Changing of the Guard: Switching to Smart Pigments for Corrosion Protection

To create a truly smart coating you need to start with smart additives. Like a good cook you need the best ingredients. The expertise of paint and coating manufacturers resides in the formulation of good and reliable products to serve their customers. They are not in the game of developing new raw materials but rely on their suppliers to bring them innovative ideas and products.
The knowledge and innovation required for the development of truly new smart additives often lies outside of the paint and coatings industry. Ceramisphere’s innovation and addition to this market (i.e. matrix encapsulation) originates from the nuclear and pharmaceutical industries, leveraging technology utilized in one area and making it applicable to another.
Microencapsulation (Core-Shell vs Matrix)
Microencapsulation is the process of entrapping a solid, liquid or gas inside a particle. The particle has the function of releasing and/or protecting the encapsulated material under specific conditions (e.g., sunlight, oxidation, pH).
There are a variety of microencapsulation geometries, the most common being core-shell microencapsulation. This technology has been leveraged by other companies for use in the paint and coatings industry.1-3 In this type of encapsulation there is a clear distinction between the external shell (protection layer) and the internal core (payload). The geometry of the particles is best represented by an eggshell. The egg, in this case, can be ruptured either physically (pressure, shear stress) or chemically (heating, hydrolysis, dissolution). However, once cracked the contents are released immediately in what is termed a “crash” or instant release. This is useful when release is required with immediate effect of the payload under a triggered condition (e.g., a pH change due to corrosion hydrolyzes the shell and releases an inhibitor or indicator). However, this form of encapsulation is not well suited to provide a constant release over a long time period. Once emptied of their payload, the capsules leave a significant void in the coating, which increases the overall porosity and weakens the physical and mechanical properties of the coating.
The use of matrix encapsulation is less common. Matrix encapsulation involves the entrapment of the active payload inside a three-dimensional polymeric network synthesized in situ. In the case of Inhibispheres®, silanol precursor monomers are used to form silica and hybrid silica polymers. As the polymerization proceeds, a 3D silica (Si-O-Si) network is formed, entrapping the corrosion inhibitors inside the pores of the network, like fish inside a net. An emulsion polymerization process is used to enable the segregation of this polymerization reaction inside the emulsion droplets. The droplet acts as a micro- or nano-reactor in which the silane polycondensation proceeds until gelation, i.e. the whole droplet is occupied by the silica 3D network containing the inhibitor inside its pores. Careful control of the precursors and the reaction conditions used to form the emulsion allows for a great degree of control over the size of the droplet, and thus the size of the particles. In contrast to the core-shell model, matrix encapsulation is closer to a sponge, where the pores in the sponge are filled with the encapsulated material. The payload is released slowly over time by diffusion through the pores of the matrix. Unlike core-shell capsules, matrix particles do not leave a significant void in the coating once the payload is depleted, as the matrix particles remain in place. The mechanical and physical barrier properties of the coating are maintained.
When comparing these two types of encapsulation (Table 1 and Figure 1) it is clear that the sponge, unlike the egg shell, can be broken down mechanically without sacrificing functionality. If a sponge is broken in two, it produces two smaller sponges. A broken egg leaves a mess. The advantages for matrix-style encapsulation over core-shell are obvious (Table 1).
