New Mill Demonstrates Improved Quality
A number of industries are based on the development of products from particulate dispersions. In many cases, these industries are faced with the competitive need to develop increasingly finer dispersions having narrower particle size distributions. Ceramics, printing and ink jet inks and automotive OEM finish manufacturers are specific areas where this is true.
Automotive OEM manufacturers and their finish suppliers are extremely style oriented. Each OEM finish manufacturer attempts to develop unique new color effects annually to gain a larger share of the OEM business. While unique colored flake pigments have been introduced in this area in recent years and are becoming increasingly popular, they must be formulated with very high transparency pigment dispersions in order to take advantage of the multi-angle color variation inherent in these flake pigments. The need for very high transparency colored pigment dispersions has pushed the processing of these pigments very close to the limits of conventional fine media milling technology.
There is considerable evidence that existing fine media mills are operating close to their limits in making these highly transparent dispersions. This evidence is characterized by extended dispersion cycles for these pigments with very little quality improvement toward the end of the cycle. This indicates that the product quality of these dispersions is close to the maximum product quality that can be achieved by these mills under their existing operating conditions. Increasing the agitator tip speed of these mills to increase the maximum shear level in the mill would result in a higher product quality. However, most fine media mills that make these transparent dispersions are operating at or near their maximum recommended agitator tip speed, beyond which mill wear could become excessive.

Because of the longer grinding cycles inherent in producing finer particle size dispersions under existing conditions, smaller, more uniform, spherical media have been used in fine media mills at concentrations that allow the generation of higher maximum shear levels and, thus, result in a smaller particle size, leading to a more transparent product. This push for the use of finer media is again evidence that the existing mills are operating at or near their maximum capability.
The comparatively recent emphasis on the circulation grinding process for media mills has helped the situation, in that circulation milling normally produces many more passages of the product through the mill in a given dispersion cycle. This results in a narrower residence time distribution in the mill, which translates to a narrower particle size distribution of the product. However, circulation milling by itself does not increase the maximum shear in the media mill and, therefore, there is little, if any, change in the maximum product quality inherent in its use.
In many cases, once the mill optimization has occurred, increases in the requirement for product quality have resulted in operation of the optimized media mill under conditions close to their operating limits. Based on historical demands for increased product quality, there is no doubt that further improvement will be required. The QMAX SupermillT was designed to provide a quality maximum significantly above that available with existing mills when operated under the same conditions.

The QMAX Series Supermill
In the design phase of the QMAX Supermill, Premier Mill extensively reviewed the capabilities of both the existing Supermills and our perspective of the future product quality requirements to be faced by fine media mills. We used for guidance a number of customer tests, including many where Supermills were compared with a range of competitive media mills. Our analysis indicated that, while some of the competitive mills were more productive than the Supermill on a mill volume basis, the ultimate product quality attainable with all media mills was comparable -- e.g., the maximum product quality produced by all tested mills, using the same operating conditions, was essentially the same, although some mills achieved this maximum quality with less residence time than others.Based on this evaluation, we identified and prioritized the design characteristics for the new mill.

The use of these AP discs resulted in a significant increase in the maximum product quality obtainable by a media mill when compared with an identical mill operated under the same conditions of agitator speed, and media type size and load. The OPTIMIZE model indicated that the primary AP disc mechanism was the acceleration of the mill media to a velocity far beyond that normally obtained with a disc. Our calculations indicated that, with the AP disc, the velocity of the accelerated media was essentially double that obtained with a standard disc of the same dimensions. This resulted primarily in an increase in the maximum level of shear available in the mill with a consequent improvement in maximum product quality and dispersion rate.
These AP discs (patent pending) also consumed more power than the standard discs, requiring an improvement in our normal cooling capability through design changes. To meet our objective of an increased product flow rate for circulation milling, the shell design was changed relative to previous Supermills of similar volume, to provide more area for product flow and more cooling surface area per unit volume. A newly designed screen module was developed to allow the handling of ultrafine media to a size as small as 0.1mm.
Operation of the QMAX series Supermill is enhanced by the use of a computer controlled startup and operation to provide precise control of process variables due to the high energy capability of the AP discs in a small volume mill. The use of this computer ensures that the system will automatically adjust to the operating conditions required for stable processing. The mill also requires efficient cooling in the product recirculating tank and lines to remove the process heat exiting the mill as an increased product temperature and insure processing stability.
A range of QMAX series Supermills is available for both laboratory and commercial use (see Figure 3). These mills are designed to use the A6P discs which require a high power input and, thus, cannot be used in conventional Supermills. A listing of specifications is given in Figure 4.

