A Versatile Polymer with Self-Healing Properties

Mercaptan (SH) or epoxy-terminated, highly reactive Thioplast polysulfide polymers are known for their chemical and solvent resistivity, low-temperature flexibility, high impact resistance and gas barrier properties. These polymers are often used in adhesives, sealants and coatings utilized in insulating glass windows, in construction or the aerospace industry. Sealant and adhesives producers like them for their easy processability and their good adhesion properties. One of the most fascinating features, however, is their self-healing capability.
These polysulfide polymers owe most of the above-mentioned properties to their high sulfur content. The sulfur is incorporated into the polymer backbone during the water-based emulsion polymerization process, in which di- and tri-halogen compounds react in a polycondensation reaction with inorganic sodium-polysulfide. During this process disulfide bridges are formed (Figure 1).
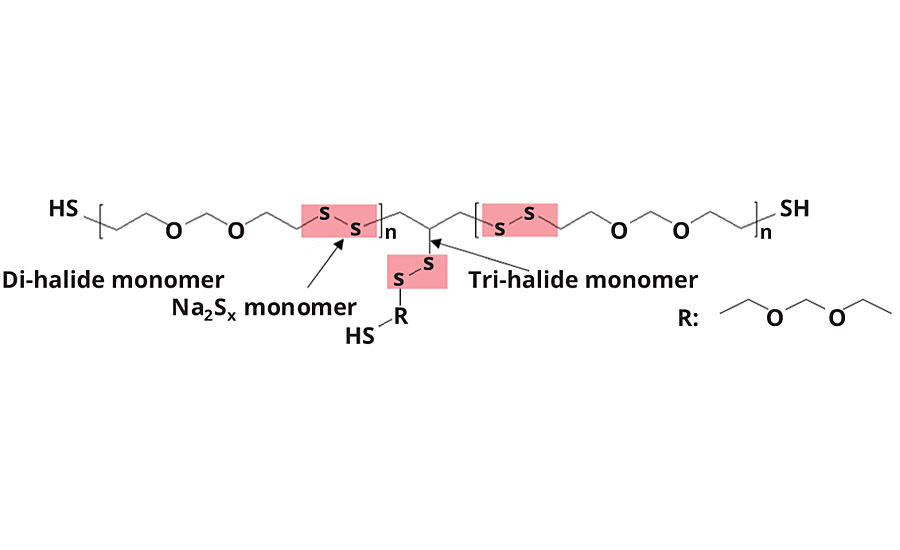
Disulfide linkages are very stable on the one hand (3rd highest bonding energy of all homonuclear bonds), and very flexible on the other (Steudel, 1975). Most remarkably is the fact that disulfide bonds can undergo exchange reactions at moderate temperatures. This allows polysulfide polymers to heal physical damages such as cracks or scratches on their own.
Self-Healing Materials
Artificial materials with an intrinsic ability to automatically repair damage caused by external physical action is not entirely new. The first self-healing materials were invented back in the time of the Roman Empire, when the Romans discovered a cementitious mixture that was capable of withstanding sea water and stopped cracks from growing. It took almost 2,000 years for mankind to build the first mechanical self-healing element. The element was designed in the 1970s and has been built for an undertaking where repairs could not easily be conducted – space exploration (Wool, 2008). From this, a new era of self-healing materials began, and today we are in the midst of it. The potential for the industry is enormously high.
Self-healing materials can be produced from a broad range of materials, e.g. mortars, metals or ceramics. The largest, and probably most important share of all materials projected to let the vision of self-healing materials come true are polymers of all kinds. Today, there are two groups of polymeric materials that are regarded as self-healing materials. The one group carries repairing agents encapsulated in its matrix, the other group brings the ability of repairing itself in its backbone. The second class represents the more “elegant” way of the two self-repair-mechanisms. Polysulfides belong to the latter.