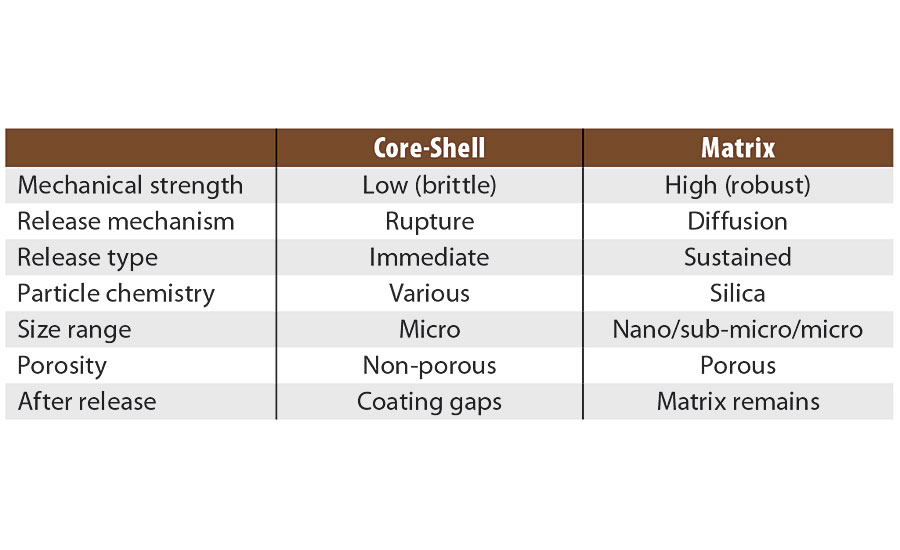
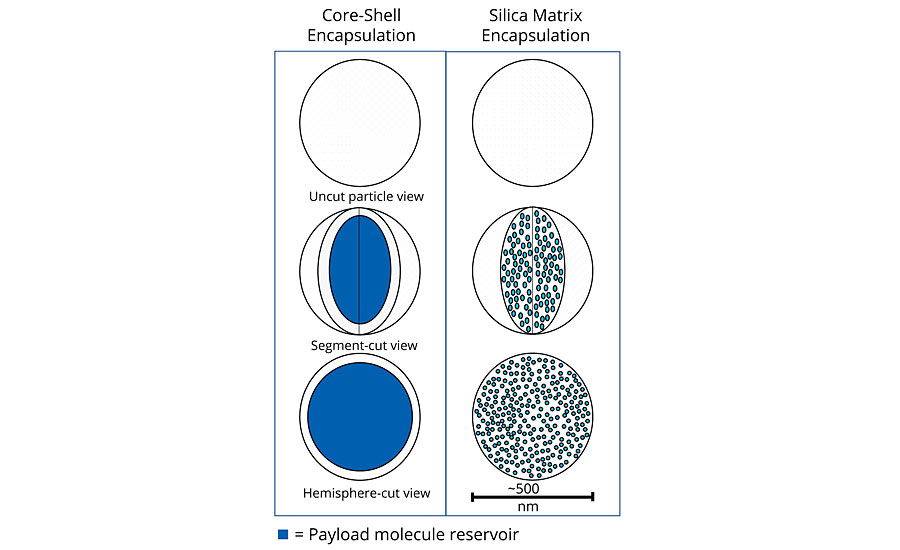
Physical/Mechanical Properties
The microparticles resulting from the matrix encapsulation are mechanically resistant. They can be broken down into smaller particles, without affecting the release rate of the encapsulated material. To demonstrate this, a dye (Rhodamine) was encapsulated inside silica microparticles, and its release rate was measured over time. Some of the particles were taken and crushed to reduce the particle size, and their release rate was measured under the same conditions. The comparison of the fraction of dye released for each sample is provided in Figure 2. There is little difference (<5%) between the release from non-crushed and crushed particles over the course of 24 hrs.
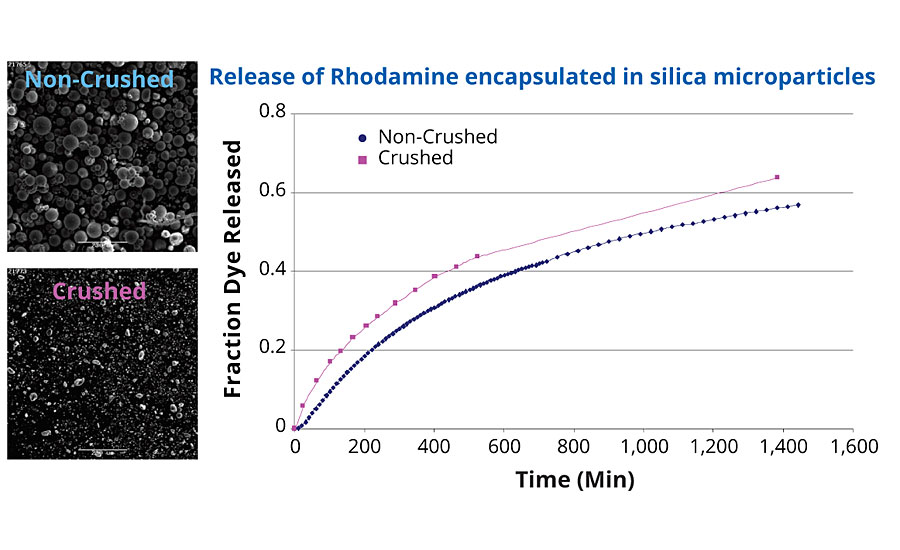
Paint Compatibility
The components required to achieve all the desired properties of a paint are in constant balance. Organic corrosion inhibitors have a tendency to upset this delicate balance. Most organic inhibitors are incompatible with paint chemistry, causing either phase separation or incomplete/premature curing.
