New Analytical Technique Uses Ultrasonic Spectrometry
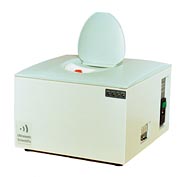
High-resolution ultrasonic spectroscopy (HR-US) is a new technique for material analysis. This technique is based on the measurements of velocity and attenuation on acoustical waves at high, ultrasonic frequencies propagating through materials. Optical transparency is not required, as ultrasonic waves propagate through opaque systems, thereby making the technique relevant for non-transparent as well as transparent samples. The technique provides a fast and non-destructive analysis of samples.
This technique was developed, patented and brought to the market by Dublin-based Ultrasonic Scientific Ltd. and was recognized with various international awards. This article explains the use of high-resolution ultrasonic spectroscopy in the coatings industry, and gives application examples of the new high-resolution ultrasonic spectrometers HR-US 101 and HR-US-102 for analysis of paint colloids.

Why Measure Paint with Ultrasound?
There are currently a number of techniques used by paint and ink manufacturers to analyze substrates, raw materials, fully formulated coatings, multi-layered coatings systems, and fibrous and particulate contaminants. These are used in developing new products, process control and quality control in production lines. The list of techniques includes fourier transform infrared spectroscopy (FTIR), attenuated total reflectance (ATR), X-ray photoelectron spectroscopy (XPS), ion mass spectrometry (SIMS), plasma-atomic emission spectroscopy (ICP-AES), X-ray fluorescence spectrometry (XRF), chromatography, optical microscopy, scanning electron microscopy-energy dispersive spectrometry (SEM-EDS), and rheological techniques.These techniques allow users to perform a variety of analytical tasks; however, one of the key tasks - non-destructive analysis of the interior and structure of paints and inks - is still a challenge. Paints are opaque and, therefore, light (electromagnetic waves) does not propagate through them. This limits the application of most traditional spectroscopic techniques for non-destructive analysis to the surface characteristics only.
The main advantage of ultrasonic spectroscopy is the ability of ultrasonic waves to propagate through paints. Paints are ultrasonically transparent; therefore, using ultrasonic techniques one can see through the paint samples, analyze their structure (and composition) and the variation with temperature, time, addition of ingredients, etc.
High-resolution ultrasonic spectroscopy offers new tools for developing new products and optimization of existing products, quality control, and process control in production lines. It provides greater productivity and cost saving for end-users, allowing customers to do a range of analyses that currently have to be done by several separate blocks of analysis. Other advantages include the following.
- Non destructive, non invasive analysis
Ability to analyze both diluted and concentrated samples
Ability to analyze intermolecular forces between the components of the sample, sample structures, composition, phase transition, etc.
Fast analysis and in-flow analysis
No sample preparation
Minimum calibration
No consumables needed
No associated health and safety requirements
Parameters Measured in HR-US
Ultrasonic spectroscopy is based on the measurements of two independent parameters, ultrasonic attenuation and ultrasonic velocity. Ultrasonic attenuation is determined by the energy losses in ultrasonic waves, and can be expressed in terms of viscosity of the medium or its longitudinal loss modulus. It allows the analysis of kinetics of fast chemical reactions, microstructure of materials including particle sizing, aggregation, gelation, crystallization and other processes and characteristics.Ultrasonic velocity is determined by the density and the elastic response of the sample to the oscillating pressure in the ultrasonic wave and thus can be expressed in terms of compressibility or storage modulus (longitudinal). This parameter is extremely sensitive to the molecular organization, composition and intermolecular interactions in the analyzed medium, and is responsible for the major portion of applications of high-resolution ultrasonic spectroscopy for analysis of chemical properties of materials. However, wide application of ultrasonic velocity requires extremely high resolution of the measurements, which was a problem in the past. HR-US spectrometers with their outstanding resolution (down to 10-5%) are the first commercial instruments, which allow users to enjoy the full potential of ultrasonic analysis.
Following are some examples of applications using high-resolution ultrasonic spectrometers.
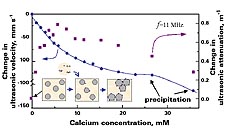
Stability of Paint Emulsions and Dispersions
An important application is the analysis of the stability of paint emulsions and dispersions. Many factors affect the stability of emulsions and dispersions. Temperature, aging or additives such as multivalent metal cations can induce destabilization, resulting in the irreversible changes of the product properties. An ideal method for analysis of stability, structure and shelf life of paints should be fast, reliable and non-invasive. This task is successfully performed by high-resolution ultrasonic spectroscopy.Examples of the ultrasonic measurement for the characterization of dispersion stability are given in Figures 1-2. Figure 1 illustrates an application of ultrasonic spectroscopy for analysis effects of temperature on stability of paint emulsions. Many companies estimate that hundreds of tons of material are being wastefully discarded due to premature disposal. Therefore, an accurate determination of the storage conditions and lifetime is important. Often indications of the shelf life are too conservative and cannot account for the varying storage conditions experienced by an individual item.
