Crystal Glass Pigments

Image courtesy of Ink Invent.
Safety Made Beautiful
Although increased visibility and improved thermal comfort, with reduced energy consumption, have been long sought-after benefits in the automotive and mobility industry, actual commercial and industrial viable solutions and applications have been lacking.1 Ways to solve these conundrums have been confined to binary solutions of a very-useful, but limited, number of light sources and retro-reflective reflectors, to accentuate extremities, and provide information on orientation of traffic participants and traffic objects. Another approach has been the development of a new NIR-transparent, black pigment, letting NIR radiation through, scatter-back-reflecting a small part of NIR radiation on a NIR-reflective primer coating, specifically for improved LiDAR visibility.2 However, these solutions still do not provide for a full visual grasp and understanding of the complete objects in an actual real-life day and/or night situation under different visibility angles for different visibility-capturing methods.2,3
Even though the application of retro-reflective pigments as a potential solution has been researched since the first half of the last century, and numerous patents have been filed in this field, RheoLight™ is the world's first 3D, form-free, industrially applicable and commercially viable, patent-portfolio-protected crystal glass-pigment (CGP) technology. This technology provides perfectly round crystal glass-microsphere dispersions that solve the technical challenges for incorporation in high-end color coating systems, providing increased visibility and detectability, while still maintaining the high-end thin-layer finish required by the automotive and wider-mobility industry.
Crystal glass pigments open up a new color dimension and enable a new toolset with a new freedom in color design, while integrating safety-enhancing features to the exterior of vehicles and objects. Brands can now market their consumer-favored, metallic, pearlescent, and darker-colored vehicles, without compromising on safety. Overall, enabling increased safety in the traffic environment by providing increased object visibility and detectability for all traffic participants. This new color dimension will aid in the safe transition to full autonomous driving.
Color Landscape Revolution
Going back to the first half of the last century, the introduction of effect pigments, such as metallics and pearlescents, constituted a revolution in the then-existing color landscape. The existing concept of solid colors in all different chromas, tints, shades, and hues was radically transformed by adding a new pigment morphology: flakes, in all different shapes and sizes. New color concepts like ‘flop’ and ‘sparkle’ saw the light of day and were subsequently quantified and characterized with newly developed color-measuring equipment. In other words, the introduction of effect pigments to solid colors brought about a first paradigm shift in the color landscape. By the 2000s, effect-pigment coloring technology evolved into full incorporation within the automotive sector, with currently over 60% of all new cars boasting effect pigments with different gradings of flop and sparkle.
Now, in the 2020s, the introduction of yet again a new morphology in pigments, namely perfectly round crystal glass pigments (microspheres), is set to enrich the existing color landscape of effect-pigment color stylings with a fundamental, new color concept. By using crystal glass pigments in new color formulations, a new play with light is introduced and a new, unexpected color dimension revealed. The impact of this change in the color landscape has the same magnitude in size of the paradigm shift introduced to the solid color landscape by effect pigments last century.
The second-generation RheoLight crystal glass pigments have now been completed with the world's first perfectly round crystal glass microspheres with a narrow particle distribution between 1-10 microns. They are provided in proprietary formulations, allowing stable storage, and direct and post-addition use in both water- and solvent-based coating systems. Providing visibility enhancing solutions for all traffic participants and objects.
A New Color Dimension: Twin-Flop
Solid colors reflect incoming or incident light in a diffuse manner. Light is reflected outward again in all directions with a relatively homogeneous distribution (see the picture on the left in Figure 1). As mentioned earlier, with the introduction of metallic and pearlescent colors in the first half of the last century, the ‘flop effect’ was introduced into the color landscape. ‘Flop’ means that light hitting an object’s surface is mostly reflected away in a specular (mirror-like) manner, in contrast to the relatively homogenous diffuse or scattered light reflection for solid colors (see the second picture from the left in Figure 1). Effect pigments thereby reduce the amount of light returning to the light source.
This also holds true for LiDAR signals, which are mostly reflected away from surfaces with high-effect-pigmented color coatings. Consequently, the amount of returning light or returning LiDAR laser signal, in the direction it came from, is reduced. This means that from the perspective of a driver in a car with headlights or a LiDAR system mounted in or on the car, most light and signal information is reflected away from where it came from. High-flop-index colors thereby reduce visibility from a driver or LiDAR system perspective.
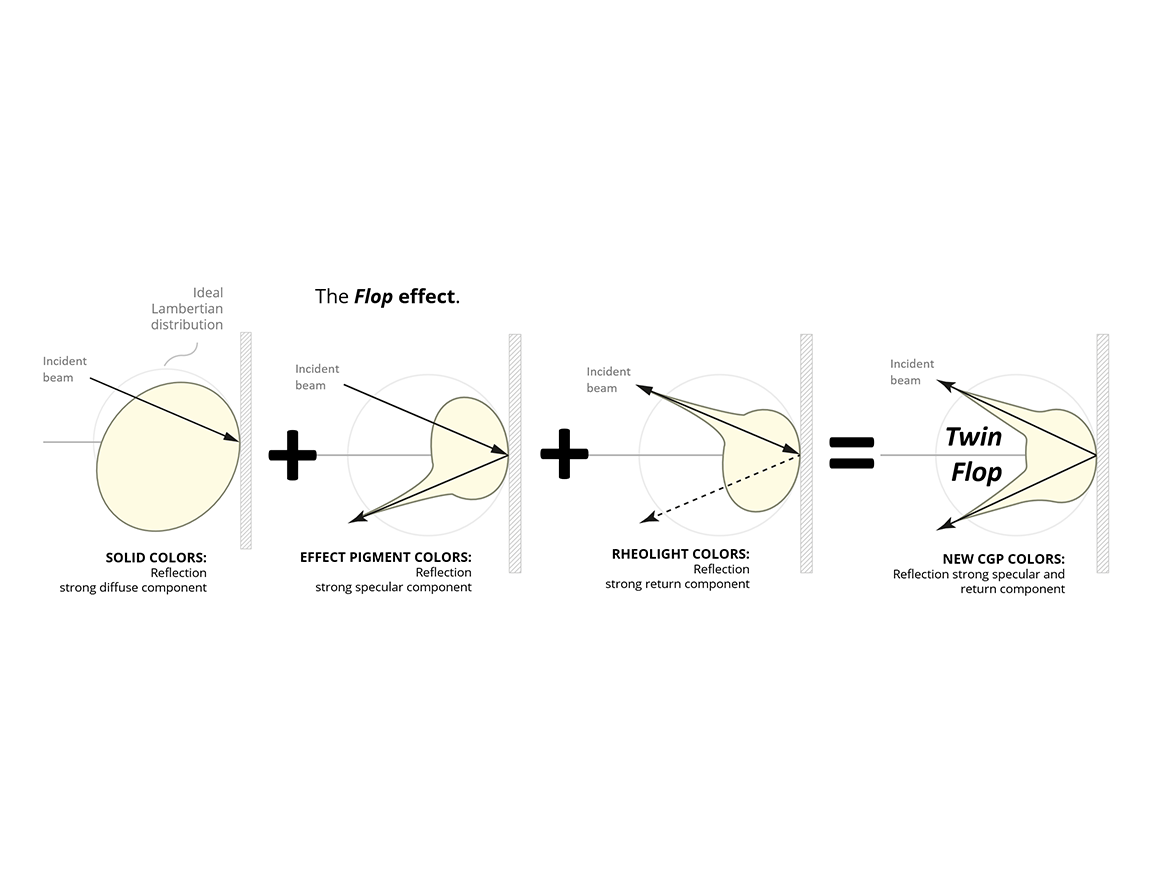
When adding high concentrations of crystal glass pigments, incoming light and LiDAR signals are returned for a very-significant part along the incident angle, thereby significantly increasing visibility for a driver or LiDAR system. When combining crystal glass pigments in lower concentrations, in an optimal way, in combination with solid colors and effect pigments, the new twin-flop effect is realized.
