DSC Technology: An Enhanced Tool for Coatings Analysis

TA Instruments has introduced the Q Series(tm) line of DSCs incorporating Tzero(tm) technology. This development involves more than just an improved DSC cell with better baseline stability. It uses a new measurement principle and an additional element on the DSC sensor that together provide compensation for known intrinsic instrument distortion of the DSC signal.1 With the virtual elimination of the latter, it is now possible to measure specific heat capacity directly in a single scan, and to achieve greater sensitivity and accuracy than ever before. Tzero technology also reduces analysis time, especially for Modulated Differential Scanning Calorimetry(r) (MDSC) measurements.
Glass Transition and Viscoelastic Properties
The mechanical properties of coatings can be largely understood in terms of the viscoelastic behavior of polymers. Due to long chain entanglements, polymers in the amorphous phase exhibit a range of properties, from viscous flow at higher temperatures, to rigid solid at lower temperatures. Material properties change most rapidly through the glass-transition (Tg) region, over which viscosity, strength, flexibility, permeability and abrasion resistance typically change by at least an order of magnitude. Thus an effective coating is one that has a Tg positioned such that an adequate mix of physical properties is obtained over the service temperature of the final product.In designing a coating product, the choice of Tg range comes down to the choice of polymer system, pigments, the solvent system (e.g., solvent- or waterborne) and an additive package. including fillers, plasticizers, curing agents and biocides. DSC is used to assess the Tg of both the formulation components and the final, applied product.

DSC of Polyurethane Cure
Figure 1 shows a DSC analysis of a 10 mg polyurethane floor finish. The first curve, "initial heat," is that of a partially set sample as it is heated from low temperature at a constant heating rate. As the sample passes through the Tg region the molecules gain sufficient mobility to affect the crosslinking reaction, which then appears as an exothermic peak. The Tg in this mostly cured material is above room temperature, indicating that the polymer matrix has hardened to the touch. As the sample is further heated the cure advances, with the exothermic peak indicating further crosslinking. In the second curve, labeled "reheat," the original sample is now reheated under the same conditions. It now shows that the Tg has shifted to a higher temperature (35.46BC), indicating that the material has achieved greater mechanical strength. Since the exothermic energy is a direct measure of the bonds formed, measuring the peak area (energy units) can be used to assess additional bond formation. Measuring the residual curing energy and comparing it to a theoretical or standard value provides a measure of the degree of cure. The Tg can also be used to estimate degree of cure if a correlation has previously been established. A deterrent to cure completion is that as the system crosslinks, the Tg increases, since the molecules lose mobility and reduced diffusion limits the cure rate.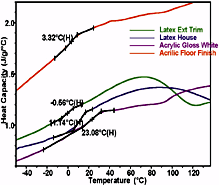
Waterborne Systems
Because solventborne finishes contain VOCs that can contribute to pollution and carry potential health risks for users, there has been a major effort to replace them with aqueous formulations. While many of the application characteristics of these newer "latex" and "acrylic" products have been improved over the older alkyd systems, mechanical properties often have not, posing a challenge for formulators of waterborne systems. For many such formulations, the strength properties are achieved without the strongly exothermic crosslinking reaction that typifies drying of the solventborne systems. The formulations provide a mix of viscoelastic components that display broad or multiple transitions. A DSC analysis of four common waterborne samples (see Figure 2) shows significant formulation differences. The changes in heat capacity (DCp) at Tg yield a measure of the amount of material in each amorphous phase, while the area of an endothermic peak reveals the amount of material in any crystalline phase. However, thermal curves from these complex formulations can be difficult to interpret, and obtaining more accurate Tg measurements requires improvements in DSC technology.
Improved Technology Baselines
When transitions extend over tens of degrees it is especially important to have a straight instrumental baseline. If the underlying instrument baseline is not straight, then the same curvature that appears in the instrument baseline is superimposed on the sample data. Then when the constructs are placed to determine Tg, the curvature will result in an incorrect placement of Tg and compromised determination of DCp at Tg. This problem is especially troublesome when high sensitivity is required to observe a subtle transition. In DSC analysis of coatings, the sample size is also limited when the material is analyzed on a substrate, or when a film of typical end-use thickness is laid down from solution in a DSC sample pan. In many cases quantitative results depend upon the sensitivity of the DSC apparatus.Tzero technology permits improvements in baseline straightness by an order of magnitude over previous DSC designs. Figure 1 shows a typical instrument baseline for the data presented. Notice that the baseline is not only flat but also is at zero milliwatts. This is because Tzero technology effectively removes the causes of instrumental baseline offset and curvature.
Figure 3 shows the DSC traces of three can lining samples, where each specimen was 1-2 milligrams. The baseline is essentially straight and noise free over a wide temperature range. Straight baseline performance is especially challenging for a DSC when the sample is a thin film evolving moisture, as is the case here. With the new Tzero technology heat flux cell design, the data are far less susceptible to the adverse effects of sample movement and moisture release than would be the case with DSCs employing the power compensation-type measuring principle.

