In previous articles we have discussed emulsification of alkyd resins. We will continue with the focus on resins and discuss acrylic emulsion polymerization, in particular, surfactant design and how it affects the polymerization of a vinyl-acrylic emulsion. I would like to thank Bruno Dario of Indorama Ventures for being my coauthor on this series of articles, and Kip Sharp, Juliane Santos, and Fabricio Pereira of Indorama Ventures for their help. In this month’s article, I will introduce some of the materials and methods used. In the future, we will dive deeper into the characterization of the emulsions including particle size, filterable solids, electrolytical stability, and more.
The emulsion polymerization process to generate vinyl-acrylic latexes can be complex, and the replacement of alkylphenol-ethoxylate (APE)-based surfactants for environmental, health, and safety (EHS)-friendly solutions can be challenging. The higher polarity of the polymer decreases surfactant adsorption, and can also decrease the water resistance of the final coating; hence, surfactant choice is crucial in the development of resilient formulations.
The effect of APE-free nonionic surfactants with different hydrophobes and APE-free anionic surfactants with different ethylene-oxide content in the emulsion polymerization of vinyl-acrylic resins was investigated in terms of particle size, filterable solids, and stability. The results were compared against vinyl-acrylic latexes synthesized with APE-based and APE-free surfactant benchmarks.
Finally, a 45% PVC paint was formulated, and the vinyl-acrylic latex synthesized with the optimized APE-free surfactant ratio increased wet scrub resistance dramatically compared to APE-free benchmark surfactants and the commercially available APE-free latex.
Vinyl-acrylic emulsion resins are some of the most important and widely used water-based resins in the coatings industry, due to their low cost, versatility, and low glass transition temperature (Tg). The hydroplasticization of the polymer and the ability to form a film below room temperature are important features that contribute to the development of paints with low-VOC content. The poorer hydrolytic stability makes it less suitable for exterior environments, but it is extensively used in the formulation of interior paints, adhesives, and more.
The high solubility of vinyl acetate in water is one of the complexities faced when generating copolymers with homogeneous composition and particle morphology. The solubilities of vinyl acetate and butyl acrylate in water at 20 °C are, respectively, 25 g/L and 1-1.5 g/L. Due to this, their concentrations in water, surfactant micelles, monomer droplets, and particles are quite different along the emulsion polymerization process. In addition to that, the rates of copolymerization of the vinyl-acetate radical with the butyl-acrylate monomer and butyl-acrylate radical with the vinyl-acetate monomers are 0.05 and 5.5, respectively. Consequently, butyl acrylate tends to homopolymerize, and the copolymers that are rich in butyl acrylate tend to be inside of the particle, while the copolymers rich in vinyl acetate tend to be on the particle surface due to the higher hydrophilicity. The use of semi-batch or semi-continuous polymerizations with continuous additions of monomer mix and initiator has been used to produce copolymers with more homogeneous composition, distribution of monomers, and uniform morphology of latex particles.
Another challenge related to the higher solubility of the vinyl-acetate monomer in water is the emulsion-polymerization process, itself, and the mechanism to generate vinyl-acrylic resins, which usually can be better described by a controlled coagulation-nucleation mechanism. This mechanism is quite different from the micellar nucleation that is normally used to describe the polymerization of hydrophobic monomers. Furthermore, the higher polarity of the polymer decreases surfactant adsorption during the coagulative process and can also decrease the water resistance of the final coating; hence, surfactant choice is crucial in the development of resilient formulations. The replacement of surfactants based on alkylphenol ethoxylate (APE) for solutions that are more EHS friendly can be challenging. The first inclination is to replace APE-based surfactants with non-APE versions with the same level of ethoxylation, but this will, in most instances, lead to poorer-quality emulsion.
The use of conventional surfactants that are less prone to adsorb on the more hydrophilic surface of vinyl-acrylic particles is one of the reasons why it is more difficult to stabilize this emulsion. Therefore, vinyl-acrylic emulsions stabilized only with anionic or nonionic surfactants have poorer mechanical stability, larger particle size, and broader particle size distribution. To overcome this issue, commercially available vinyl-acrylic emulsions are usually stabilized by both anionic and nonionic surfactants, and a protective colloid. These protective colloids are polymers, such as poly(vinyl alcohol) (PVA) and hydroxyethyl-cellulose (HEC) derivatives, partially grafted onto the latex particles to generate hydrated shells around them, contributing to increased steric repulsion stabilization. Even though protective colloids are required to improve the overall stability of the emulsion, studies suggest that high-solid-content vinyl-acrylic latexes are only stabilized in the presence of nonionic surfactants. Despite this, there are few studies in the literature that evaluate and discuss the performance of nonionic surfactants with hydrophobes, different from alkylphenol. Because of this lack of literature, we decided to study this testing.