Encapsulation within a matrix can help to compatibilize materials normally considered incompatible. For example, an organic corrosion inhibitor was mixed into an epoxy paint resin pack. The mixture was left at 40 degrees for a month for accelerated aging. The same inhibitor was encapsulated using the equivalent amount of inhibitor in the same epoxy resin pack, and the same test was performed. The images show that the encapsulated material didn’t cure the epoxy (Figure 3), while the unencapsulated material did. The inhibitor was an organic material with chemistry and functional groups capable of reacting with the epoxy group.
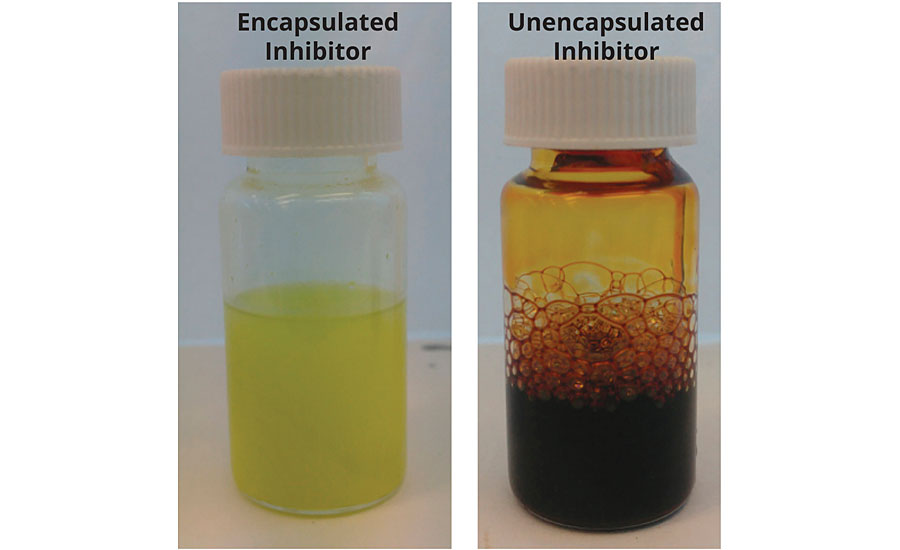
Particle Size Control
The control of pigment particle size is essential to obtain the desired properties and physical characteristics of any coating. Particle size also controls the optical properties in the coating (matte, gloss, lightfastness, color). Moreover, the shape and size of a pigment will determine the rate of penetration of moisture inside the coating, which leads to corrosion of the metal surface.
Through extensive research and development over the last 20 years, Ceramisphere can precisely control the particle size of its products. The particles can vary over three orders of magnitude, from 0.1 to 100 μm (Figure 4). In general, submicron particles are preferred, as they are compatible with most coating thicknesses and do not affect the gloss.
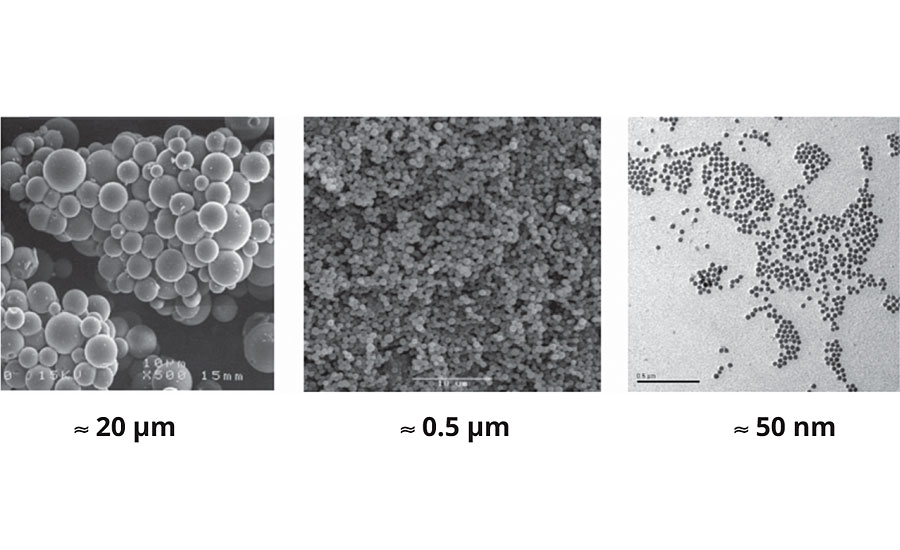
Controlled Release
Through careful selection of the precursor materials and the parameters controlling the polymerization in emulsion, the factors affecting release can be controlled. These include the particle size, the pore size and the internal chemistry of the pores. Figure 5 shows a schematic for how the inhibitors are released from the particles.