Testing the QMAX Supermill
Using the A6P disc, comparison runs were made vs. our existing standard discs, with the same number of each disc type, under identical conditions of agitator tip speed, media type, size and load. A typical result is shown in Figure 5 for the 20% calcium carbonate slurry dispersion. In this example, the QM-10 mill was operated at an agitator tip speed of 2,700 FPM, with an 80% media load of 0.7-1.2 mm Zirconox media. The circulation process was used with a 119 liter batch size and a circulation rate of 240 GPH for seven hours. The circulation rate was limited by the standard discs, which are more sensitive to hydraulic media packing.The results of this comparison illustrate the superiority of the AP disc concept. The batch processed using the existing Supermill standard discs had a measured product quality vs. residence time curvefit QMAX median particle size of 0.503 microns on a volume basis. The batch processed using the A6P discs had a measured product quality curvefit QMAX value of only 0.310 microns. This is a significant improvement in the measured QMAX value achievable under the same operating conditions. Use of the OPTIMIZE model allowed us to define the effective agitator tip speed of the A6P disc mill at over 5000 FPM -- i.e., the QMAX obtained with the AP discs, operated at a tip speed of 2,700 FPM, were equivalent to that which would be obtained with standard discs operated at a tip speed in excess of 5,000 FPM. It is the higher maximum shear obtained under these conditions that results in the significant increase in the maximum product quality achievable in the A6P disc mill.
In other industries demanding ultrafine pigment dispersions, such as automotive OEM and inkjet applications, a series of tests was run to define what the AP discs could achieve. In each case, the QM mill with A6P discs exceeded the quality currently being produced by that customer on his Supermill or using a competitive mill. A number of tests were carried out with customer supplied materials. The results were analyzed using OPTIMIZE and substantiated the results obtained from the calcium carbonate tests and verified the postulated dispersion mechanism.
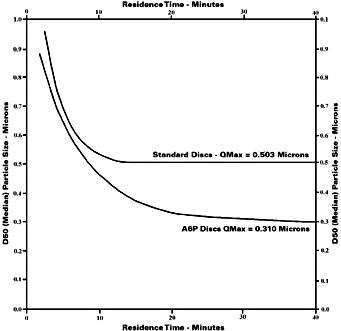
Predicted Results from the OPTIMIZE Model
The advantages of using the A6P disc mill over existing mills can be seen in the example in Figure 6. This figure illustrates the situation discussed at the start of this article, namely a situation where the existing mill has been optimized for an existing product and, because the required product quality has increased to the point where it almost matches the available QMAX, no further improvement can be anticipated.
This figure compares two otherwise identical mills, one using the existing standard Supermill discs and the other using the A6P discs and operated under identical conditions. The current disc technology mill will produce the existing product at a residence time of 28.43 minutes, but cannot increase the product quality beyond the current standard. The use of the QMAX Supermill will produce the same product quality at a residence time cycle of 10.99 minutes but, more importantly, can produce a product having a quality increase of up to 105% of the current standard in less residence time than the current, lower quality, product. This quality improvement cannot be matched by existing mills for, if changing the existing mill operating conditions will increase the ultimate product quality, our tests show that operating the QMAX Supermill, under the same conditions, will continue to significantly exceed the product quality achievable with the existing mill.