In the current example, the effect of heating and cooling (between 0.5?C and 50?C) on the stability of two different coating emulsions is monitored by change in ultrasonic velocity (upper curve) and attenuation lower curve. Reversible changes of ultrasonic parameters were observed in one of the emulsions (sample 1) during several consecutive cycles of heating and cooling between 0.5 deg C and 50 deg C. This indicates that this emulsion remains stable after the thermal treatment. In contrast, there is a sharp drop in ultrasonic velocity and attenuation in the second sample observed at the first heating cycle around 40?C, which is not recovered in subsequent ramps. This indicates the irreversible changes in the emulsion.
The decrease in ultrasonic parameters can be explained by the coagulation of the oil particles induced by the temperature. The aggregation of the original particles into larger particles following the phase separation between oil and water phases results in the diminishing of the scattering contributions to ultrasonic parameters: dispersion of ultrasonic velocity and ultrasonic attenuation. This is accompanied by a decrease in ultrasonic velocity and attenuation (see sample 2). The slope of temperature dependence of the velocity in the second emulsion changes the sign at a temperature around 35-38?C. After breakpoint, the temperature slope corresponds to one in oil phase, showing phase separation of the emulsion.
Figure 2 illustrates application of ultrasonic spectroscopy for the analysis of the calcium effect on the suspension of polystyrene particles. Interaction of calcium ions added to an aqueous suspension of polystyrene particles (0.5%) was analyzed by the change in ultrasonic velocity and attenuation, with the stepwise addition of calcium.
In this example, ultrasonic velocity and attenuation were measured by titrating with a concentrated solution of calcium cation. The added cations accumulate on the surface of particles. This binding is accompanied by the dehydration of the external layer of the particles, and results in a decrease in ultrasonic velocity. When the concentration of cations on the particle surface increases, it results in the destabilization of the dispersion. The aggregation of particles results in an increase of ultrasonic attenuation, while the precipitation of particle aggregates is indicated by the decrease of ultrasonic attenuation at high concentration of calcium added.
Characterization of Surfactants
Coatings formulations include "colloidal dispersions" of two immiscible phases (such as oil-water emulsions or dispersions of solid particles in water) or "association colloids" formed by self-assembly of soluble surfactants or polymers (such as pastes, inks, shampoos and foodstuffs). Because of the high surface-to-volume ratio of colloidal dispersions, the energetics of the interface plays a more important role in the phase behavior and flow behavior of complex fluids than in simple fluids. The behavior of multiphase media as a consequence of interparticle forces, microscopic transport phenomena and engineering processing can be studied with ultrasonic analysis. Applications include retarding phase separation (e.g., the formulation of paints and printing inks with a useful shelf-life); or, conversely, acceleration of phase separation.Interparticle (colloidal) forces are to complex fluids what intermolecular forces are to simple fluids: they are responsible for all physical properties and material behavior. Originated by the 'energy cost' of interfaces in colloids, these forces make colloids thermodynamically unstable. These forces bring the particles together to form larger structures like a floc. Formation of flocs (and phase separation) can be avoided by the addition of a surfactant, which adsorbs on the surface of particles to reduce the energy cost of the interface and also produce a repulsive colloidal force (steric repulsion or electrostatic repulsion). Such repulsive interactions impose an energy barrier, which delays the formation of flocs almost indefinitely, thereby lending kinetic stability to the dispersion or emulsion.
As well as preventing the formation of some structures (flocs), the addition of surfactants can cause other kinds of structures to form. Above a critical concentration, surfactant molecules tend to aggregate to form micelles. These micelles can solubilize otherwise insoluble components. Micelles can also transform a kinetically stable emulsion into a thermodynamically stable micro-emulsion. Alternatively, the presence of non-adsorbing micelles can produce depletion attraction between larger particles of the dispersed phase. This might destabilize a colloidal dispersion, thereby producing the opposite effect usually intended by the addition of surfactants.