This twin-flop effect is a unique property, intrinsic to crystal glass pigments, which always adds increased point-of-view visibility to colors. In each application, crystal glass pigments always reflect a return signal component back along the direction of incoming light or laser. By optimizing the addition of crystal glass pigments, the best of both worlds is combined in retaining the desired ‘flop effect’ (caused by the orientation of effect pigments in an aligned manner), and adding a return signal along the direction of the incoming light or laser source. This results in the twin-flop effect, while enhancing the point-of-view visibility for every coating, as shown in Figure 1.
Tunable Detection and Determination Technology
Applied in a color coating in low concentrations (i.e. below 5 w%), crystal glass pigments give a more-subtle twin-flop effect, a subtle new play with light for optical appeal and design (see the left picture in Figure 2). In higher concentrations (i.e. up to 25 w%), the addition of crystal glass pigments significantly increases the visibility of vehicles in any color, but trades off the ‘flop effect’ (see the right picture in Figure 2). In low concentrations, while retaining the flop effect, RheoLight can, for the first time, make metallic and dark-colored objects more visible to LiDAR systems where such colors were previously not or hardly detectable. Where mobility color trends are still dominated by dark colors and metallics, manufacturers now have the opportunity to offer these CGP-enhanced colors that are more visible for LiDAR systems.
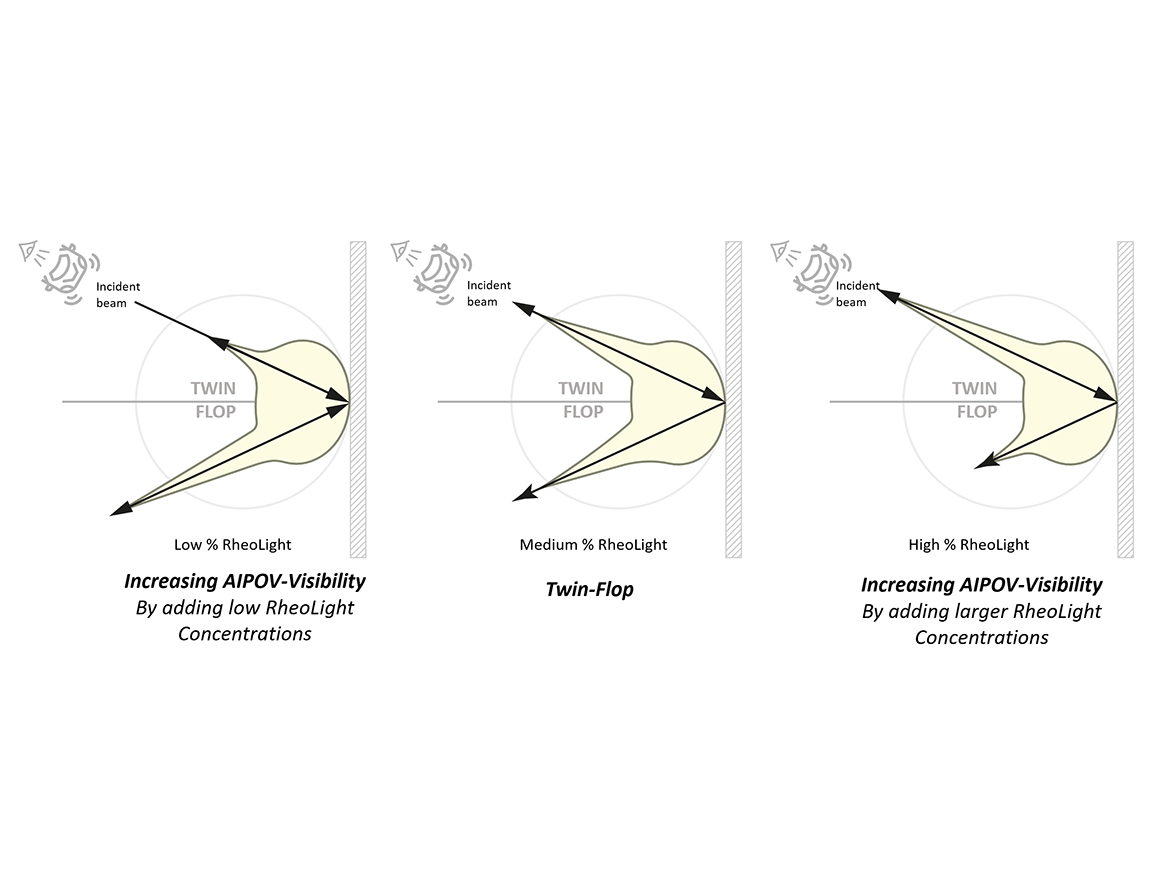
Vulnerable road users (VRUs), such as bikes and scooters, often opt for the higher return visibility of the higher concentrations of crystal glass pigments, with a strong focus on the increase in return signal of LiDAR and incident light for enhanced safety. In other words, the addition of crystal glass pigments provides a tunable detection and determination (TDD®) technology. This technology allows for enhanced visibility of objects for computer vision, LiDAR, and the human eye, enabling improved accuracy and response times in traffic environments.