Conclusion
The QM mill demonstrates the capability to produce higher transparency dispersions, which should allow enhanced styling effects in automotive OEM finishes and printing inks. In ceramics, the AP disc technology can produce finer particle size ceramics, generate nano size particles with narrow distributions and in electronic printing inks, the finer particle size attainable via this mill could allow even finer printed circuits to be developed.Zirconox is a registered trade name of Jyoti Ceramic Inds. P. Ltd. for Ceria stabilized Zirconium Oxide.
For more information, contact Premier Mill, a Lightnin Co., Exeter Industrial Park, One Birchmont Drive, Reading, PA 19606-3298; phone 610/779.9500; fax 610/779.9666; or Circle Number 141.
Reference
1 Edwards, W. H. - "Fine Media Mill Dispersion" ; Seminars in Science and Technology - Pigments Dispersion: Science and Technology; The Institute of Materials Science - New Paltz.The OPTIMIZE Mathematical Dispersion Model
The OPTIMIZE mathematical dispersion model has been used for some time for predicting the effect of changing the operating conditions used for making a dispersion. Its accuracy also allows the evaluation of new mill and agitator designs without necessarily having to build them. OPTIMIZE was used to calculate the effect of mill configuration and operating condition changes for this program. The model requires input of product quality vs. average residence time data for a given product batch and the definition of the conditions under which it was made. The basic quality vs. residence time equation has been previously published.1 The OPTIMIZE version of this equation is:This relationship is shown in Figure 1.
Note that the finite values of QMAX and QSTART are computed by the curve fitting segment of the model and are not entered as data. QSTART can be measured when particle size, transparency or tinting strength are used as the quality measurement, but the clumps of pigment inherent in the premix sample result in large measurement errors. QMAX, by definition, is the maximum product quality obtainable with a specific media mill, operated under a set of defined conditions for an infinite residence time. For this reason, we prefer to extrapolate the curve fit to determine the values of QSTART and QMAX. Note that the product quality vs. residence time curve will change with any change in operating conditions. The RATE and QMAX values used in the model are affected by changes in the mill configuration and the mill operating conditions. The value of the OPTIMIZE model is that it can predict the effect of these changes and redefine the product quality vs. residence time curve for the revised conditions or a different mill.
Significance of QMAX
Actual product quality measurements are normally based upon physical measurements of dispersion samples. These measurements include particle size, transparency, and absorption and scattering coefficients. The OPTIMIZE model converts these physical measurements into a product quality index in which the selected quality standard is given a value of 100%. QMAX is defined as a quality value relative to that of the standard and is given as a percentage of the standard.
As mentioned earlier, one of the characteristics of the dispersion industry is that, for certain end uses, the requirements for product quality usually increase with time. Figure 2 is a typical product quality vs. residence time curve, where the original product standard was produced at a residence time of 15 minutes and valued at 100%. The maximum quality (QMAX) attainable using the mill operating conditions is 5% higher in measured quality than the standard and, thus, has a product quality of 105% relative to that of the standard. The requirement for an increase in the dispersion quality results in the selection of a new quality standard, using the same mill operating conditions and, therefore, having the same product quality vs. residence time curve but requiring a residence time of 17.88 minutes to produce the new quality standard. This new standard now is assigned the standard value of 100% and the QMAX, which has an unchanged finite value, is only 3% higher than this new standard and, modelwise, has a relative value of 103% of the standard.
Further increases in the required product standard quality, without a change in the mill operating conditions, will narrow the difference between the increased product quality standard and the QMAX, until the product quality standard is virtually equal to the finite QMAX of the quality vs. residence time curve. Assuming that the relative QMAX value is only 0.5% higher than the newest standard, the QMAX, which is unchanged in measured quality value, now has a relative value of 100.5% of the newest standard. The newest product quality standard, produced under the same operating conditions as the original standard, now requires a residence time of 28.21 minutes. Further improvements in the standard product quality using the same process operating conditions are impossible, since QMAX represents the maximum quality attainable under these operating conditions.
The obvious answer to increasing the product quality further is to change the process operating conditions to provide a new product quality vs. residence time curve having a higher measured QMAX value. However, if the mill operating conditions have already been optimized in producing the original product quality vs. residence time curve, further improvement in the product quality is not possible with the existing mill. Therefore, a new mill capable of higher shear and providing a higher QMAX value under the same operating conditions is required to meet future quality requirements.
Looking for a reprint of this article?
From high-res PDFs to custom plaques, order your copy today!