Even though steric stabilization plays an important role in the stability of vinyl-acrylic emulsions, these polymers are also stabilized through electrostatic forces. Sulfate groups from the initiators are usually located on the surface of the particle conferring an electrostatic barrier to coagulation. Anionic surfactants also contribute to it, and there is a conviction in the market that anionic surfactants with higher content of poly(ethylene oxide) chain are more appropriated for emulsion polymerization of vinyl-acrylic latexes. However, there is no technical evidence concerning emulsion polymerizations that supports these suggestions.
Therefore, we investigated the effect of APE-free anionic surfactants with varied sizes of poly(ethylene oxide) chains and APE-free nonionic surfactants with different hydrophobes on the copolymerization of vinyl-acetate and butyl acrylate. An APE-based and another APE-free package of surfactants were chosen as benchmarks. The emulsion quality was investigated in terms of particle size, filterable solids, electrolytic resistance, and stability. The emulsions synthesized were formulated in a 45% PVC paint, and the results were compared against benchmark vinyl-acrylic latexes synthesized with APE-based and APE-free surfactants in terms of scrub resistance, blocking resistance, and touchup.
Materials and Methods
Surfactants, Vinyl-Acrylic Emulsions, and Paint Formulation
The surfactants used in the study and their main properties are summarized in Table 1.
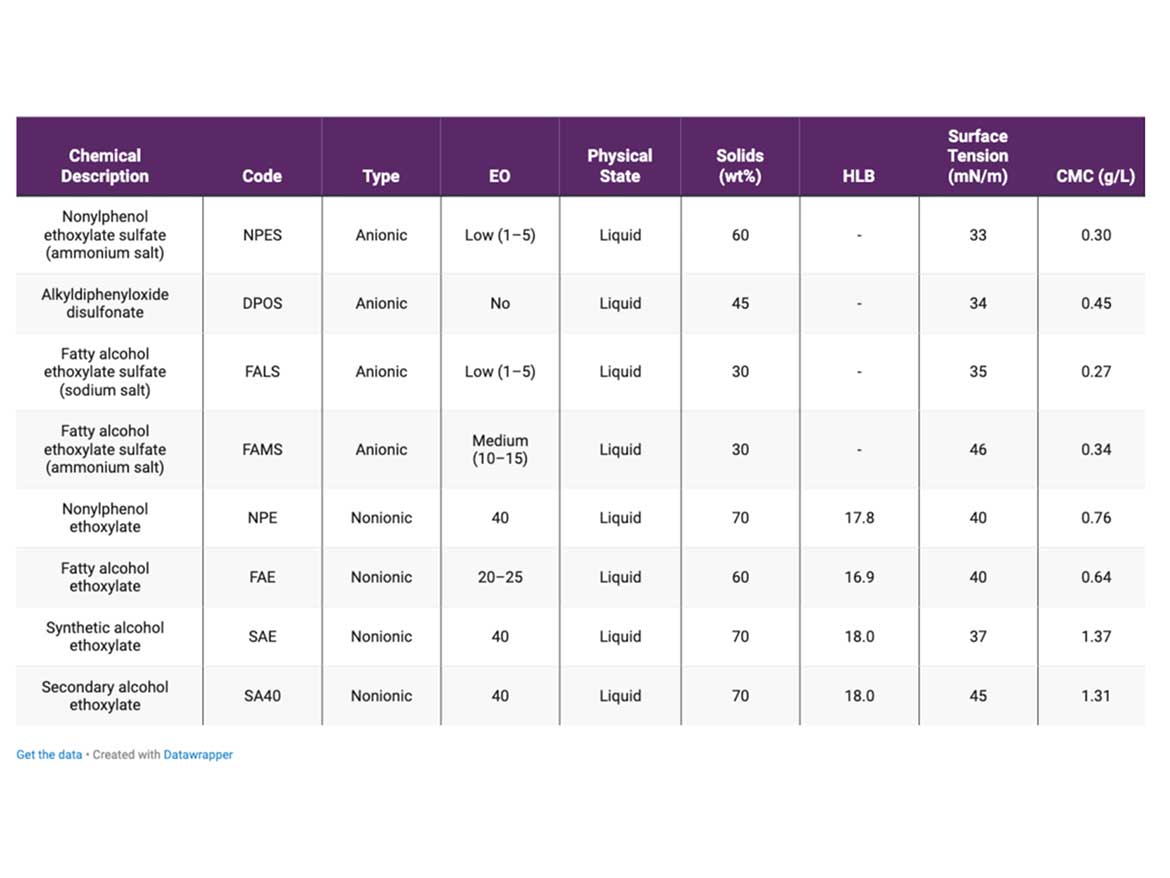
Table 2 contains the general formula for the vinyl-acrylic emulsion. The monomeric composition was chosen to generate latex with calculated Tg about 1 °Cso it would require minimum coalescent, and due to this, allow the development of paint formulations with VOC content lower than 50 g/L. The emulsions were synthesized in a 3-L reactor following a semi-continuous process under starve-feed conditions.