Self-Healing in Polysulfides
Liquid Thioplast polysulfide polymers are available in many different grades, varying in their degree of branching, viscosity, molecular weight and SH content. All grades, however, feature sulfur linkages in their backbones. Due to these disulfide bridges, it is possible to mix two different polysulfide grades and – after a while – an entirely new polymer grade is formed (being a mixture of the branching degree, viscosity and molecular weight of the two parental polysulfide polymer grades). Thus, formulators can adjust the resin in their formulation to their desired properties. The underlying mechanism is not only of practical use for formulators searching for the ideal binder material viscosity, it is also enabling polysulfides to self-heal in the cured state.
In a crosslinked system, there are abundant disulfide bridges in spatial proximity. The right conditions will stimulate the sulfur bridges to open and find a new bonding partner close by. When the bonding partner is part of the backbone of a neighboring polymer chain, a new polymer chain is formed (Figure 2). This process is based on the so-called “Sulfur Exchange Reaction”.
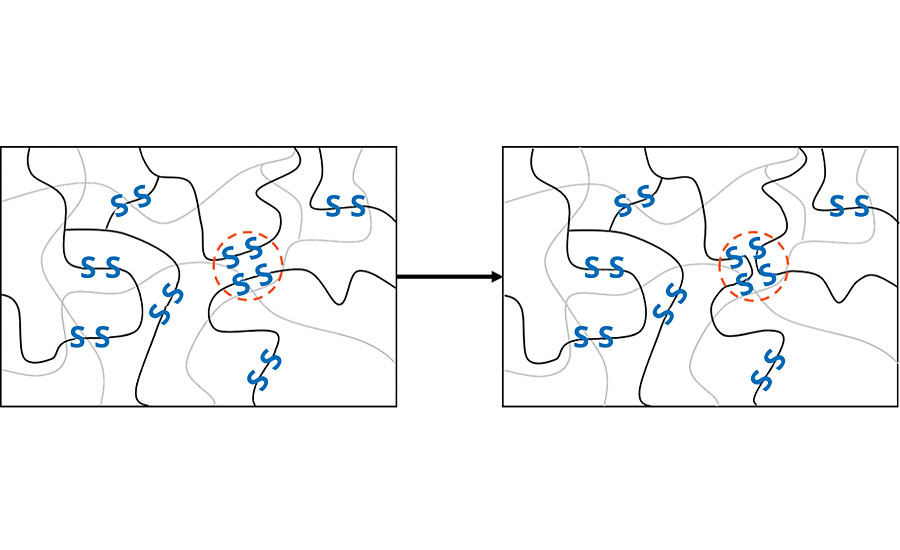
The timescale in which such intrinsic self-healing processes occurs is closely related to the glass transition temperature (Tg) of the polymer, which indicates the temperature above which polymer chains can move. Below this temperature, the movement of the polymer is completely frozen, and the material becomes brittle. So, when it comes to self-healing, a low Tg is favorable. In DSC measurements, polysulfides show a Tg around -55°C, which ensures good self-healing abilities even at very low temperatures. Another factor influencing the efficiency of the exchange reactions is the pH value. The highest exchange reaction rate is reached in an amino-basic environment.
These theoretical considerations are of very practical use, and naturally, this phenomenon has raised the attention of industry, but has also been subject to many studies in academia. Imagine two macroscopically separated objects that are brought into contact after their rupture, will eventually heal to one object again. Many research groups around the globe are working on this topic to fully understand the underlying mechanism. Van der Zwaag et al. have evaluated the self-healing capabilities of an epoxy-terminated polysulfide polymer cured in a polyaddition reaction using a mercaptan-functionalized curative or crosslinker. Their remarkable result is shown in Figure 3.