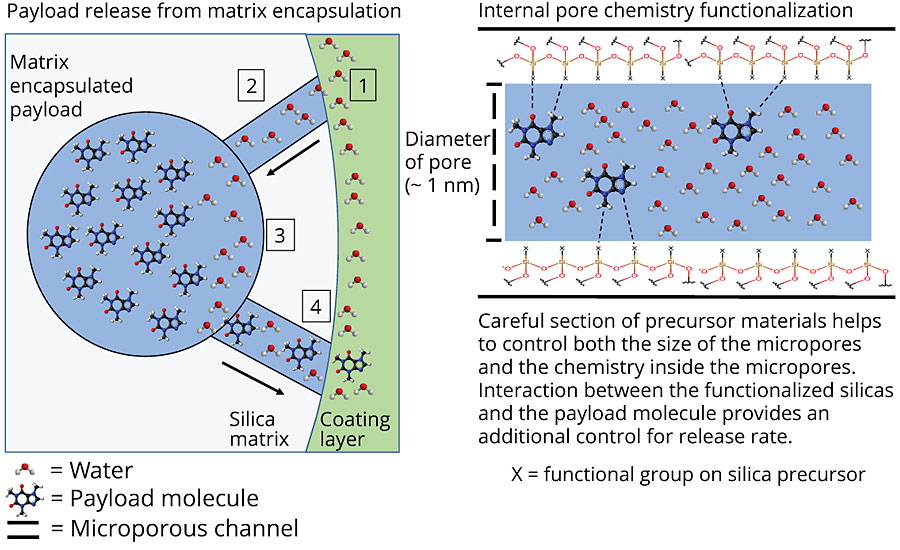
The internal chemistry of the pores can interact with the payload molecule (in a way similar to the packing in a HPLC column). This can be tailored to either slow down or speed up the release of a molecule. Figure 6 shows the release profile from two different matrices. The payload molecule in both cases is the same. The difference between the two is the internal chemistry of the pores as determined by the silanol precursors. The fast-release matrix has a low affinity for the payload molecule and releases 100% of the payload in just over a day. The slower-release matrix only releases 40% of the payload in the same time period. Even after seven days, the slow-release matrix has only released 60% of the payload.
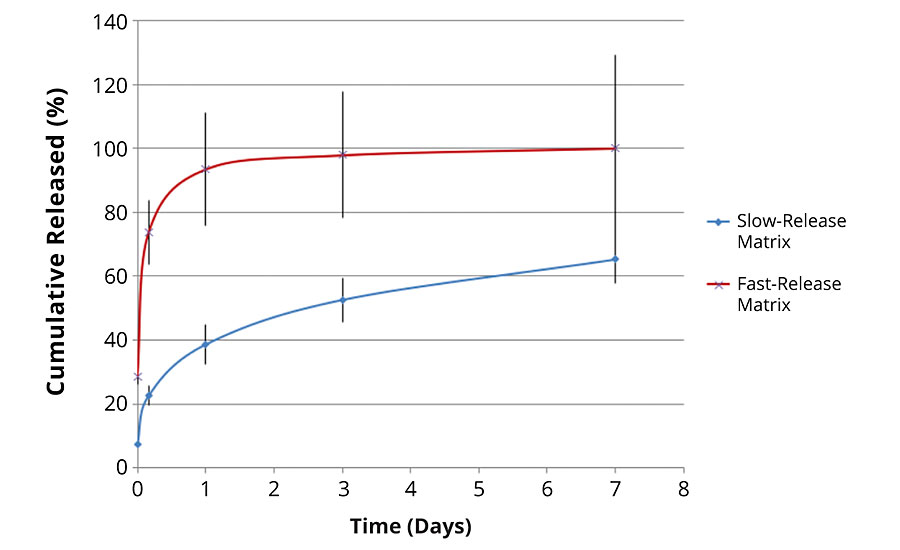
A similar experiment shows the release of a payload molecule from particles with two different pore sizes. The micro and mesoporous systems show different release rates. Over the course of one day you can see that the mesoporous system releases nearly 60% of its payload. The microporous system only releases about 10% in the same time frame. The difference is in the size of the pores alone (Figure 7).