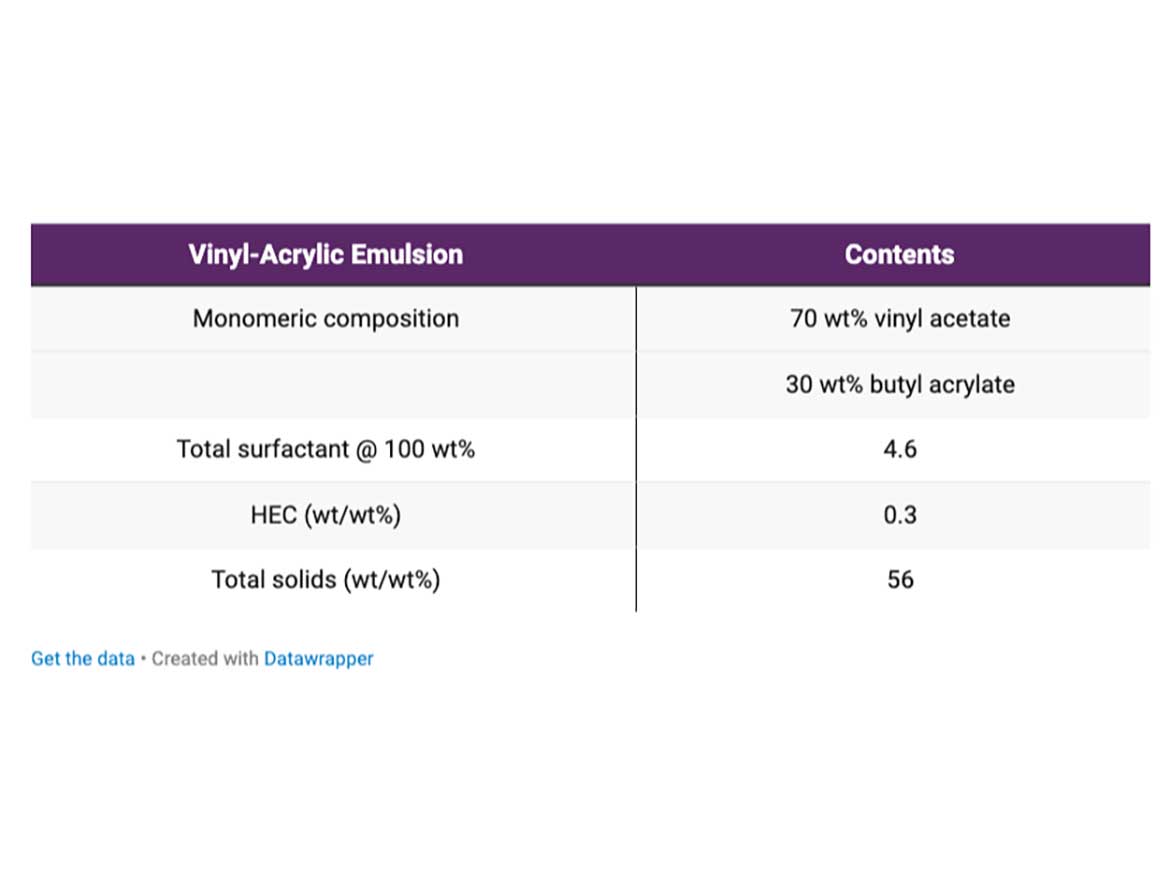
The compositions, processes, and raw materials used in this study were not changed during the assays. The only difference between the formulations is the type of surfactants used and the ratio between anionic to nonionic surfactant. Table 3 contains the different surfactant compositions used in the study and the code for each formulation.
