Optimizing Dispersant Levels for Waterborne Pigment Color Concentrates

The optimum dispersant concentration (ODC) is typically quantified as dispersant weight per unit pigment weight. The higher the surface area per unit volume of a pigment, the higher the ODC. The ODC also depends on dispersant type: Is the dispersant a simple surfactant? A small molecule? A low-molecular-weight copolymer? A resin? Additionally, technical literature indicates variations in ODC between different dispersants that are classed in the same family.
Recent publications have labeled copolymeric dispersants as “high molecular weight” (HMW) and other low-molecular-weight dispersants as “traditional.”2-4 The exact molecular weights of commercial HMW dispersants are proprietary, but the literature has focused on the molecular weight differences to distinguish HMW dispersants from traditional dispersants.
Color pigments may be dispersed directly into a coating or dispersed as a color concentrate that is stored and later used to tint a coating. This article focuses on the dispersion of color pigment to make color pigment concentrates, hereafter referred to as color concentrates.
Pigment Dispersion
Pigment dispersion is the process of breaking apart a pigment agglomerate (a collection of adsorbed pigment particles) into individual pigment particles, wetting all pigment surfaces, and sustaining separation between individual pigment particles.Pigment agglomerates are broken apart using techniques such as ball milling, small media milling and high-speed dispersing. The separation of pigment particles and the desorption of gas and liquid residues from the pigment surface exposes new pigment surface. Pigment wetting is achieved when dispersants and solvents adhere to exposed pigment surfaces. Dispersants sustain pigment particle separation by maintaining a minimum safe distance between two pigment particles: ~10 nm if the dispersant is a resin, and ~5 nm if the dispersant is a surfactant.5,6 If the distance between pigment particles is less than the minimum safe distance, attractive forces will bring particles together, forming flocculates. Flocculation leads to noticeable changes to pigment properties such as color strength, gloss and viscosity.
Dispersants maintain pigment separation by two mechanisms: electrostatic stabilization and steric hindrance. For electrostatic stabilization, a double layer of ionic charges surrounds each pigment particle. For example,1 a pigment preferentially adsorbs anions [–], and is subsequently surrounded by a second layer of cations [+]. When two pigment particles approach each other, coulombic repulsive forces dominate, changing the trajectory of each particle to prevent close contact. The electrostatic stabilization mechanism requires a polar solvent, such as water. Water ionizes to H3O+, and OH–, and thus can displace conjugate ions, allowing cation [+] / anion [–] dissociation.
For steric hindrance, the bulk of a dispersant chain is the barrier to close contact between pigment particles. When chains intermingle, osmotic pressure builds up and drives solvent between the chains. The steric hindrance mechanism works in both polar (e.g., water) and nonpolar (e.g., mineral spirits) solvents.
Degree of Dispersion
Following are descriptions of several tests1 that are used to determine the degree of pigment dispersion.- Hegmann grind measures the size of the larger pigment particles. A well-dispersed pigment gives a higher Hegmann grind reading. Two coatings with the same Hegmann grind reading may give different degrees of opacity and/or color development.
- Colorimetry measures the color strength quantitatively as vectors in color space. For this article, color strength values are obtained by adding color concentrates to a white tint base and measuring reflectance values from the dried films in CIELAB L* (Commission Internationale d’Eclairage 1976 L*a*b*). Colorimetry has been called the most effective evaluation method for extent of dispersion.7
- Particle size analysis provides information about the pigment particle size, including both the range of particle diameters and relative pigment population as a function of particle diameter.
- Brookfield viscosity quantifies the difference between a color concentrate with a poorly dispersed pigment and a color concentrate with a well-dispersed pigment.
The following method uses quantitative colorimetry measurements to judge the degree of dispersion. Literature was researched for theories and/or test methods regarding optimum pigment dispersion, and one detailed procedure was found, the Daniel Flow Point Method.1
The Shaker Test
The proposed laboratory method for preparing color concentrates, referred to as the shaker test, uses a platform type paint shaker (Harbil high-speed paint mixer — model #3300000) to screen dispersants for efficacy and to optimize dispersant levels. The shaker test can accommodate four samples simultaneously. This guarantees that samples in a comparison study have received identical milling times. The shaker test reduces the time required for a dispersant study using a laboratory small media mill by 80%.Method
Glass beads (1.25 mm diameter), pigment, liquid (5 parts water:1 part propylene glycol) and dispersant are placed in a 4 oz. glass jar, which is secured between two parallel plates and shaken for a total of 12 minutes in three-minute intervals. The color concentrate mass ratios are calculated as follows.1. Set the total mass for the color concentrate (excluding glass beads), i.e., 70 g.
2. Set the pigment concentration based on total mass. For example, 21% carbon black=14.70 g carbon black [70*(21/100)=14.70].
3. Set the dispersant concentration based on carbon black mass. For example, 18% dispersant=2.65 g dispersant [14.70*(18/100)=2.65 g].