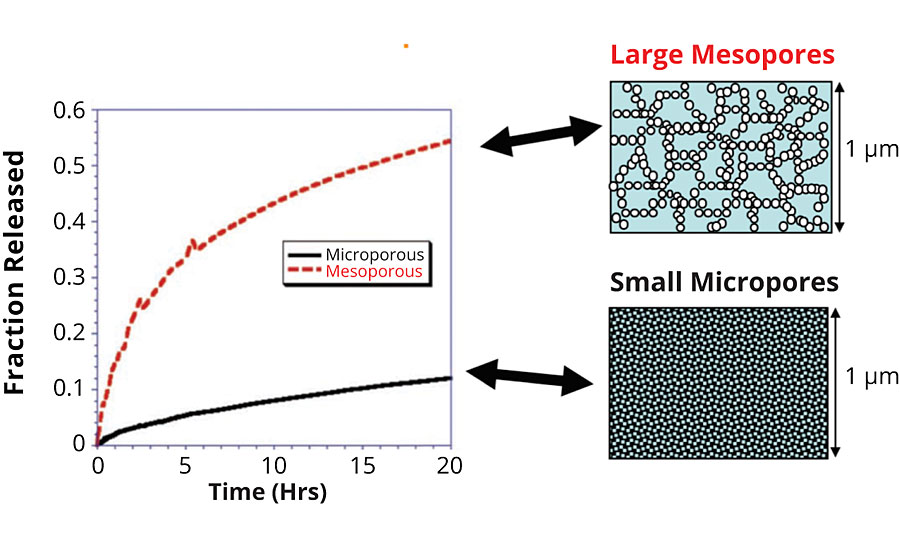
Using these methods, it is possible to tailor the release rate for a product to its required application. The key advantage over traditional corrosion inhibitors is the ability to release an inhibitor at a constant rate over the lifetime of the coating. Figure 8 shows a curve that is representative of the dosing mechanism for a traditional unencapsulated corrosion inhibitor in comparison to an encapsulated inhibitor with a controlled release mechanism like Inhibispheres. Initially the dose is high for the traditional inhibitor, but the material is used up, and a constant level of protection can’t be maintained over the lifetime of the coating. Additionally, when the traditional inhibitor is depleted it leaves behind voids in the coating, which can lead to catastrophic coating failure due to a significant increase in the coating porosity. The tailoring of the encapsulated inhibitor release rate means that a constant release can be achieved and an efficacious dose can be delivered over the full lifetime of the coating. In other words, a sustained release system, such as the Inhibispheres, provides a much more efficient use of the active material (e.g. inhibitor).
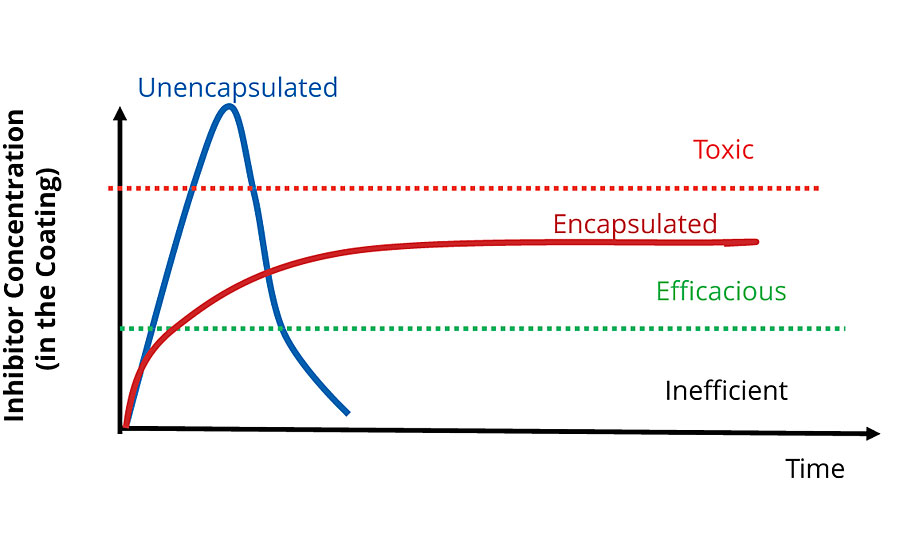
Mode of Action
Inhibispheres can be dispersed in a coating by milling in a basket mill or a bead mill with an appropriate dispersant for a liquid coating or in an extruder for powder coating. The particles themselves are essentially silicas or hybrid silicas. Once evenly dispersed in a coating, the Inhibispheres are activated by the presence of moisture. In general, without moisture there can be no corrosion. Moisture can reach the surface of the substrate by either penetration thought the coating or rupture in the coating.
The inhibitors used inside the Inhibispheres are organic or organometallic in nature. Their mode of action works differently than traditional corrosion pigments. The organic molecules are slowly solubilized and move with the solvent front to the metal surface. Once on the metal surface (Figure 9) the inhibitors typically prevent attack on the metal surface by corrosion initiators at the anodic site (e.g. Cl-, SO42-, H2O).
