footprint of coatings.
Acknowledgements
Mr. Mike Hales, Corporate Eco-Innovation Manager, Dow Corning Corporation; Mr. Masayuki Hayashi, Development Chemist, Dow Corning Toray Ltd.; Dr. Peter Hupfield, Technology Leader, Dow Corning Ltd.; Dr. Yasou Itami, Chief Researcher, Daikin Industries Ltd.; Mr. Eiji Kitaura, Development Chemist, Dow Corning Toray Ltd.; Dr. Don Kleyer, Senior Development Chemist, Dow Corning Corporation; Dr. Tetsuya Masutani, Business Development Manager, Daikin Industries Ltd.; Dr. Yasuhiro Nakai, Research Chemist, Daikin Industries Ltd.; Dr. Bill Schulz, Application Engineering Manager, Dow Corning Corporation; Ms. Janet Smith, Development Technologist, Dow Corning Corporation; Mr. Yoshinori Taniguchi, Process Engineer, Dow Corning Toray Ltd.
For more information, visit www.dowcorning.com/ContactUs or e-mail steve.block@dowcorning.com.
Looking for a reprint of this article?
From high-res PDFs to custom plaques, order your copy today!