4. Add defoamer (0.10 g used for all samples).
5. For the remaining mass, the 5 parts by weight water:1 part by weight propylene glycol mixture is added [70 – (14.70 + 2.65 + 0.10) = 52.55 g water/propylene glycol].
6. Add a specified mass of glass beads [e.g., 84 g of glass beads].
The color concentrate mass ratios may change with a change of the pigment being tested.
The lid of the glass jar is secured with electrical tape. Up to four glass jars are placed in a wooden holder (see photo, page 34) to prevent glass jars from shaking free from the parallel plates. The wooden holder contains four cylindrical insets into which the bottoms of the glass jars will fit. The jars are shaken for a total time of 12 minutes in three-minute intervals.
At the conclusion of the shaker test, 1.25 oz glass jars are filled with 18.00 g of a commercial white tint base (100%-acrylic, interior pastel base). The color concentrate is added, 0.75 g, and the jars are sealed. The glass jars are placed between the parallel plates, and shaken for three minutes. A drawdown, using a 3 mil Bird Film Applicator, is made on a Leneta™ Penopac chart. A rubout is performed on the unsealed portion of the chart, with 50 circular rubs ~30 seconds after the time of the draw down. The drawdowns are air dried at room temperature, and colorimetry data is measured. Color measurements are made with a Microflash® 200d (Data Color International).
Laboratory Small Media Mill
For the laboratory small media mill control test, a pearl mill impeller driven by a Dispermat® (model AE02-M) at 3,000 rpm is used. A series of 1.25 oz glass jars are filled with 18.00 g of white tint base, prior to the milling. A premix of pigment, liquid (5 parts water:1 part propylene glycol) and dispersant is placed in a 500 ml water-jacketed stainless steel container. Glass beads with a 1.25 mm diameter are added and the top of the container is closed with a metal lid. A time interval between data points is chosen. At the selected time interval the mill is stopped, and a color concentrate aliquot of 0.75 g is removed and added to the white tint base in a glass jar. The rotor speed is set to 3,000 rpm for the time interval, and the process is repeated. When all sample jars have been filled, the jar lids are secured with electrical tape, and the color concentrate/white tint base mixtures are shaken for three minutes. Three mill drawdowns are made. A rubout is performed on the unsealed portion of the chart, with 50 circular rubs ~30 seconds after the time of the drawdown. The drawdowns are air dried at room temperature, and colorimetry values are measured.Experimental - Verification of the Shaker Test
A shaker test was described as a means to reduce the time required to screen dispersants for efficacy and optimizing the dispersant concentration for a specific pigment. To determine the effectiveness of the method, the following experiments were carried out.
Experiment 1: Does the method provide pigment dispersion?
An improvement in color strength with time indicates that pigment dispersion is taking place. A mixture of 14.7 g carbon black, 51.23 g liquid (5 parts water:1 part propylene glycol), 3.97 g dispersant, 0.10 g Troykyd® PA 230 defoamer and 83.88 g glass beads was weighed into a 4 oz. glass jar. This yields a slurry with 27% dispersant on pigment and 21% pigment on total mass. The jar is sealed and shaken for three-minute intervals taking 0.75 g aliquots at each interval until eight aliquots were collected. Samples were prepared from these aliquots for colorimetry measurement by mixing with white tint base, as previously established in the shaker test. The reflectance value, reported as CIELAB L*, is measured and the rubout is observed. L* decreases with increasing color development of the carbon black pigment. Data is shown in Figure 2.
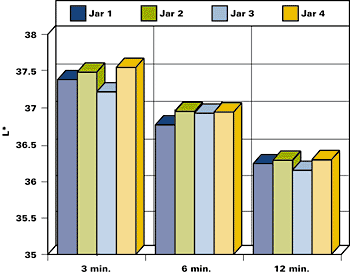
Experiment 2: Is the data reproducible?
The same color concentrate formulation presented in Experiment 1 was repeated. Four identical samples were prepared and shaken simultaneously. Aliquots were taken at three, six and 12 minutes. Samples were prepared from these aliquots for colorimetry measurement by mixing with white tint base, as previously established in the shaker test method. The reflectance values, reported as CIELAB L*, are measured to test the reproducibility of experimental results and the rubout is observed. Data is shown in Figure 3.
Experiment 3: Is the data comparable to established laboratory dispersion methods?