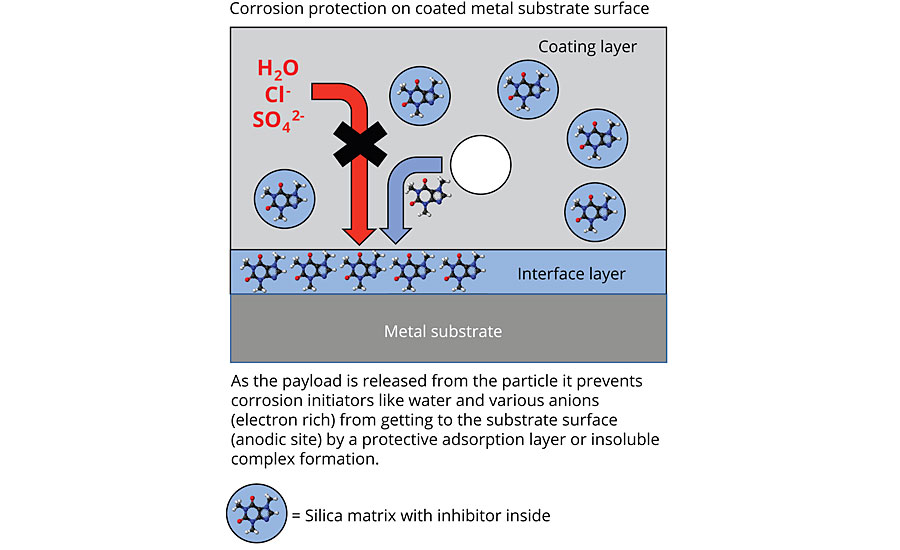
Performance
The true test of a smart additive is how it performs against the incumbent technology. Inhibispheres have been used in a number of different scenarios to explore their potential for corrosion protection.
Traditional Inhibitor Comparison
A comparison of the performance of the smart inhibitors was conducted against a traditional corrosion inhibitor.
Cold rolled mild steel was coated with a high-gloss solvent-based 2K epoxy. Strontium zirconium phosphosilicate (SZP) was added to the coating at 10% by weight loading and built up to a coating thickness of 85 μm. Using 2% of Inhibispheres A in the same coating type, the coating was built up to 60 μm. The addition of the smart inhibitor had no detrimental effect on the gloss of the coating due to the small particle size (D50 = 0.5 μm) in stark contrast to the SZP, which reduced the gloss of the coating.
Both panels were scribed with an X after curing and added to a salt spray chamber for 1,000 hrs. After 1,000 hrs in the booth the panels were removed and the coating was peeled off to examine the corrosion performance underneath, as well as creep along the scribe (Table 2). The creep is significantly reduced by the use of the Inhibispheres in the coating by comparison with the traditional SZP type corrosion inhibitor. A measure of the average of the corrosion creep as well as the longest creep from the scribe for each panel are also shown in Table 2. Inhibispheres particles were composed of 15% inhibitor by weight. The loading in the coating of the Inhibispheres particles was 2%, so the total weight of inhibitor in the coating was 0.3% by weight. The 0.3% of the organic inhibitor in the smart Inhibispheres particle outperformed the 10% of the SZP traditional corrosion inhibitor over the course of the 1,000 hrs of corrosion testing. The organic inhibitor is so effective that in some parts the scribe (bottom right branch of the X) at 1,000 hours, the effective corrosion creep is 0 mm.
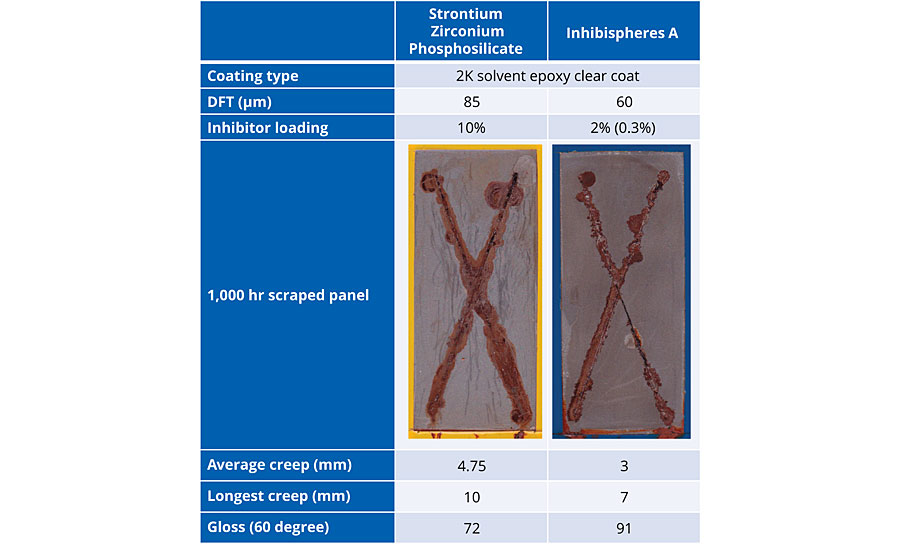
Dose Response Testing
A high-build alkyd primer was used to test the impact of increasing dosage of Inhibispheres. The materials were added to the primer at different concentrations to evaluate the effect on the corrosion performance. The test was performed on sandblasted cold rolled mild steel (EN10130). The panels were coated with a single coat to a DFT = ~75 μm. The coated panels were added to a salt spray chamber for testing with ASTM B117. The panels were unscribed. The original primer already contains a zinc phosphate corrosion inhibitor as well as micaceous iron oxide for corrosion control. The corrosion performance of these coatings can be seen in Table 3 after 500 hrs testing.