Point of View Visibility
With crystal glass pigments integrated into new colors stylings, color in itself has definitively moved into the realm of functionalized utilization of its intrinsic properties, thereby profoundly changing the color landscape. Colors already have a recognized visibility and thermal-comfort effect on objects.2 For example, it is well known and accepted that a white car at night is more visible than a black car, and everyone would prefer to get into a cooler, white car mid-summer, over getting into a black car.
In the case of color visibility of objects, one is essentially talking about the perceived shape and movement of an object within its surroundings. The resulting visibility of the object for an observer is the result of diffuse and specular reflecting surfaces, lighted up from the different available light-sources. Visibility in traffic environments, however, is specifically concerned with the visibility from the viewpoint of the driver or observer, who has to make decisions to stay safe and arrive on time, and in one piece, at their intended destination.
In well-lit environments, visibility is often not recognized as an important topic, as nearly all objects are easily seen and discerned against their surroundings. Lower-visibility situations and complex, higher-speed situations that call for a higher degree of interpretation, improved visibility becomes more important. In these cases, for example, headlights of a car and ADAS systems, such as LiDAR, computer vision, and pseudo-LiDAR, are used to aid in providing accurate, integrated, and reliable data, supporting better and quicker decision making. Especially in situations where complexity and traffic participant speed increase, and quick but reliable decisions need to be made, supportive data and information must be of the highest quality and reliability to avoid accidents, and minimize subsequent injuries and damage.
In these cases, the point-of-view of the driver or observing (ADAS) system is quintessential. A driver is sitting in his car looking outward at the world around him, trying to make sense of it, maneuvering through it with the help of the car’s headlights. In the same way, an on-board LiDAR or multi-camera system is also looking outward from the car to the world, trying to aid in providing a better understanding of the location, movement, and intent of other traffic participants, and location of possible dangerous objects, relative to the car (see Figure 3).
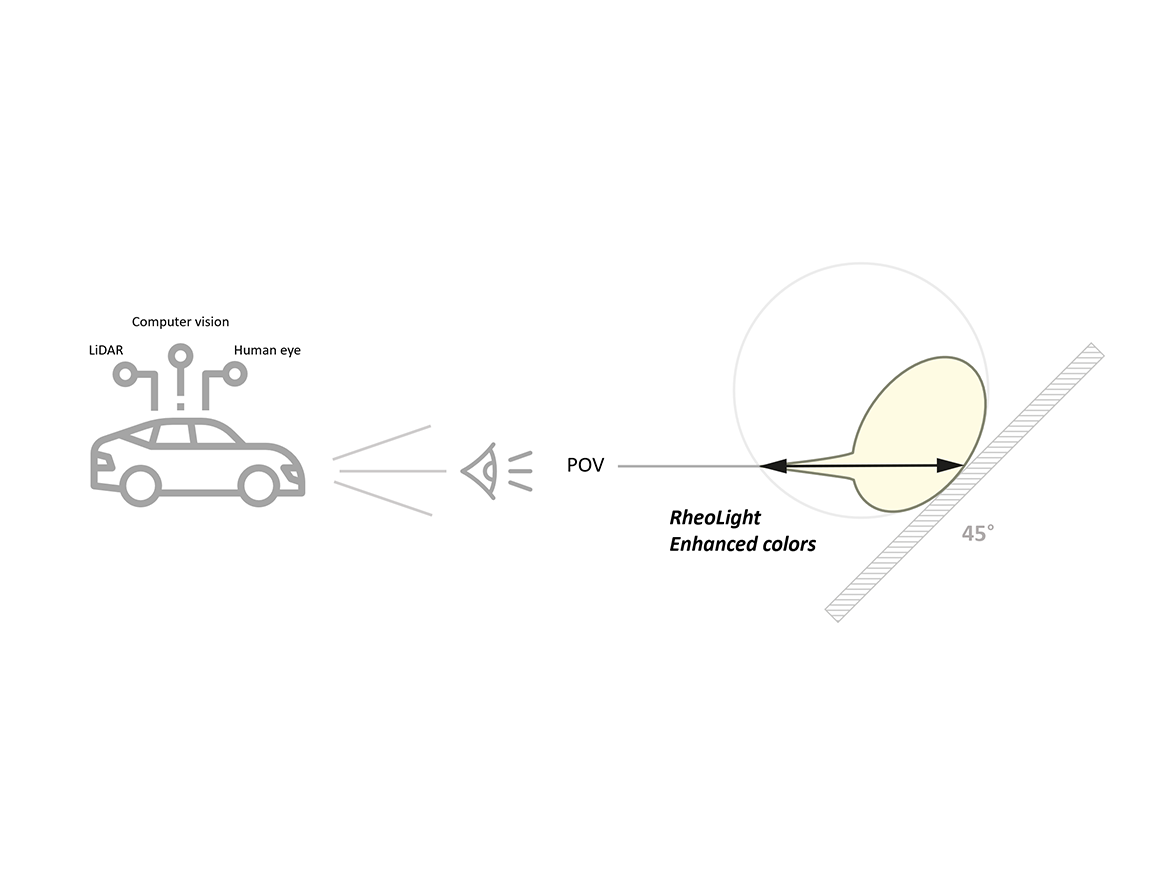
So, a need for this sharper definition of visibility in traffic situations starts to emerge. Point-of-view visibility is defined as being from the vehicle outward, along the line of headlight and laser signals, from both the driver and ADAS systems, and determines the originating set-up of a new visibility concept, namely point-of-view (POV) visibility.
Decreased POV Visibility of Metallic Curved Surfaces
With this new POV-visibility approach and recognition of the importance of the visibility of curved surfaces and its consequences within a low-visibility environment, the visibility effect of solid colors and the addition of effect pigments can now be revisited.