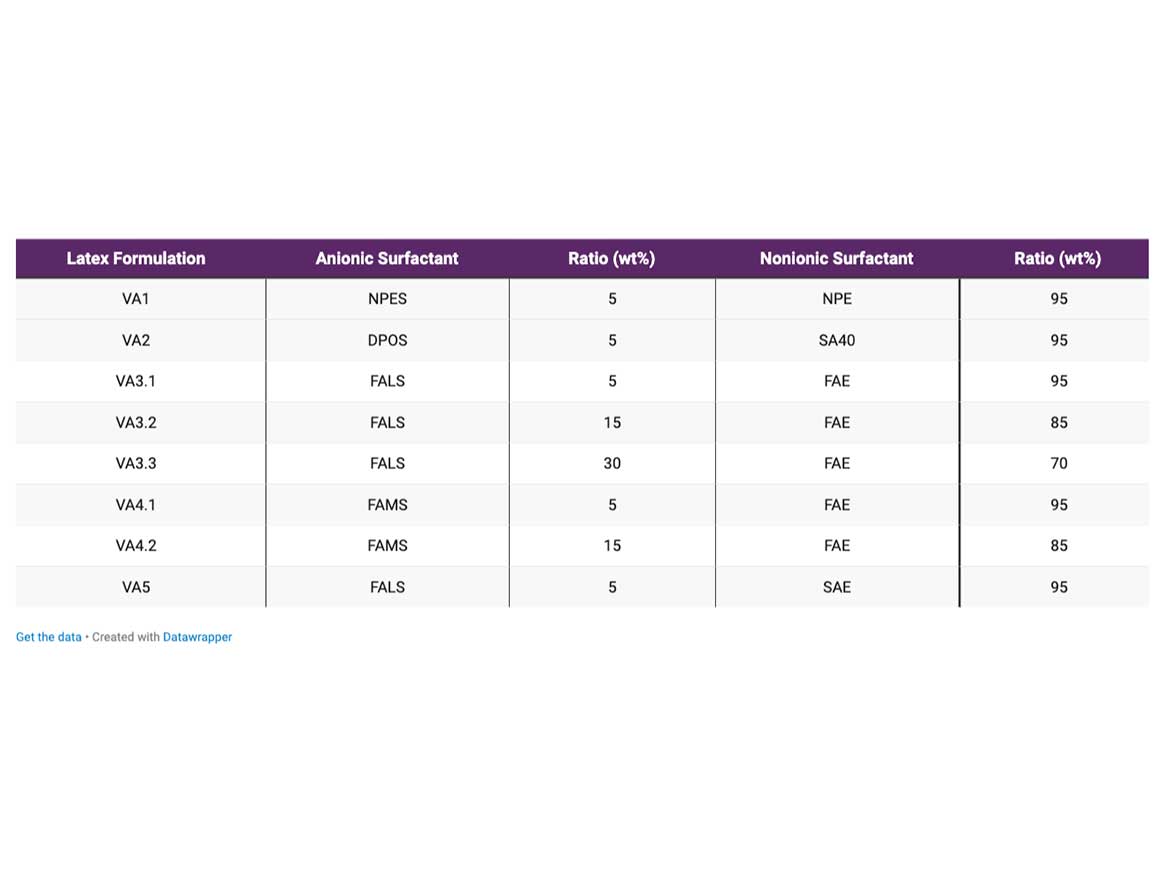
For the paint evaluation, five of the latexes synthesized were selected and two benchmarks were chosen. The benchmarks represent an APE-based and an APE-free latex. Table 4 contains the codes for the paint formulation according to the vinyl-acrylic latex used in each one.
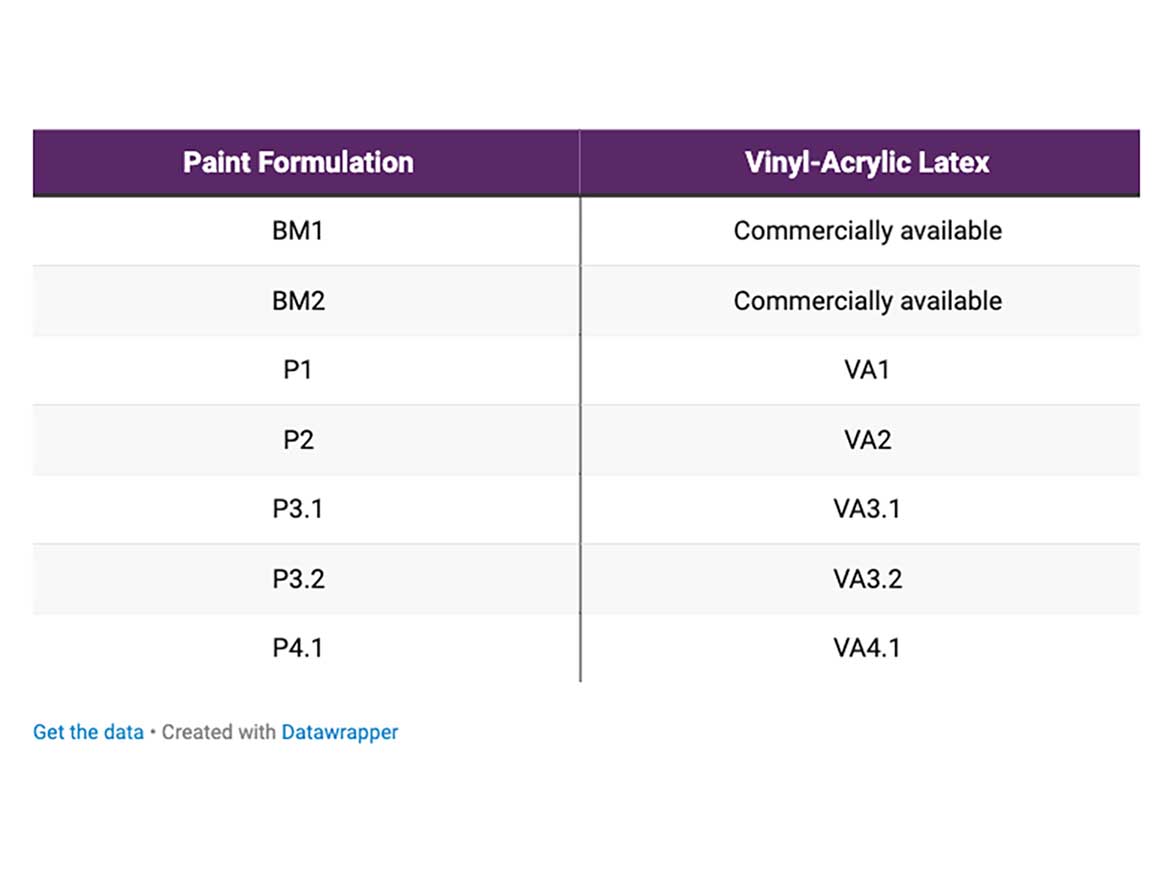
All formulations had the same composition and were prepared according to the same process, except for the vinyl-acrylic latex used as benchmarks, which were purchased. The paint formulation is a 45% PVC with calculated VOC lower than 15 g/L.
Vinyl-Acrylic Latex Characterization
Filterable Solids
The latexes were filtered through 40-mesh and 150-mesh sieves to collect filterable solids, which were quantified by gravimetric method.
Solid Content
The solid content of the latexes was determined by the gravimetric method according to EPA Method 24.
pH and Viscosity
The pH of latexes was determined using a benchtop pH meter using 4, 7, and 10 pH standards. The viscosity of latexes was measured at 25 °C using a spindle rheometer.
Particle Size
Particle size of latexes was determined in a Zetasizer NS from Malvern.
Mechanical Stability
The mechanical stability of latexes was performed according to ASTM D1417 using a Klaxon MK3 latex stability test machine from LATEXMST.
MFFT and Tg
The minimum film formation temperature (MFFT) was measured according to ASTM D2354. The Tg was measured according to ASTM D3418.
Contact Angle
The latex films were cast on a glass substrate using a 150-μm film applicator and then dried for 7 days at 25 °C and 60% R.H. The contact angle of deionized water on the cast films were measured on an OCA 15 from Dataphysics.