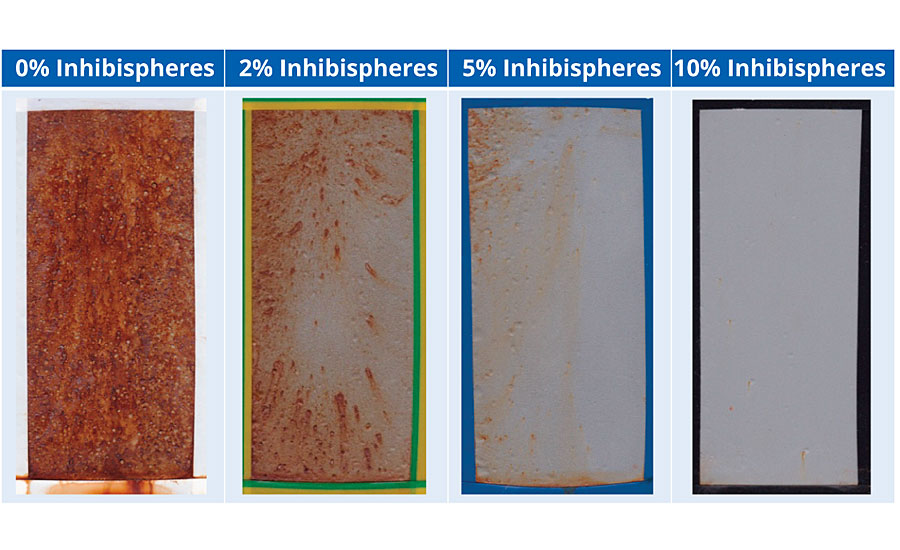
The addition of the Inhibispheres into the coating is displaying a typical dose response. The more Inhibispheres present in the coating, the less corrosion is seen on the test panels. The release of the active is having a significant effect on reducing the corrosion seen in the field of the coating. The smart Inhibispheres are so effective that at a loading of 10% there is almost no indication of corrosion seen on the test panel.
Improved Corrosion Rating
The Inhibispheres can also be used to improve the corrosion rating of a coating system containing traditional corrosion inhibitors by improving the corrosion performance and extending the corrosion protection in time above what would be traditionally expected.
A two-coat corrosion system was rated for a C3 corrosive environment.4-6 The two-coat system is made up of a zinc-rich epoxy primer with a polyurethane topcoat. Inhibispheres A was added to the primer layer of the coating system and compared against the original. In addition to this, a three-coat system was also prepared that is rated for C5M or C5I corrosion environment. The primer and topcoat in the three-coat system are the same as those in the two-coat system. The additional layer is an epoxy build layer to increase the thickness and reduce the porosity of the coating system (Figure 10).