When looking at solid colors, a diffuse reflection causes an (for that color) optimal scattered reflection in all directions (for white solid colors, even near-Lambertian). This happens for all incident angles. The return signal or visibility of a surface at a higher incident angle still returns a relatively optimal amount of visibility information, even though it decreases in size with increasing angles.
When adding effect pigments, however, the inherent reflective properties cause the incoming light and laser signals to reflect away in a specular fashion. Thereby deflecting light and LiDAR signals, and diminishing the amount of information returning to the source of headlight (i.e. driver) and LiDAR system. This is an unintended but glaringly obvious side-effect of the addition of effect pigments to colors, which introduce a strong and intended specular color effect. This specular color effect creates the so-called flop effect or high-flop-index colors, developed to add a luxurious look and feel to high-end car colors. However, with the introduction of the new POV-visibility concept, and recognition of the need from the driver and ADAS system for improved return signals, effect pigments therefore have a strong tendency to accelerate the decrease of point-of-view visibility for curved surfaces exponentially.
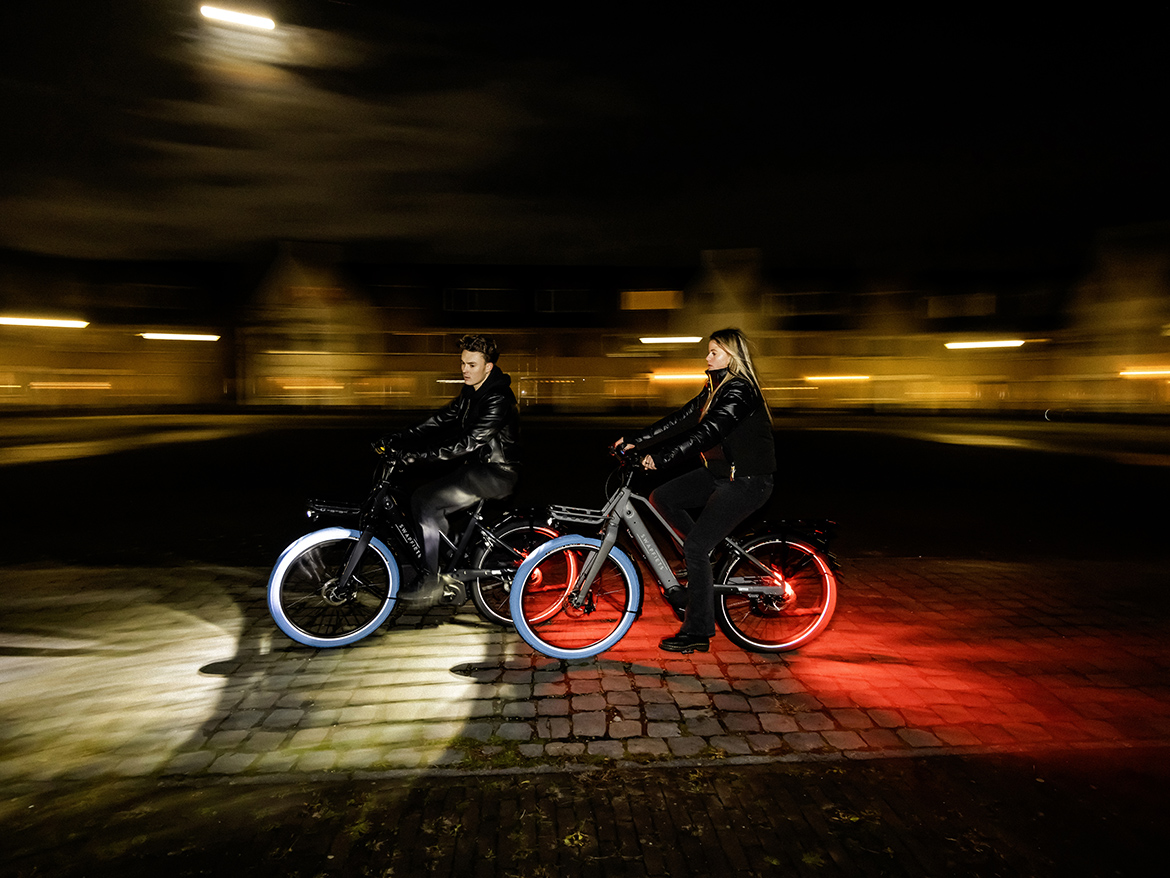
99% of Traffic Participants’ Surface Area is Curved (Away from POV)
When taking POV visibility from the observer (driver or ADAS system) as the basic premise, the next new visibility aspect arises. As much as possible of the complete form of other traffic participants or objects needs to be seen for an optimal understanding and interpretation of a traffic situation. During the day with ambient and diffuse lighting this does not pose a serious issue. Our mind even plays tricks on us. We know, for example, a car to be just one shade of silver metallic gray, but we see many, many shades of gray. So which one color does the car have in Figure 4, and how many colors and hues do we actually see?