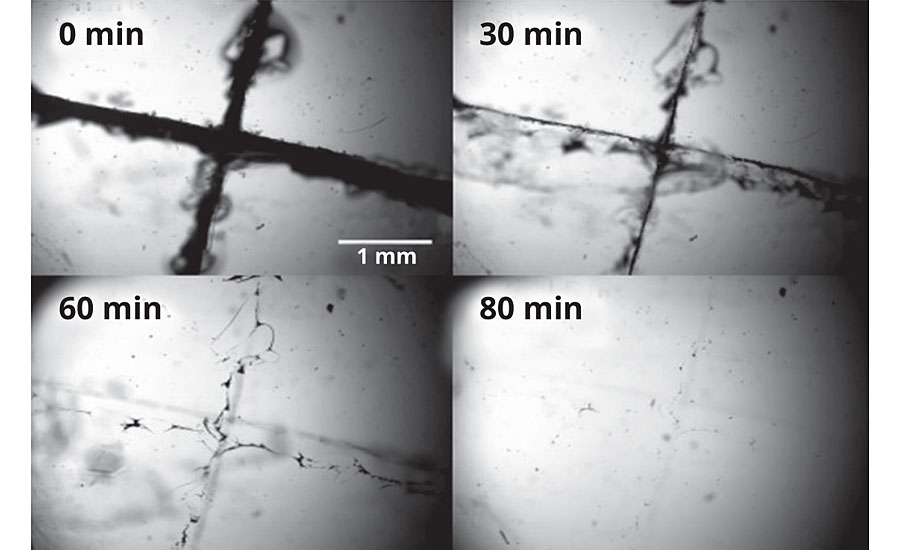
The figure shows optical microscope images that reveal the self-healing capabilities of polysulfide polymers. In this case, the material has been cut and heated up to 100 °C. After 80 min, no damage was observable anymore. By variation of the crosslinker amount, the researchers could even reduce the duration of the self-healing process to less than 20 min. The self/healing capabilities of polysulfides have even been shown at temperatures as low as 60 °C.
Naturally, microscopy images are not a proof for 100% restoration of the sample’s properties before the physical damage has been caused to it. Thus, Quan et al. have prepared dog-bone-shaped tensile test samples from mercaptan-terminated polysulfides cured with a standard bisphenol F/A epoxy resin to see if the initial tensile strength and elongation values can be reached after cutting the specimen into two pieces (Figure 4).
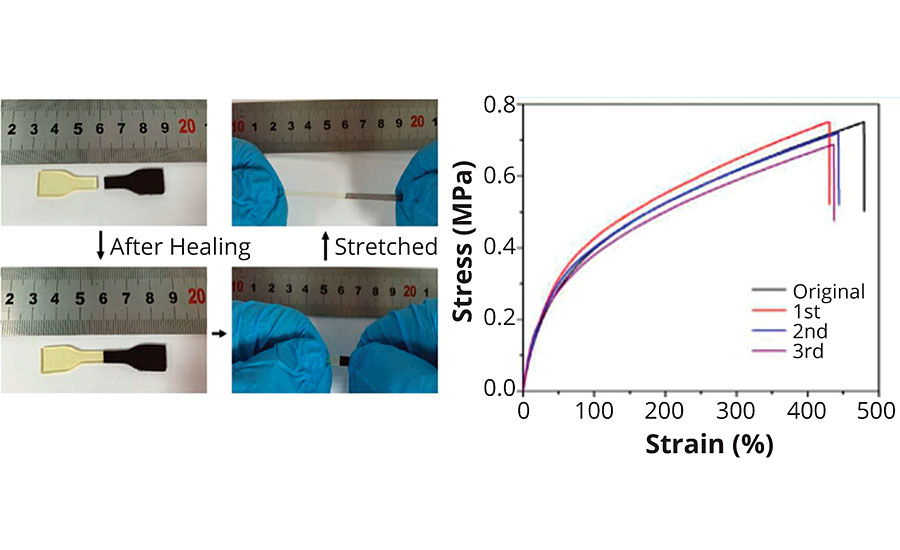
The Chinese research group has shown that polysulfide polymers are even able to self-heal after severe damage. In this case, the specimens have macroscopically been separated and brought into contact again at 75 °C for about an hour. After this time, the samples showed almost the same tensile strength and elongation at break as the original, unharmed sample.