Critical Coagulation Concentration
The critical coagulation concentration (CCC) of the emulsions were performed titrating the emulsion with 0.1 wt% solids of a 1 mol.L-1 CaCl2 solution, and monitoring the turbidity or the particle size of the latex. The onset of the increase of the turbidity or particle size is the CCC.
Paint Evaluation
Viscosity
The paint viscosity was measured at 25 °C in KU-2 viscometer from Brookfield.
Wet Scrub Resistance
The wet scrub resistance of the paints was performed following ASTM D2486. Blocking Resistance
The blocking resistance of the paints was performed following ASTM D4946.
Leaching Resistance
The surfactant leaching of the paints was performed following ASTM D7190.
Touchup
The touchup of the paints was performed following ASTM D7489.
In next month’s edition of “Formulating with Mike,” we will show results from the testing of these emulsions.
All information contained herein is provided "as is" without any warranties, express or implied, and under no circumstances shall the author or Indorama be liable for any damages of any nature whatsoever resulting from the use or reliance upon such information. Nothing contained in this publication should be construed as a license under any intellectual property right of any entity, or as a suggestion, recommendation, or authorization to take any action that would infringe any patent. The term "Indorama" is used herein for convenience only, and refers to Indorama Ventures Oxides LLC, its direct and indirect affiliates, and their employees, officers, and directors.
Report Abusive Comment