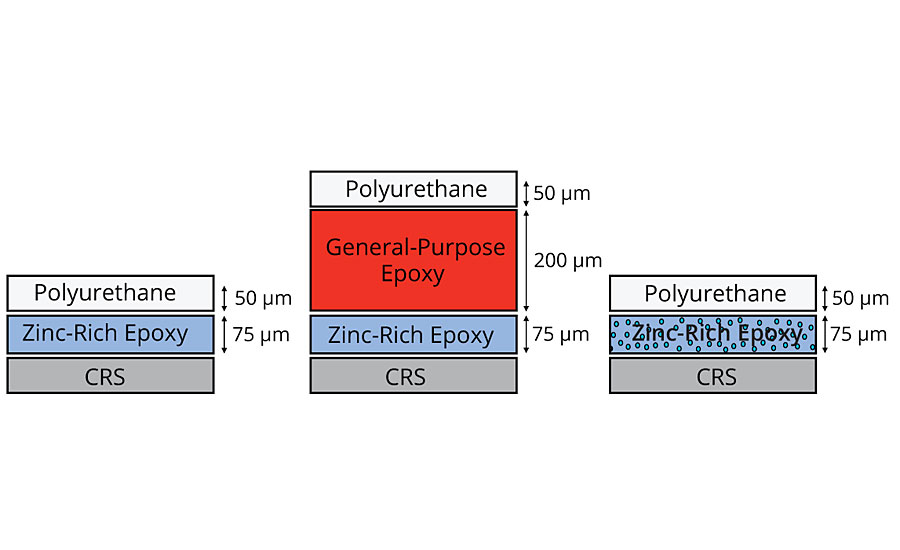
The panels were added to a salt spray chamber (ASTM B117) and exposed for 1,000 hrs. The corrosion test panels can be seen in Table 4 with the coating removed around the scribe. The two-coat control panel had the worst performance, followed by the three-coat panel. The best performance is seen with the two-coat corrosion panel with Inhibispheres in the primer. This demonstrates that a small addition (2 wt%) of Inhibispheres to a primer can potentially improve the coating’s corrosion rating from class C3 to C5 or greater.
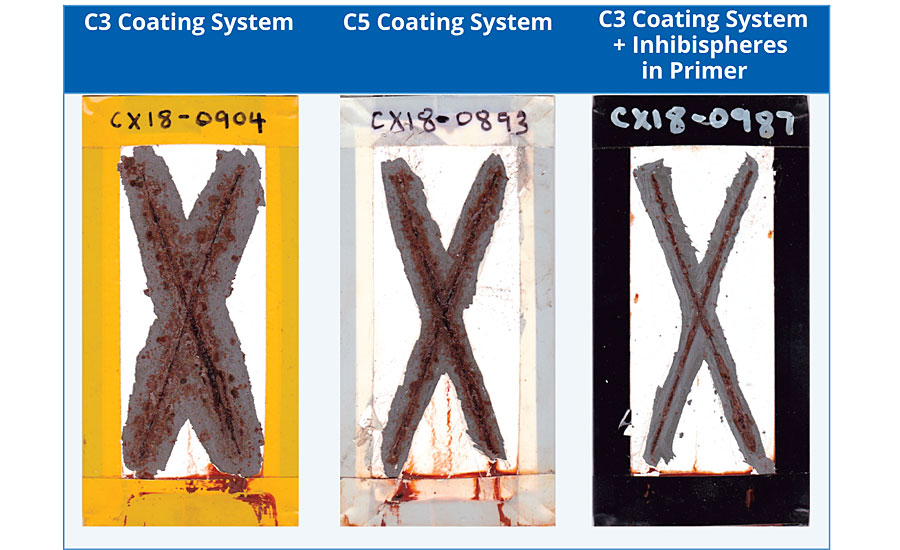
Conclusions
Inhibispheres are a unique product in the coatings industry. They represent a step change in the market for paint and coatings formulators and manufacturers. Traditional corrosion inhibitors cannot compete with these smart additives.
While Inhibispheres are currently focused on controlled release of corrosion inhibitors for the protection of ferrous and non-ferrous substrates in paints, coatings, concretes, rubbers and adhesives, the nature of the encapsulated material can be easily changed. For industrial purposes the obvious types of molecules are corrosion inhibitors. However, as you extend the protection of the payload and/or controlled release aspect of microencapsulation, you can look to a future that includes biocides, effect pigments, antioxidants, flame retardants, catalysts and colors, just to name a few. This form of encapsulation can help compatibilize molecules and pigments that would be difficult to incorporate in specific coatings formulations (e.g. aluminium pigment in water-based systems, in-can biocide release, organic color pigments in concrete). The encapsulation technology makes them accessible to formulators.
The future applications of those smart additives are only limited by the formulator’s imagination.
References
1 Lalgudi, R. Paint and Coating Industry Magazine, Nov. 2017, pg 28-31.
2 Lalgudi, R.; Cain, R.; Muzynski, B. Paint and Coating Industry Magazine, March 2016, pg 40-46.
3 Wilson, G.; Andersson, H.M. Paint and Coating Industry Magazine, May 2012, pg 58-60.
4 BS EN ISO 12944 - Paints and varnishes corrosion protection of steel structures by protective paint systems.
5 ISO 9223 - Corrosion of metals and alloys – Corrosivity of atmospheres – Classification.
6 AS/NZS 2312:2002 - Guide to the protection of structural steel against atmospheric corrosion by the use of protective coatings.
Looking for a reprint of this article?
From high-res PDFs to custom plaques, order your copy today!