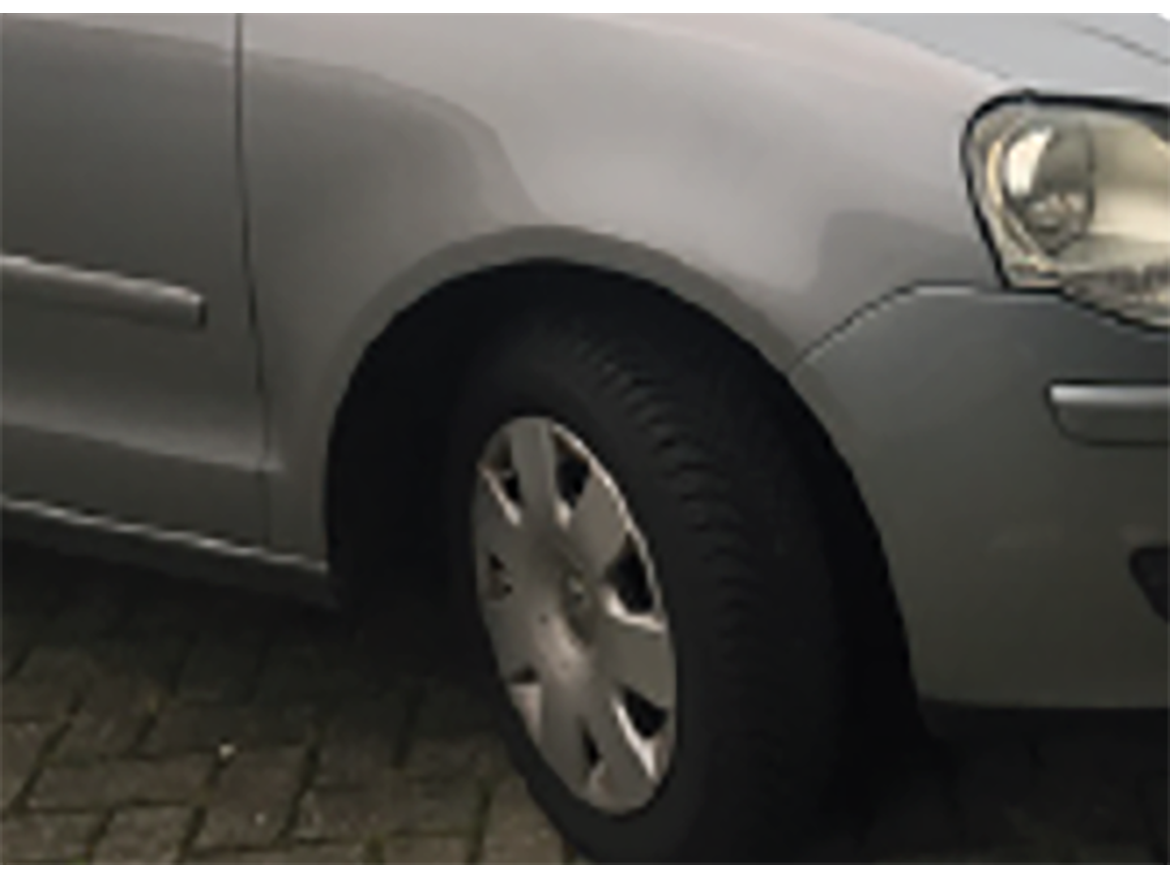
At night-time, however, or in dimly lit surroundings, visibility changes and point-of-view visibility become more important. At night, for example, while perpendicular parts are highly visible due to specular reflection of the headlights, these perpendicular areas to the viewing angle are relatively small. All of its curved surfaces, curving away from the perpendicular smaller part, are much larger, while they decrease sharply in POV visibility with increasing angle (see Figure 5).
The relatively large ratio of the larger curved surface area with regard to the smaller perpendicular area calls for a new way of interpreting full-object visibility. We think we see the car completely, but our mind translates and interprets reality to what we think we see, as the visibility of areas with increasing angle recedes quickly. We think we see the whole car, but only a small part is actually very visible and a much larger part decreases in visibility quickly. This effect also influences the object sharpness or contrast of the edges of the car. At these higher angles the car visibility morphs into the black surroundings (see Figure 5), thereby also decreasing the propensity for object recognition and determination.4
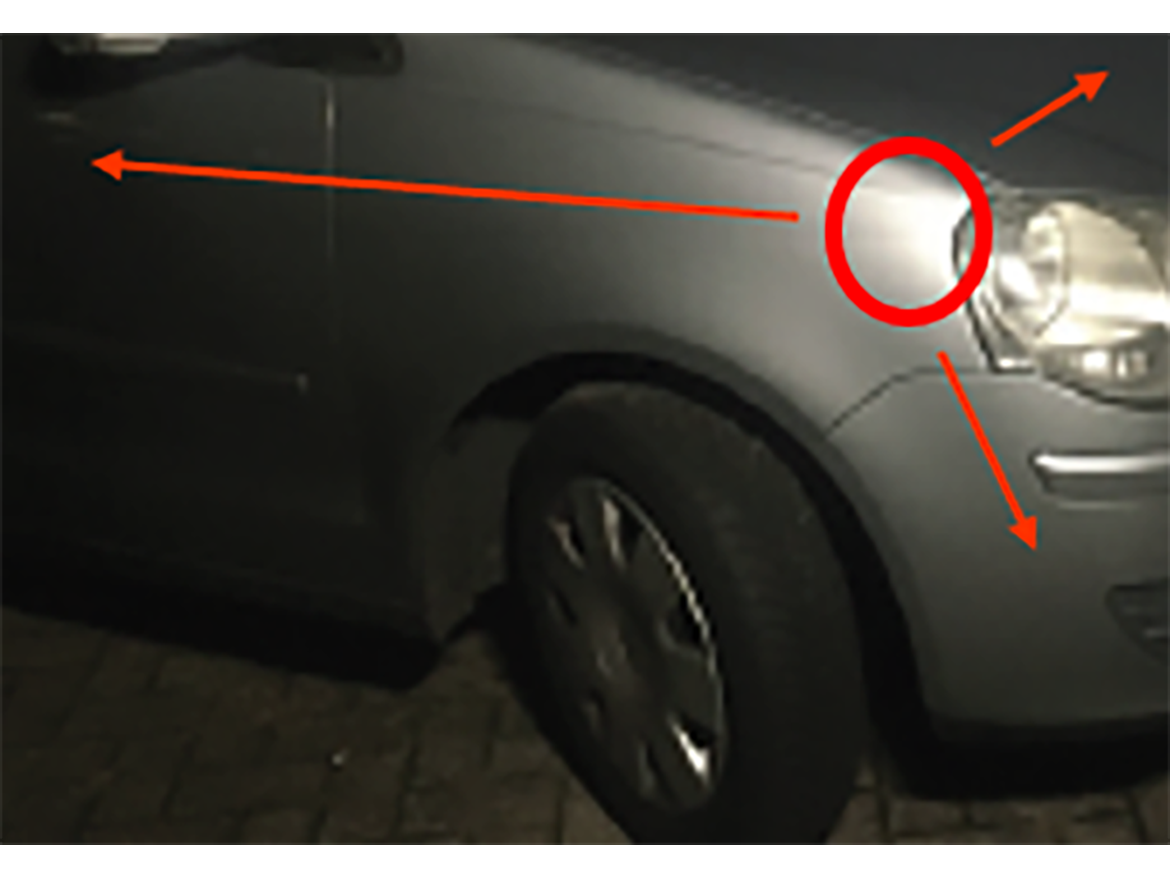
Summarizing, during the day and in highly lit environments, specular and diffuse reflection of external (non-POV) light sources take care of visibility of, for example, a complete car. In poor-visibility circumstances, the observer’s car headlights and ADAS systems are intended to help in enhancing sight and understanding for traffic participants of the traffic situation, with LiDAR being an active system, and computer vision a passive system requiring reflected headlight. With a decreasing visibility of increasingly angled surfaces, the accuracy and resolution of pseudo-LiDAR depth maps, for example, deteriorate quickly in low-visibility environments of dark-colored and metallic-coated objects. This is where an unmet and still unknown need for increased visibility surfaces occurs.