HR-US is a powerful tool for the characterization of the surfactant and the analysis of processes involving surfactants. A range of information can be obtained about surfactant systems. In particular, the ultrasonic technique allows the measurement of critical micellar concentration (CMC) internal and macroscopic structure of surfactant self-assembly, micelle size, kinetics and thermodynamics of micellization and micellar transitions. Providing the range of parameters for surfactant micelles, HR-US goes further and allows the analysis of complex systems such as polymer-surfactant, surfactants in colloids and it gives information on internal-and macroscopic structure of complexes, particle size, colloid stability, kinetics of phase separation, critical aggregation concentration (CAC) and binding constants. Using one simple automatic titration regime you will have not only CMC, but also characterize the hydration of the surfactant in monomer form and in micelle, internal and macroscopic structure and kinetics of self-assembly (using multi-frequency mode), size of micelles and micellar transitions. In addition, with the convenient temperature ramps you can "see" thermal transitions in micelles, thermodynamic micellization (e.g., compressibility, or enthalpy of micellization derived from CMC obtained at different temperatures).
Figure 3 illustrates the ultrasonic analysis of micelle formation for three different surfactants that have positively charged heads and hydrophobic tails -(CH2)n-1CH3, where n is 12, 14 and 16. In this experiment, surfactant was added stepwise to water and concentration increment of ultrasonic velocity (relative difference between ultrasonic velocity in solution and water divided by concentration of surfactant) was measured. As different surfactants have different concentration increment of ultrasonic velocity, for comparison purposes only the change of the increment is plotted in Figure 3. As can be seen from the figure, at small concentrations the concentration increment of ultrasonic velocity stays constant, thus demonstrating the absence of interactions between molecules of surfactants. Beginning from some concentration of surfactant, the increment decreases with some saturation at high concentrations. The concentration at which the deviation accrues is CMC and is different for different surfactants. The total change in the concentration increment of ultrasonic velocity is determined by the elasticity of the hydrophobic core of the micelle. As can be evaluated from the curve, the elasticity (compressibility) of the core is proportional to the length of the hydrophobic tail of the surfactant.
Because this is a high-frequency rheological technique, ultrasonic spectroscopy allows the analysis of the sample structure, including particle sizing without dilutions as required by light scattering techniques. It also can measure concentrations of components in emulsion and suspensions. In opaque samples it allows analysis of the interior (bulk properties) of the samples in contrary to many optical techniques collecting the signal reflected by surface only.
Using ultrasonic instrumentation and technology provides greater productivity and cost saving for end-users, allowing the customer to do a range of analyses that previously had to be done by several separate blocks of analysis. The speed at which analysis can be performed, achieving an immediate response, is a strong advantage. The minimum amount of skilled intervention required at input and at output stage is highly attractive.
Conclusion
The technology breakthrough has resulted in Ultrasonic Scientific reaching previously unattained levels of resolution, which the company has brought to market as the high resolution ultrasonic spectrometer (HR-US), an instrument suitable for a variety of analytical applications in the coatings industry.For more information, contact Ultrasonic Scientific Ltd., e-mail info@ultrasonic-scientific.com or visit www.ultrasonic-scientific.com.
Key Characteristics of HR-US Spectrometers
1. High resolution (down to 10 -5% velocity - 0.2% attenuation) allows:- analysis of a variety of processes
- analysis of components at low concentration (down to 0.3 ppm)
- broad range of wavelength for high resolution probing of structures
- analysis of chemical processes across a broad range of relaxation times
- Temperature ramp - analysis of thermal transitions
- Kinetics - analysis of rate of chemical processes
- Titration - analysis of binding reactions, effects of composition on molecular behaviour
- Flow through - online application, e.g. HPLC detection, process control
- Multi-frequency - any of the above individual regimes can be conducted at various frequencies simultaneously.
- the low power ultrasonic waves applied to the sample do not effect the state of the sample
- allows analysis of samples across a broad temperature range
7. Physically and chemically stable sample cell:
- source and receiver ultrasonic sensors are not in direct contact with the sample
- eliminates degradation, contamination and misalignment of sensors enabling use of aggressive (and sticky/problematic) substances
- allows mechanical and chemical cleaning methods
- reduced sensitivity to external environment (mechanical vibrations, temperature changes, physical movements, etc.) with no realignment required
- sealable chamber eliminates evaporation
- optional stirring enables uniform mixing (measurements in sediment samples, titration measurements, etc.)
- standard 1ml cell
- customized cells of 30 deg l and above are available
- small sample size volume is ideal for expensive materials and enables good temperature controls
Looking for a reprint of this article?
From high-res PDFs to custom plaques, order your copy today!