Specific Heat Capacity
The fundamental thermodynamic property one needs to analyze is the specific heat capacity. The normal output of a DSC is heat flow, which includes the specific heat of the sample (times the sample weight and scan rate) plus the baseline characteristics of the analyzer. Once the signature of the instrument has been removed by using the full four-term heat flow equation made accessible by Advanced Tzero Technology, the displacement from the zero line (whether heat flow or specific heat units) is directly the heat flow to the sample specimen. As a result, any DSC analysis can be a specific heat analysis - it is merely a choice of units. On other DSC designs, the heat flow signal includes offset, slope and curvature from the instrument, so it is necessary to perform DSC scans with, and without a sample, and a third scan using a standard material, in order to determine specific heat. Figure 2 shows Cp data on waterborne finishes on an expanded scale. The lower Cp of the pigment as compared to that of the polymer is responsible for the Cp gap between the pigmented and clear finishes.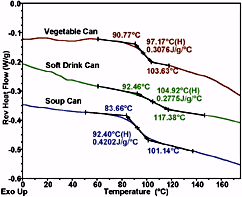
MDSC Aids Interpretation
The DSC analysis of multiple phase polymeric systems is complicated by the various phase and crystallite equilibria, which shift with temperature. The result is often a complex thermal curve that may include superimposed glass transitions, evaporation and curing peaks. Perhaps the greatest DSC advance in the recent decade has been that of Modulated DSC,2 in which a small temperature modulation is applied to the underlying linear temperature program. By applying Fourier Transform analysis to the resultant heat flow signal, it is possible to separate out rapidly reversing processes, such as specific heat and Tg, from kinetically controlled processes, such as evaporation, crystallization and crosslinking reactions. Figure 4 shows an MDSC analysis of a polyurethane floor finish, where the heat capacity related, reversing heat flow signal containing the Tg event, is totally separated from the kinetic related nonreversing signal, which contains curing and evaporation phenomena. In this way, volatiles lost and further curing in the Tg region does not adversely effect the Tg determination; and the change in Cp, which attends the curing, does not adversely effect the calculation of the heat of curing, or of kinetic analysis of the curing process.MDSC can also aid interpretation of the can liner data presented in Figure 3. From the heat flow data of conventional DSC, it would be difficult to unambiguously assign a value for Tg. However, in Figure 5, a typical Tg transition in the reversing heat flow curve is accurately obtained by the ability of MDSC technology to remove the competing irreversible effects of volatiles release and residual curing.
MDSC is also a valuable tool in the interpretation of complex amorphous systems. A previous need to use a slow underlying heating rate (e.g., 2-3?C/min), which resulted in reduced sensitivity and long experimental times, has now been resolved by Advanced Tzero Technology. The MDSC data shown in Figure 4 was taken using a period of 20 seconds and an underlying heating rate of 10degC/min, a standard for DSC polymer analysis. The MDSC analysis took no longer than DSC analysis in Figure 2.
Conclusion
DSC has long been used to develop successful coatings products. However, the Q Series DSCs with Tzero technology provide significantly more accuracy and precision in the measurements made. By providing straighter baselines, improved sensitivity, better resolution, direct Cp data, and faster MDSC measurements, the new Q1000 with Advanced Tzero technology is an enhanced tool in the design of coatings.For more information on differential scanning calorimetry, contact R. Bruce Cassel, TA Instruments, 109 Lukens Drive, New Castle, DE 19720; or Circle Number 65.
References
1 Danley, R.L. and Caulfield, P.A. "DSC Baseline Improvements Obtained by a Heat Flow Measurement Technique", Proc. 29th Conf. N. Amer. Therm. Anal. Soc. (2001).2 Thomas, L.C. "High Heating Rate MDSC, Using Tzero(tm) Technology", Proc. 29th Conf. N. Amer. Therm. Anal. Soc. (2001).
Looking for a reprint of this article?
From high-res PDFs to custom plaques, order your copy today!