Angular-Independent Point of View – AIPOV – Visibility
As discussed earlier regarding point-of-view visibility, all colored surfaces become less visible under increasing angles of observation, even during the day. In a traffic environment, where safety is driven by detectability and visibility, not being visible or decreased visibility is detrimental to the safety of all participants. Crystal glass pigments provide a tool for significantly influencing the visibility of a surface under increasing angles of observation in a positive way.
Angular-independent point-of-view, or AIPOV, visibility is a concept first introduced by Ink Invent in 2023 to describe this behavior, the opposite exhibited and demonstrated during the development of NIR-transparent black coatings,2 especially with regard to reduced backscattering of increasingly angled black surfaces. Measuring angle-dependent reflectance in the infrared spectrum is also highlighted in a Sensors 2020 article acknowledging this ubiquitous decrease of reflectance along the incident angle with increasing angles of incidence.3 AIPOV visibility refers to the 3D visibility of colored surfaces, especially under varying angles of incidence. The orientation of the surface is taken into account with regard to the direction of lighting (headlights and LiDAR signals) in relation to the viewing angle. Traditional visibility is limited to the near-perpendicular, single point-of-view (see Figure 5) such as when an object is viewed under an angle. However, increased AIPOV visibility by crystal glass pigments expands the visibility of objects in a three-dimensional set-up, making these shapes more easily detectable and recognizable.
Crystal glass pigments, with their unique properties, provide increased AIPOV visibility by reflecting light back to its source, independent of the angle of the surface. This enhances the full visibility of objects coated or formulated with crystal glass pigments, making them more visible and detectable to the human eye, to LiDAR systems, and for computer vision technologies (see Figures 6 and 7).
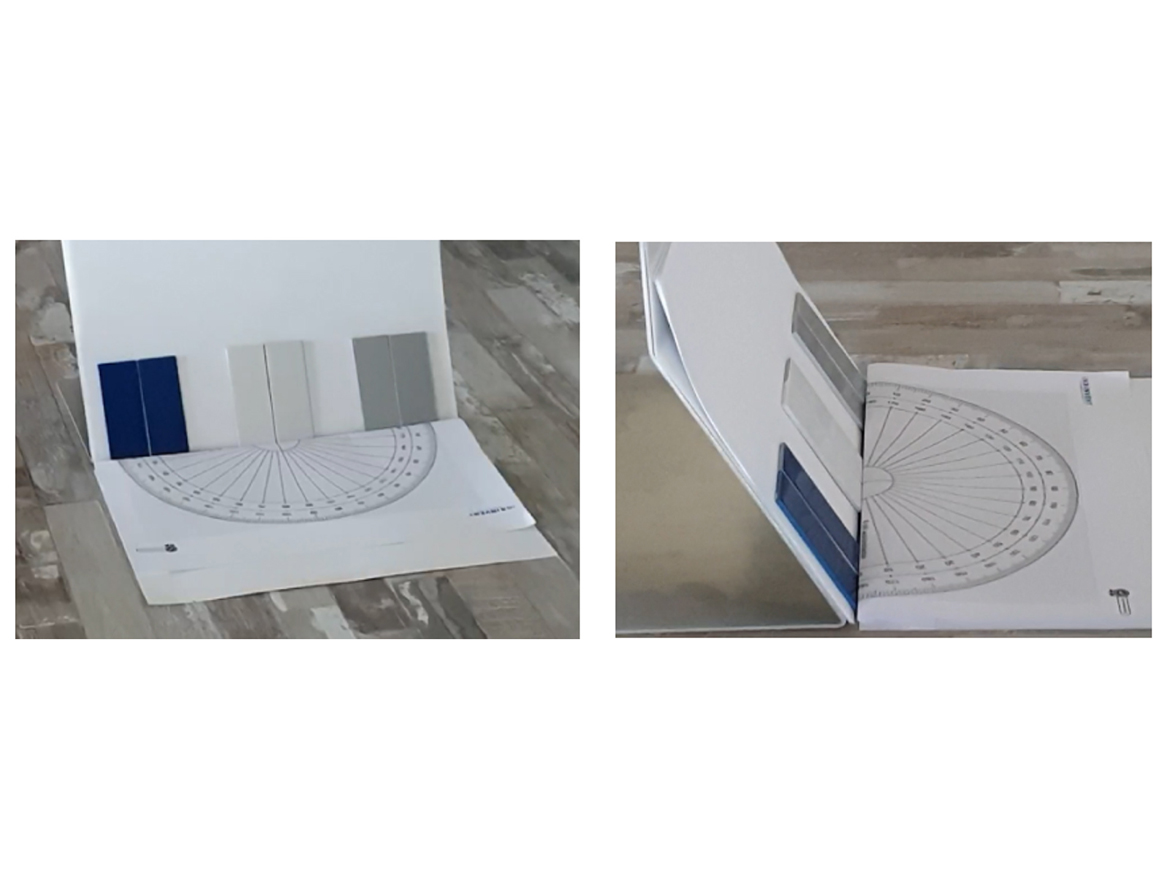
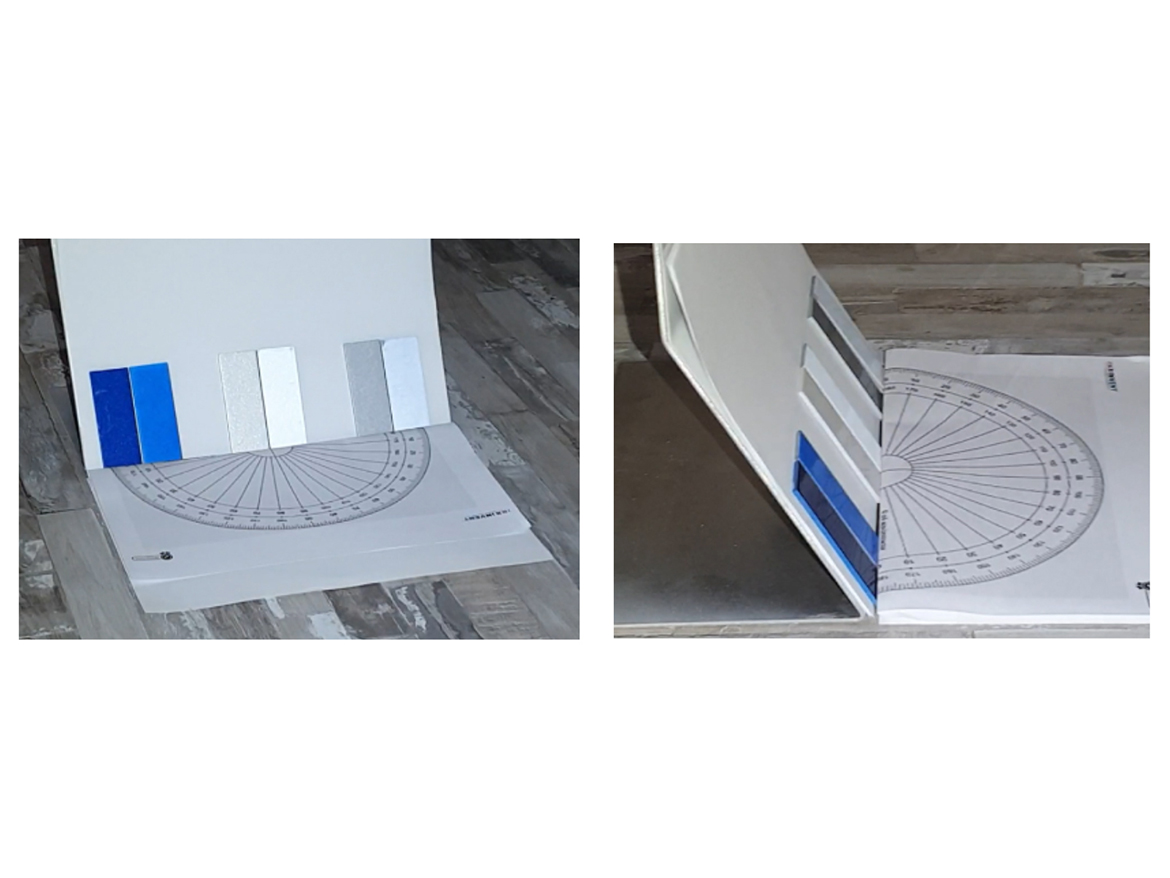
By increasing AIPOV visibility, crystal glass pigments aim to improve safety in many applications, especially in the automotive and mobility industry, where enhanced visibility can contribute to better object detection, and thereby decreased number of incidents by accident prevention (see Figures 8 and 9).
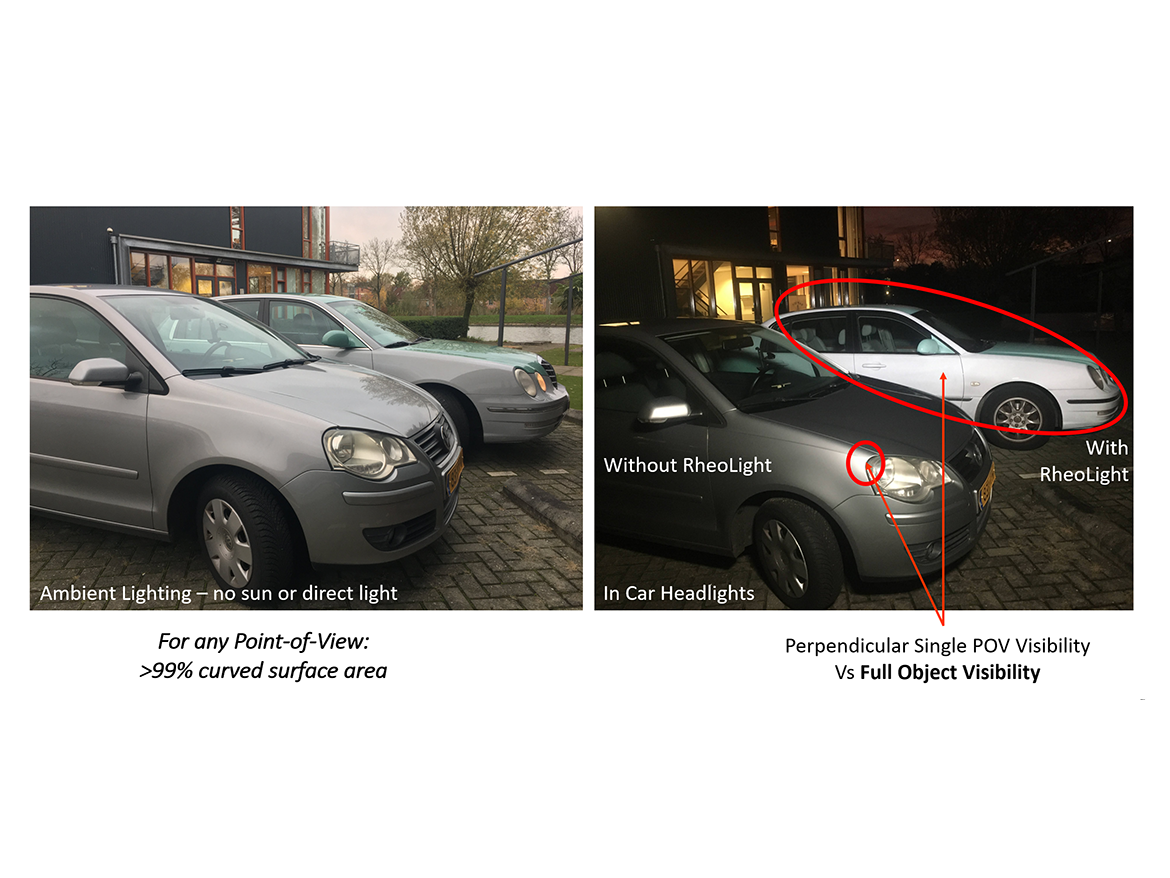
Metallic colors without crystal glass pigments demonstrate perpendicular, single point-of-view visibility of a small area, with receding visibility at higher angles, and morph into a non-contrasted background.