“Real-Life” Application Examples
An outstanding example of a practical use of the self-healing feature are Thioplast-based coatings of aircraft tanks (Figure 5). In this demanding application, not only a superior resistivity against jet fuel and a broad permanent service temperature range (-55 °C-120 °C) are required, but a quick and reliable repair of the material is of utmost importance, too. During the 30 years of an aircraft’s lifetime, cracks may form in the fuel tank sealant. In that case, maintenance workers simply cut out the broken piece of sealant and spread a new layer of fresh polysulfide-based sealant on it. Due to the self-healing capabilities of polysulfide polymers, the newly applied material builds covalent bonds to the old but intact parts of the sealant and – after some waiting – no interface between the two materials remains.
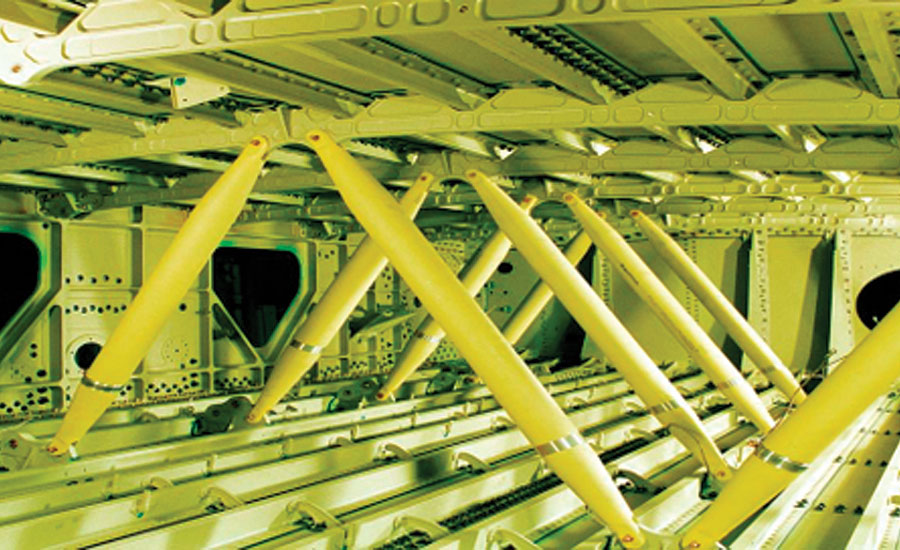
Self-healing via sulfur exchange reactions is not only needed in the aerospace industry. Construction joints and sealants that undergo single large movements, e.g. upon settling of a construction sealant joint, must be capable of stress-relaxation. If the sealant joint is not capable of stress-relaxation movements, the joint will be under constant physical stress and will eventually fail. The unique self-healing and self-rearrangement performance of Thioplast polysulfide polymer-based joints allows such movements.
Conclusion
It is up to the creative minds of the industry to make use of polysulfide polymer self-healing properties to create uncontested market environments for polysulfide-based sealants, adhesives and coatings, and priceless added value to their customers.
References
1. Steudel, R. (1975). Eigenschaften von Schwefel-Schwefel-Bindungen. Angewandte Chemie (19), S. 683-720.
2. U. Lafont, H. v. (2012). Influence of Cross-linkers on the Cohesive and Adhesive Self-Healing Ability of Polysulfide-Based Thermosets. ACS Applied Materials and Interfaces, S. 6280-6288.
3. Wentong Gao, M. B. (2017). Self-Healable and Reprocessable Polysulfide Sealants Prepared from Liquid Polysulfide Oligomer and Epoxy Resin. ACS Applied Materials and Interfaces, S. 15798-15808.
4. Wool, R. (02 2008). Self-healing materials: A review. Soft Matter, S. 400-418.
Looking for a reprint of this article?
From high-res PDFs to custom plaques, order your copy today!