Following the laboratory small media mill method, a dispersion was made using the same color concentrate formula used in Experiments 1–2. The laboratory small media mill requires a quantity of color concentrate three times larger than the shaker test. It was decided that seven aliquots would be taken at five-minute intervals. Samples were prepared from these aliquots by mixing with white tint base, as previously established in the shaker test. For the colorimetry test, the reflectance values, reported as CIELAB L*, are measured and the rubout is observed. Data is shown in Figure 4.Use of the Shaker Test
The proposed shaker test provides pigment dispersion, reproducibility and dispersion results comparable to an established laboratory small media mill. The usefulness of the shaker test will be demonstrated in the following three experiments. The following materials were selected for the study.- Traditional dispersants — TRAD-1, TRAD-2 and TRAD-3 at 100% active; and TRAD-4 at 70% active
- High-molecular-weight dispersants — HMW-1 at 52% active, a block copolymer dispersant
- Pigment — Raven 1200 beads, a general-purpose carbon black supplied by Columbian Chemicals.Figure 5 / Dispersant Efficacy
Experiment 4: Evaluating dispersant efficacy
Four experimental traditional dispersants, TRAD-1, TRAD-2, TRAD-3 and TRAD-4, were evaluated using the shaker test to determine efficacy in the dispersion of a carbon black pigment. Sixteen different color concentrates were used: four dispersants, each at four dispersant concentrations. Using the color concentrate formulation used in Experiment 1, the dispersant concentrations were varied. The dispersant concentrations were 12%, 15%, 18% and 21% on pigment weight. Four samples at each dispersant concentration were shaken simultaneously for 12 minutes in three-minute intervals with aliquots taken at 12 minutes. Samples were prepared from these aliquots by mixing with white tint base as previously established in the shaker test. For the colorimetry test, the reflectance values, reported as CIELAB L*, are measured and the rubout is observed. The process was repeated for each dispersant concentration. From the graph of the colorimetry data, efficacy can be identified by decreasing L* values (TRAD-2 and TRAD-4), as shown in Figure 5.Figure 6 / Optimizing Dispersant Concentration (Carbon Black)Experiment 5: Optimizing dispersant concentration for a carbon black pigment
Based on results of Experiment 4, TRAD-4 was selected for optimization of carbon black pigment. Four color concentrates were prepared. Using the color concentrate formulation used in Experiment 1, the dispersant concentrations were varied. The dispersant concentration values were 18%, 21%, 24% and 27% on pigment weight. Samples were shaken simultaneously for 12 minutes in three-minute intervals with aliquots taken at 12 minutes. Samples were prepared from these aliquots by mixing with white tint base, as previously established in the shaker test method. For the colorimetry test, the reflectance values, reported as CIELAB L*, are measured and the rubout is observed. The data is shown in Figure 6.Figure 7 / Equal Weight SubstitutionExperiment 6: Importance of dispersant optimization
An experimental dispersant, Trad-4, optimized at 21-24% dispersant per weight of carbon black was compared to a commercial high molecular weight dispersant, HMW-1, requiring 65-80% dispersant (manufacturers recommendation) per weight of carbon black. Four color concentrates were prepared. Using the color concentrate formulation used in Experiment 1, the dispersant concentrations were varied. Two color concentrates used Trad-4 at a dispersant concentration of 21% and 80% on pigment weight. Two color concentrates used HMW-1 at a dispersant concentration of 21% and 80% on pigment weight. Samples were shaken simultaneously for 12 minutes in three- minute intervals with aliquots taken at 12 minutes. Samples were prepared from these aliquots by mixing with white tint base as previously established in the shaker test. For the colorimetry test, the reflectance values, reported as CIELAB L*, are measured and the rubout is observed. The data is shown in Figure 7.Figure 8. Below Optimum Concentration vs. Optimized ConcentrationConclusion
The shaker test method can be used to determine dispersant efficacy and optimum dispersant concentration for a carbon black pigment. The method was evaluated for effectiveness and reproducibility and compared to an established laboratory small media mill method. The experiments show that the proposed shaker test is effective for the determination of dispersant efficacy and the determination of the optimum dispersant concentration for a particular carbon black pigment. In our tests, dispersants with efficacy were identified by substantial decreases in L* values with increasing dispersant concentration.The proposed shaker test allows the comparison of four dispersants or dispersant levels at one time. This provides four samples, for comparison, with the exact same time and energy of dispersing action. Experiments show that the shaker test is reproducible from one sample to another and results matched those obtained using a traditional laboratory small media mill. The ability to test four samples at one time and the small amount of clean up required for the shaker test, results in a time saving of over 80% when compared to the time required using the traditional small media method.
The importance of optimizing dispersant concentration for a particular pigment is pointed out in Experiment 5. The comparison of an experimental traditional dispersant (Trad-4) to a high-molecular-weight dispersant (HMW-1) showed that the HMW-1 dispersant was not efficacious when used at the optimized level of the experimental traditional dispersant, 21% on carbon black pigment weight. Increasing the level of the HMW dispersant to 80%, the manufacturer’s recommended use level, gave results comparable to the traditional dispersant at 21%. This was shown by the L* values and comparing color acceptance using the rub up test.
This paper was presented at the Waterborne, High-Solids and Powder Coatings Symposium on March 1–3, 2000, in New Orleans.
For more information on pigment dispersions, contact Troy Corp., 8 Vreeland Road, Florham Park, NJ 07932.
Looking for a reprint of this article?
From high-res PDFs to custom plaques, order your copy today!