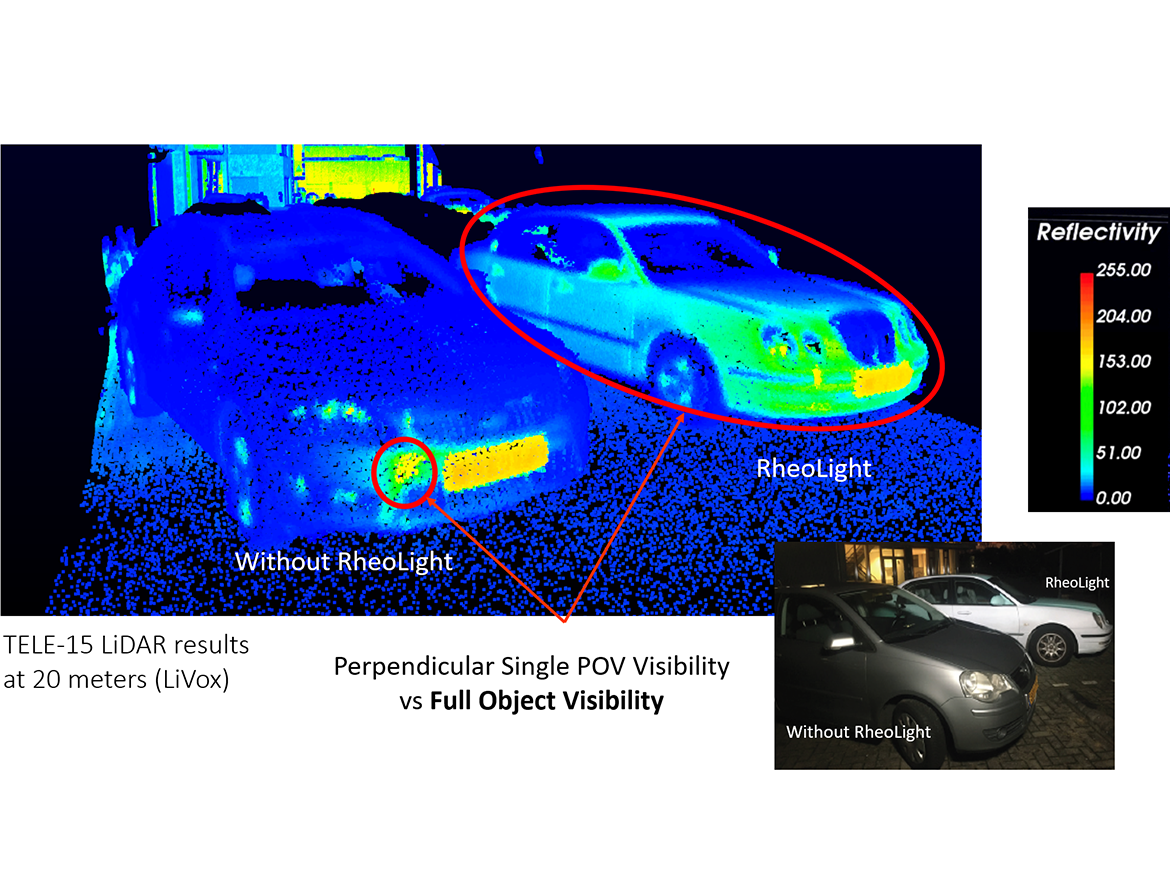
Results at a Glance
- A novel class of effect pigments allows for increased visibility.
- Twin-flop introduced as a new aesthetic styling parameter.
- Crystal glass pigments to improve point-of-view visibility of curved surfaces.
- Crystal glass pigments improve angular-independent point-of-view (AIPOV) visibility.
- Perylene black increases LiDAR visibility for black colors, but does not solve for low contrast.3
- Enables the development of a 3D-color visibility label, empowering real-life color comparison.
- Demonstrates a propensity for improving heat management.
- Aiding OEMs to address beauty, safety, and energy management.
- Will help facilitate the transition to self-driving cars.
The Future: Crystal Glass Pigments
In its engineering and functionality design, the patented technology platform, RheoLight, was optimized for form-free 3D application of crystal glass pigments, while still giving a high-end coating finish and providing a new and innovative color styling tool. As an industrially applicable and commercially viable solution, it provides increased safety for all by allowing automotive and mobility brands to (tunably) integrate safety into their newest functional color designs. The design freedom that comes with the new color dimension that crystal glass pigments unlock, makes this new class of effect pigments a highly sought after and extremely interesting styling parameter for designers and brands to include increased visibility and detectability in their new mobility value propositions.
Due to new requirements for LiDAR visibility of colors and total solar reflectance, technical and functional departments are already designing and developing new sensor-friendly colors together with CMF-design departments. Crystal glass pigments enable the creation of colors that provide a sharper and visual definition of traffic participants and objects from a point-of-view perspective. They provide a more data-dense and accurate representation of objects in 3D space, with a sharper definition and lower-fuzzy depth appearance. As LiDAR works 24/7, the integrated visibility for LiDAR systems is welcomed throughout color and functional design processes. Furthermore, given the mode of action of reflecting more light, which includes heat-wave radiation under increasing angles of incidence, crystal glass pigments are also set to provide a cooling effect when compared to colors without these pigments. Keeping the interior of a car cooler and thus consuming less energy for air conditioning systems adds an attractive and valuable design component for energy management in both EVs and traditional mobility.
Crystal glass pigments for a brilliant, beautiful, and safer world.
*All graphics and photos courtesy of Ink Invent BV.
References
1 Crosby, M.; Swain, S.; Bendo, A.; Brown, P.; Frey, T.; Jakobi, M. Improving Lidar Signal Reflection Using Functional Black Pigments. 2016.
2 Brown, P.; Heiler, J.; Driving Improvements in NIR Management. European Coatings Journal, 2021.
3 Kline,A.; Kline, D.; Kline, T. The Adrian/CIE Visibility Model: A Visibility Level Calculator & Future Research. Journal of Science and Technology in Lighting, 2020, 44.
4 Muckenhuber, S.; Holzer, H.; Bockaj, Z. Automotive Lidar Modelling Approach Based on Material Properties and Lidar Capabilities. Sensors, 2020.
Looking for a reprint of this article?
From high-res PDFs to custom plaques, order your copy today!